Fig. 11.1
(a–c) Dorsal and palmar photographs and radiograph of an “atypical” cleft hand, now classified as a central absence or oligodactylic type II symbrachydactyly, affecting the left hand of a 4-year-old boy. Published with kind permission of Neil F. Jones ©2014. All rights reserved
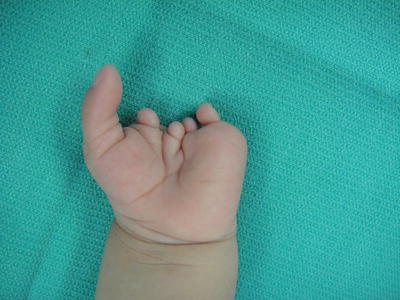
Fig. 11.2
Monodactylic type III symbrachydactyly of the left hand in a 2-year-old girl. The four fingers are represented by nubbins and this would be classified as a U4R1 hand. Published with kind permission of Neil F. Jones ©2014. All rights reserved
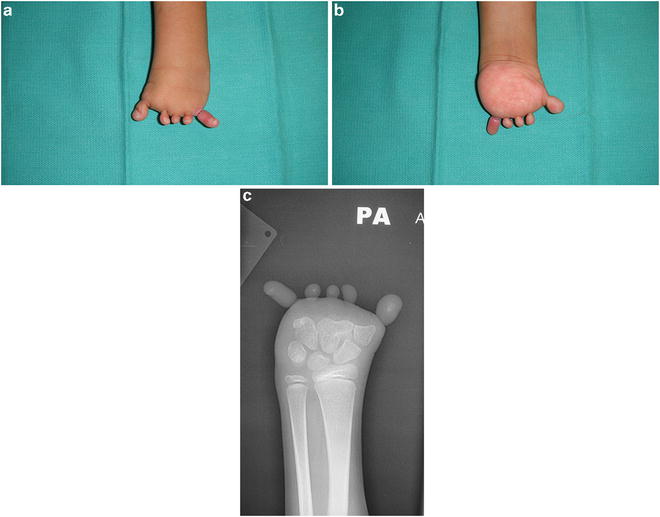
Fig. 11.3
(a–c) Dorsal and palmar photographs and radiograph of a 2-year-old girl with adactylic type IV symbrachydactyly of her left hand. All five digits are represented just by nubbins and are missing from the level of the carpometacarpal joints. This would be classified as an R5 hand. Published with kind permission of Neil F. Jones ©2014. All rights reserved
Suguira refined the Blauth and Gekeler classification in 1976 [9]. He further subclassified the type one or short-fingered hands based on the number of phalanges in each digit. The least involved digits were the triphalangeal type. Diphalangeal and monophalangeal types have two phalanges and one phalanx respectively.
Ogino et al. analyzed 76 children with symbrachydactyly [10]. All were unilateral; 48 were classified as type I, 9 were type II, 8 were type III, and 11 were type IV. Ogino argued that type I symbrachydactyly is a mild form of an intercalary transverse deficiency, whereas types II through IV represent terminal transverse deficiencies.
Yamauchi further divided the classification of symbrachydactyly into seven types [11]. In type 1 or triphalangia type, the hand has its full complement of bony structures although the middle phalanges are usually short. Type 2 or diphalangia type hands have one phalanx, usually the middle phalanx, missing. Type 3 or monophalangia type hands have a digit or digits containing only one phalanx. Type 4 or aphalangia type hands have a digit or digits that are missing all three phalanges. Type 5 or ametacarpia type hands have absence of the metacarpal and all three phalanges in one digit or several digits. Type 6 or acarpia hands have absence of all the digits and a partial or complete absence of the carpus. Type 7 or forearm amputation have absence of the distal part of the forearm.
All of the classification systems have inherent weaknesses. In particular, the IFSSH classification originally placed the two transverse abnormalities in two different categories, with transverse arrest being placed in category I failure of formation and symbrachydactyly under category V undergrowth. Swanson wrote in his original paper “failure of formation may be manifest as an almost transverse arrest of the entire hand with only rudimentary radial and ulnar digits present,” yet showed a case of symbrachydactyly as an example. The Japanese Society for Surgery of the Hand considers symbrachydactyly to be synonymous with transverse failure of formation and therefore believes that symbrachydactyly be moved to category I of the IFSSH classification. Symbrachydactyly is now being seen as a distal manifestation of transverse deficiency, whereas transverse arrest is seen as a more proximal manifestation of transverse deficiency. However, this understanding combines symbrachydactyly in which the initial deficiency is hypoplasia of the middle phalanges with preservation of the distal elements, with transverse deficiency in which the distal elements are missing completely.
Jones and Kaplan [12] suggested a new documentation system for congenital absent digits based on their review of photographs and PA radiographs of 235 hands in 204 children born with absent digits. This documentation system does not attempt to imply any underlying embryological causation, but unlike most other classifications, it provides a simple description of either the morphological or radiographic appearance of a child’s hand to facilitate communication between physicians. Three letters can describe each hand: R (radial), C (central), and U (ulnar) as well as five numbers. The first letter and number describe which rays are missing and the second and third letters and numbers describe the rays that are present. A normal hand is therefore described as R0. An absent thumb would be described as R1U4. The spectrum of radial deficiencies includes R2U3, R3U2, and R4U1 (Fig. 11.4). The spectrum of transverse deficiencies and ulnar deficiencies include U1R4, U2R3, U3R2, and U4R1 (Fig. 11.5). A hand with a thumb but absent fingers would be designated as U4R1. This is the most common phenotype and corresponds to the Blauth and Gekeler monodactylic type III form of symbrachydactyly (see Fig. 11.2). Typical cleft hand or central longitudinal deficiency as it is now known, would be designated as C1R2U2. Other central deficiencies (Fig. 11.6) include C1R1U3, C1R3U1, C2R1U2, C2R2U1, and C3R1U1 (the old “atypical” cleft hand, which corresponds to the Blauth and Gekeler oligodactylic type II form of symbrachydactyly). Complete absence of all five digits would be designated as R5, which corresponds to the Blauth and Gekeler peromelic type IV form of symbrachydactyly (see Fig. 11.3). The documentation system can be further refined by describing the level at which the rays are absent: w—radio carpal joint to carpometacarpal joint, m-distal to the carpometacarpal joint to just distal to the metacarpophalangeal joint, p—distal to the metacarpophalangeal joint out to the proximal third of the middle phalanx or the tip of the thumb, and d—distal to the proximal third of the middle phalanx to the tip of the finger.
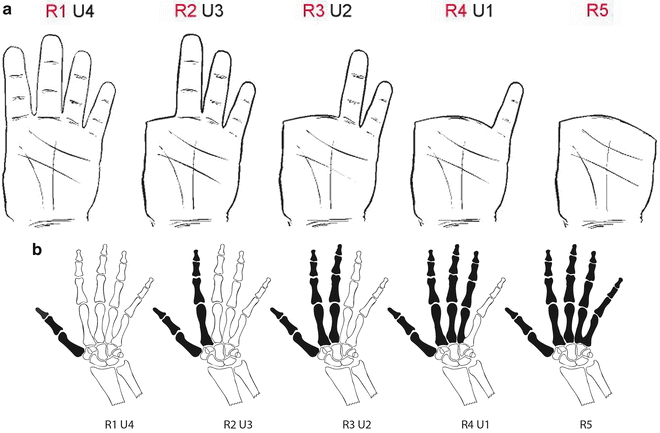
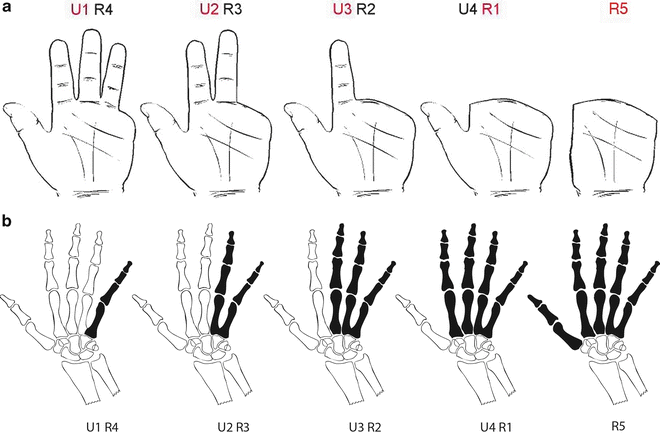
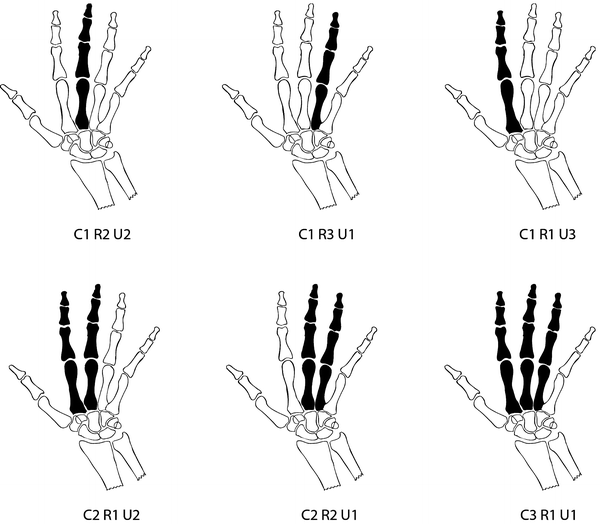
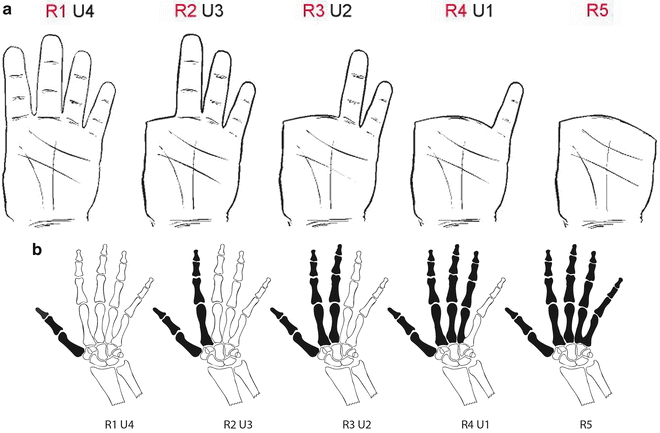
Fig. 11.4
(a, b) Schematic representation of congenital absent digits affecting the radial side of the hand. Published with kind permission of Neil F. Jones ©2014. All rights reserved
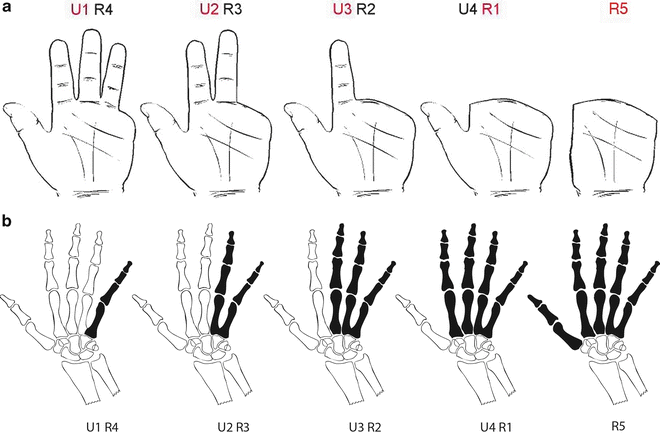
Fig. 11.5
(a, b) Schematic representation of congenital absent digits affecting the ulnar side of the hand. Published with kind permission of Neil F. Jones ©2014. All rights reserved
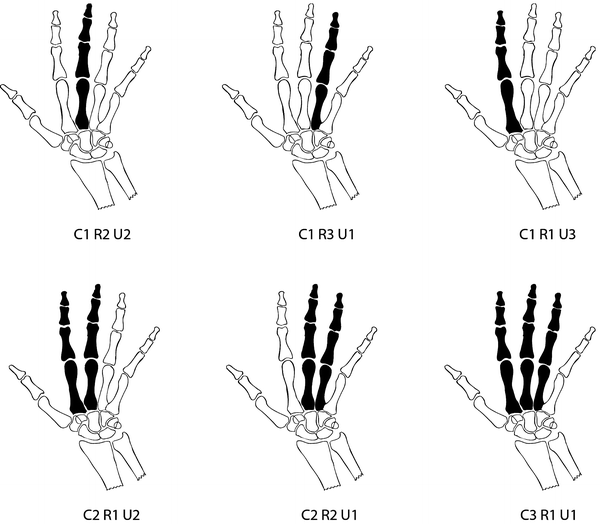
Fig. 11.6
Schematic representation of congenital absent digits affecting the central part of the hand. Published with kind permission of Neil F. Jones ©2014. All rights reserved
The Jones and Kaplan system incorporates all the previous subclassification systems that have attempted to describe congenital absent digits in transverse deficiencies, central deficiencies, and symbrachydactyly and simplifies the documentation of these children’s hands. Blauth and Gekeler [8] and Buck-Gramcko [13] postulated a “reduction theory” in symbrachydactyly which starts at the level of the middle phalanges producing hypoplasia of the middle phalanges (“brachymesophalangia”) and then proceeds proximally, so that the distal phalanges or parts of the distal phalanges are always present as digital nubbins with rudimentary nails. With progression, there is absence of the proximal and middle phalanges of the central three fingers, the index, middle, and ring fingers, resulting in the oligodactylic type II “atypical cleft hand” form of symbrachydactyly, which corresponds to the C3R1U1 phenotype (see Fig. 11.1). Reduction progresses to involve the small finger resulting in the type III monodactylic form of symbrachydactyly, which corresponds to the U4R1 phenotype (see Fig. 11.2) and finally extends to the thumb resulting in the type IV peromelic or adactylic form of symbrachydactyly with absence of all digits, corresponding to the R5 phenotype (see Fig. 11.3). Therefore, symbrachydactyly is represented by the C3R1U1, U4R1, and R5 phenotypes.
Another issue is the reclassification of cleft hand within symbrachydactyly. The description “typical cleft hand” has now been replaced with the term “central longitudinal deficiency.” But the old term “atypical” cleft hand (see Fig. 11.1) has now been reclassified as “symbrachydactyly central absence type” within category I transverse deficiency [14, 15]. However, reduction of rays proceeds ulnarly from the central three digits in the oligodactylic type II form of symbrachydactyly, leaving only a thumb and no fingers, resulting in the monodactylic type III form of symbrachydactyly and corresponding to a U4R1 phenotype (see Fig. 11.2); whereas in an “atypical” cleft hand, reduction proceeds radially leaving the ring and small fingers or only a single small finger on the ulnar side of the hand, resulting in a R3U2 or R4U1 phenotype (Fig. 11.7). In the authors’ opinion, these are two completely different phenotypes that are being placed together in the same category!
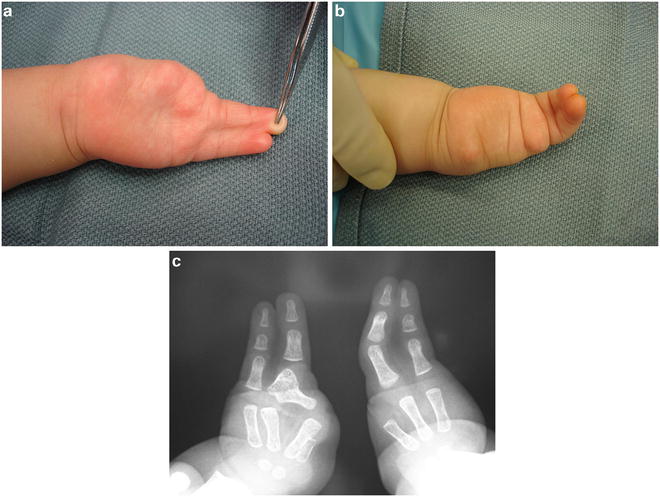
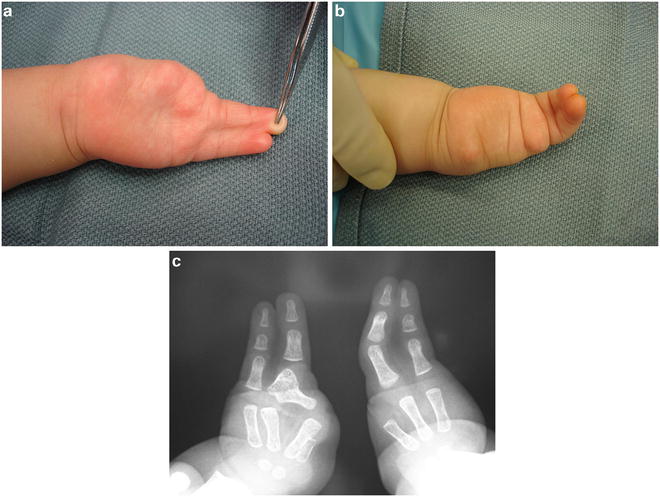
Fig. 11.7
(a–c) Two-year-old boy with bilateral cleft hands, missing the thumb, index, and middle fingers. The ring and small fingers are involved in a complete simple syndactyly. This would be classified as a R3U2 hand. Published with kind permission of Neil F. Jones ©2014. All rights reserved
Clinical Features
The hand affected by symbrachydactyly can present with a variety of findings. A common feature is short digits frequently involved in varying degrees of simple incomplete to complex complete syndactyly, or instead of fingers just “nubbins” [16]. Another feature associated with symbrachydactyly is skin invagination in the palm, thought to represent the attachments of forearm extrinsic muscle-tendon units [17]. The extensor tendons in symbrachydactyly are more normal and extend out over the hypoplastic metacarpals, but the flexor tendons often form a single amorphous tendon mass within the carpal tunnel [18].
Distinguishing symbrachydactyly from other conditions caused by other transverse failures of formation can be difficult. Kallemeier et al. [19] examined the relationship between transverse deficiency and symbrachydactyly in 271 children with a diagnosis of transverse deficiency at the level of the forearm; 207 of these children (93 %) had manifestations of symbrachydactyly—soft tissue “nubbins” or skin invagination at the distal aspect of their limbs. They concluded that symbrachydactyly and congenital transverse deficiency of the forearm represent two points on a single continuum, in that transverse deficiency at the level of the forearm represents a more proximal form of symbrachydactyly.
Miura and Suzuki [20] also highlighted these difficulties when they attempted to differentiate between typical cleft hand and the “atypical” cleft hand (central absence type) seen in symbrachydactyly. They examined the length of the metacarpals in normal hands, syndactyly, cleft hands, symbrachydactyly, and constriction band syndrome and found that hands with symbrachydactyly and failure of formation of parts had shortened metacarpals; whereas hands with syndactyly, constriction band syndrome, and typical cleft hand had normal length metacarpals.
Pediatricians and even some surgeons have difficulty differentiating transverse failure of formation, symbrachydactyly, and congenital constriction ring syndrome. A child’s hand affected by a transverse failure of formation usually has shortened digits with smooth “amputation” stumps, without “nubbins” or evidence of constriction rings (Fig. 11.8). Radiographs may show tapering of the phalanges or shortened metacarpals. A child’s hand affected by symbrachydactyly usually shows either a thumb and a small finger separated by “nubbins” or a wide cleft (see Fig. 11.1); or a thumb but missing all four fingers just represented by “nubbins” (see Fig. 11.2); or absence of all five digits represented just by “nubbins” (see Fig. 11.3). Radiographs will reveal shortened or absent metacarpals in the affected digits. Finally, a child’s hand affected by congenital constriction ring syndrome will show a relatively smooth amputation of one or several fingers with evidence of constriction rings affecting other digits or more proximally the wrist or forearm (Fig. 11.9a); or amputation of several fingers with adhesion of the amputation stumps together distally (acrosyndactyly) with sinuses representing the web spaces more proximally (see Fig. 11.9b). Radiographs typically show normal bony architecture proximal to the constriction rings.
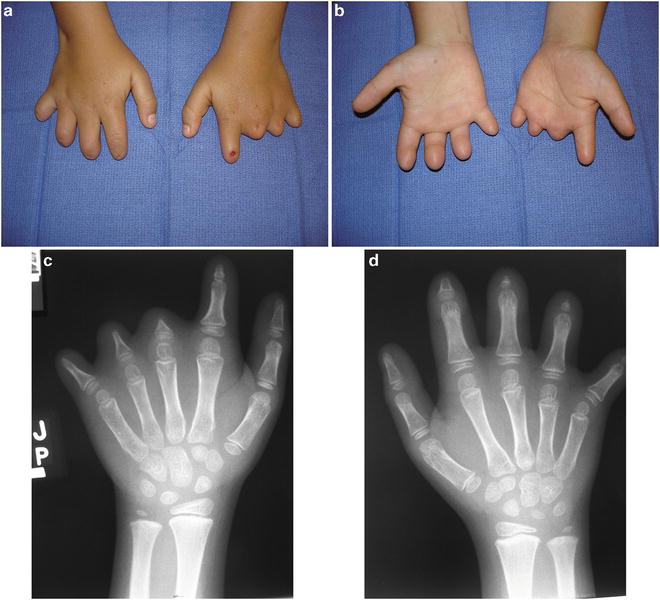
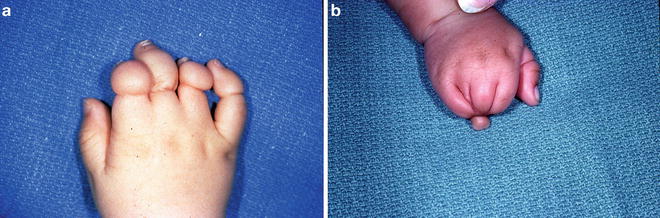
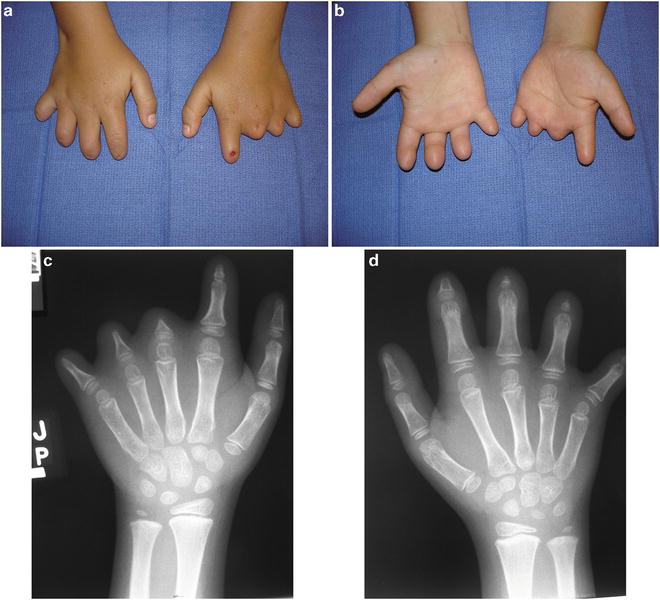
Fig. 11.8
Dorsal and palmar photographs (a, b) and radiographs (c, d) of a 6-year-old boy with a transverse failure of formation affecting both hands. In the right hand the failure of formation is at the level of the base of the middle phalanges. In the left hand the level of failure of formation is at the level of the proximal phalanges in the middle, ring and small fingers and at the base of the middle phalanx in the index finger. Published with kind permission of Neil F. Jones ©2014. All rights reserved
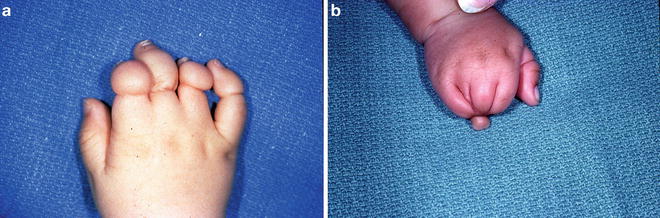
Fig. 11.9
Congenital constriction ring syndrome affecting the index, middle, ring and small fingers of the right hand (a). Acrosyndactyly of the right hand with amputation of the distal phalanges and coalescence of the terminal portions of the fingers together with sinuses represent the web spaces more proximally (b). Published with kind permission of Neil F. Jones ©2014. All rights reserved
Etiology
The exact cause of symbrachydactyly is not known. Mesenchymal stem cell defects in the hand plate are presumed to be the cause due to the hypoplastic nature of the hand in symbrachydactyly [21]. The likely mesodermal nature of the defect explains the persistence of ectodermal structure such as the finger pulp, nail fold, and nail even in severe presentations [22]. Bavnick and Weaver proposed that subclavian artery disruption at different points in embryological development could explain a variety of mesodermal anomalies seen in Poland’s syndrome, Mobius syndrome, and Klippel-Feil syndrome [23]. There is no known hereditary pattern of inheritance described for symbrachydactyly.
There is no known animal model for symbrachydactyly. There are however, mice with functional null mutations in growth and differentiation factor 5 (Gdf5) that display shortened limb bones with a phenotype very similar to symbrachydactyly in humans. They are referred to as brachypodism mice [24]. The metacarpals, metatarsals, and proximal phalanges are significantly shortened, and the middle phalanges are often absent. Kanauchi et al. [24] examined the bony histology of the hypoplastic bones in these brachypodism mice. The hypoplastic bones showed an endochondral ossification pattern but lacked a growth plate and epiphysis. The authors speculated that a similar mechanism explains the hypoplastic bones seen in symbrachydactyly.
Surgical Treatment
Surgical options for reconstruction of children with transverse failure or symbrachydactyly include nonvascularized toe phalangeal bone grafting, distraction osteogenesis, and microsurgical toe-to-hand transfers.
Nonvascularized Toe Phalangeal Bone Grafts
The first treatment option advocated for the treatment of short digits in symbrachydactyly was the nonvascularized transfer of toe phalanges.
The first report of nonvascularized toe phalangeal transfer was by the German surgeon Wolff in 1910 [25] and 1911 [26]. He reported the transfer of the second toe proximal phalanx to the proximal phalanx of a finger that had been destroyed by a tuberculosis infection. Entin [27] first used this technique in 1959 in the treatment of severe transverse deficiency. The technique was reintroduced by Carroll and Green in 1975 [28]. They reported on 159 toe phalanges transferred in 79 patients. They found that no open physes continued to grow, but did not see evidence of resorption. Complications of this technique in their series included skin necrosis at the tip of the lengthened digit in four patients and a pin tract infection in one patient.
Goldberg and Watson [29] examined their experience with 20 patients and 36 digits treated with nonvascularized toe phalangeal transfer. In contrast to the findings of Carroll and Green [28], 90 % of the growth plates in children under the age of 18 months remained open at an average follow-up of almost 4 years. This rate dropped to 67 % in patients 18 months to 5 years old and to 50 % in patients over the age of 5 years. They reported growth rates 90 % of the contralateral non-transferred phalanges when growth plates remained open.
Buck-Gramcko [30] reported on his experience with 40 patients with symbrachydactyly and constriction band syndrome who underwent transfer of 63 nonvascularized phalangeal bone grafts. He reported 100 % “take” of the bone graft provided that the periosteum over the phalanx was not disrupted and the graft was not split. He found that the best timing for transfer of the phalanges was between 19 and 48 months. Attempts to recreate a functional joint led to variable results with a range of motion ranging from 0 to 90°. Complications in his series included skin necrosis in five patients and joint subluxation requiring reduction in two patients.
Radocha et al. [31] described their experience in the transfer of 73 phalanges with a minimum follow-up of 1 year. They found a 94 % rate of open physes in patients operated on before 1 year of age. The rate dropped to 71 % for those operated on between 1 and 2 years of age and dropped further to 48 % for those older than 2 years of age. Growth rates per age group were found to be 1 mm/year in the two younger age groups and 0.5 mm/year in the group over the age of two. The authors stressed the importance of extraperiosteal dissection during harvesting of the phalanx; ligament, and tendon repair in the recipient digit and a young age (under 12 months) as important factors in maintaining an open physis and therefore the potential for further growth.
Cavallo et al. [32] reported on the transfer of 64 phalanges in 22 children with aphalangia from symbrachydactyly and constriction band syndrome. They found a total digital elongation of 6 mm at an average of 5 years of follow-up. The average range of motion at the newly created joint was found to be 60°. The most common complication in their series was graft instability or malposition, seen in 17 % of the cases, more commonly in cases of atypical cleft hand.
Gohla et al. [33] reported on the transfer of 113 nonvascularized toe phalanges in 48 patients with diagnoses of symbrachydactyly and constriction band syndrome. The operative technique used was similar, and the patients were grouped as previously described by Buck-Gramcko [30]. Epiphyseal plate survival was highest in those patients treated before 18 months of age with an 87 % rate of open physes at follow-up examination. The rate of open physes dropped only to 86 % in patients aged 19 months to 4 years and to 64 % in patients over the age of 4 years. They also looked at rates of bone resorption and found a 45 % rate of resorption in patients over the age of 4 years compared to a rate of only 4 % in patients under 18 months old. Eighteen of the 48 patients developed a complication, including four cases with necrosis of the skin resulting in the loss of the phalangeal bone grafts. Six children had scarring significant enough to require secondary procedures such as Z-plasties or local flaps. Six other transfers were complicated by digital instability or graft displacement.
Donor Site Morbidity and Patient/Parental Satisfaction
The transfer of nonvascularized bone into a soft tissue envelope has been complicated by bone resorption, lack of bone growth, and donor site morbidity. Unglaub in 2006 [34] looked at outcomes of toe phalangeal transfers including growth, resorption, donor site morbidity, patient satisfaction, and parental satisfaction. He divided patients into similar groups as did Buck-Gramcko [30]: under 1.5 years old, 1.5–4 years old, and older than 4 years. Patients under 1.5 years showed good growth of the transferred phalanges with very few cases of resorption. Patients in the middle age group showed no growth in the transferred bone. Patients over 4 years of age had a 54 % rate of graft resorption. He found little morbidity attributable to the donor site. He felt that the functional gains of the procedure were mostly from increased length as little active motion was achieved in the transferred joints in his series. Seventy-five percent of the parents in this series were highly satisfied with the functional gains and “manual skillfulness” provided by the nonvascularized toe phalangeal bone graft procedure.
The issue of donor site morbidity was also addressed by Bourke and Kay [35] who noted that all the toes with phalanges harvested by the technique described by Buck-Gramcko [30] were shortened, floppy, and had a tendency to cross over other toes. They introduced a technique of placing a nonvascularized iliac crest bone graft in the donor toe with epiphysis present. This tubular bone graft was placed between the epiphysis at the base of the resected phalanx and a small cap of bone left from the harvested phalanx and pinned in place with a longitudinal Kirschner wire (K wire). They reported that their series of 11 patients had better preservation of toe length and stability.
Garagnani et al. [36] studied donor site morbidity clinically and radiographically in a series of 40 patients with hypoplastic digits. A total of 136 phalanges were harvested using supraperiosteal dissection as previously described, with repair of the extensor tendon after removal of the phalanx. The mean follow-up for the series was 122 months with a minimum follow-up of 36 months. The Oxford Ankle-Foot Questionnaire (OAFQ) is a validated questionnaire for children aged 5–16 years old that assesses subjective patient and parental satisfaction. Over 80 % of patients and families reported some degree of emotional problems related to their feet. Footwear related problems were noted by over 60 % of both patients and families. All of the patients interviewed reported a tendency to hide their feet. From a clinical perspective, shortening of the harvested toes was universal, and malrotation was seen in 76–100 % of the toes. Not surprisingly, clinical deformity increased when multiple phalanges were harvested from a single foot. Radiographic examination revealed hypoplasia of surrounding bony structures including the distal phalanx, middle phalanx, and metatarsal. One patient in their series even underwent amputation of bilateral overriding and unstable fourth toes with significant postoperative improvement in the appearance of the feet.
Indications and Patient Selection
Jones [37] described three specific indications for the transfer of nonvascularized toe phalanges. The first is stabilization of a floppy hypoplastic digit consisting of only a soft tissue envelope. The second is lengthening and stabilization of a digit that contains a remnant of the proximal phalanx. The third indication is stabilization of an intercalated defect between the distal phalanx and the metacarpal of a thumb. Based on the outcomes described earlier, the ideal patient for nonvascularized toe phalangeal transfers is a child under the age of 18 months with multiple short digits and a bony skeleton out to at least the level of the distal metacarpals with a sufficient soft tissue envelope [38].
Surgical Technique
Under tourniquet control, the hypoplastic digit is explored through a dorsal longitudinal incision. If a significant palmar soft tissue contracture is present, a volar approach could be chosen. Blunt dissection within the soft tissue is used to create a cavity for the donor bone. It is crucial to maintain a sufficient pad of soft tissue at the distal aspect of the digit to prevent necrosis caused by pressure from the donor bone. Typically, the flexor and extensor tendons are confluent over the hypoplastic metacarpal head. They are sharply divided to create independent flexor and extensor tendons and radial and ulnar collateral ligaments.
Typically the proximal phalanx from the third or fourth toe is used as a donor phalanx. The second toe can be used if a microsurgical second toe transfer is not planned for the future. A gently curved incision is used over the dorsum of the toe because a straight incision over the dorsum of the toe can result in an extension contracture of the toe. The extensor tendon is split longitudinally to expose the proximal phalanx. Previous experience [29–31] has shown that an extraperiosteal dissection of the proximal phalanx in a child under the age of 18 months provides the best chance for preventing resorption of the transferred bone. The collateral ligaments of the PIP joint are divided off the proximal phalanx while the collateral ligaments and volar plate of the metatarsophalangeal joint are harvested with the proximal phalanx.
The tourniquet on the leg is then released and hemostasis achieved. A variety of methods have been described to prevent shortening of the donor toe [30, 31, 35]. The simplest of these is suturing the extensor tendon to the flexor tendon. Iliac crest bone graft with its associated apophysis as described by Bourke and Kay [35] can be inserted to help maintain the length and stability of the toe. A 0.035-in K wire is then introduced retrograde through the toe into the metatarsal head and left in place for 4–5 weeks to hold the toe out to length.
The toe phalanx is transferred to the hand and the bone graft can be positioned in one of three basic constructs. In digits with a partial proximal phalanx, the graft can be placed distally in the “on top” position. In digits with an intercalary defect between a hypoplastic distal phalanx and metacarpal, the graft can be interposed between the two bones. Finally, the graft can potentially be used to simultaneously reconstruct the metacarpophalangeal joint and provide length to the floppy digit in children lacking all skeletal elements distal to the metacarpal head.
A 0.035-in or 0.028-in K wire is inserted through the phalanx. Nonabsorbable sutures are placed but not tied between the flexor tendon and the volar plate of the transferred phalanx as well as between the radial and ulnar capsule of the MCP joint and the radial and ulnar collateral ligaments of the transferred phalanx. The K wire is then advanced distally out through the distal soft tissues of the digit. The phalangeal bone graft is reduced into the soft tissue envelope of the digit and held in appropriate position relative to the metacarpal. The previously placed sutures are tied. The K wire is then advanced retrograde into the metacarpal. The extensor tendon and dorsal capsule of the MCP joint are repaired to the dorsal capsule of the donor phalanx. The hand is immobilized in a plaster splint and the K wire is left in place for 4–6 weeks postoperatively.
Because of only modest growth of nonvascularized toe phalangeal bone grafts; the problems of subluxation, instability, and resorption of the bone graft; and problems of the donor toe; we now rarely use this technique. Currently, we only use nonvascularized toe phalangeal transfer for elongating and stabilizing soft tissue finger stumps with bone out to the level of the metacarpal heads or just distal to the PIP joints and for intercalated bone grafting in a thumb missing the proximal phalanx (Figs. 11.10 and 11.11).
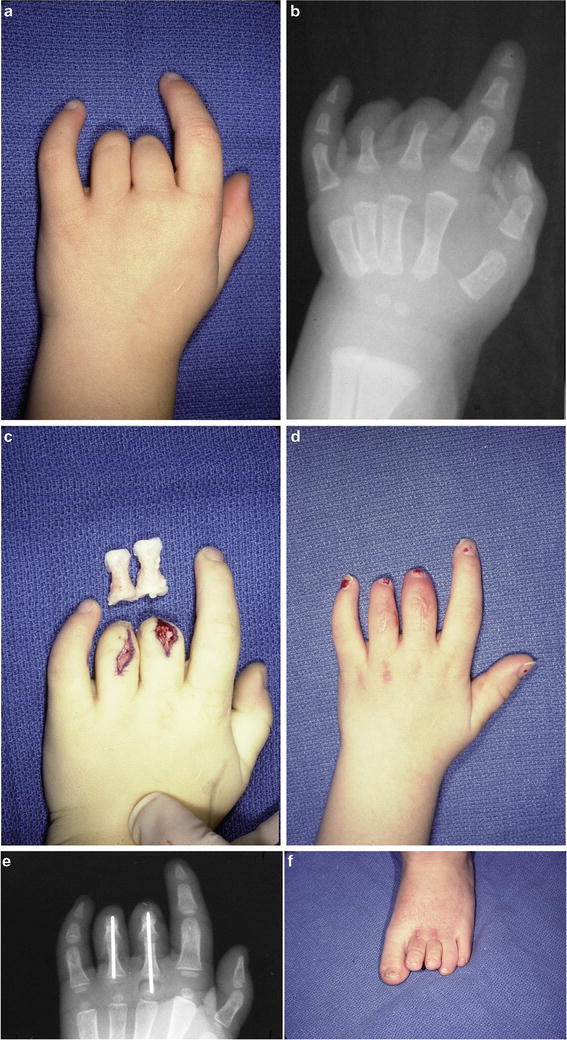
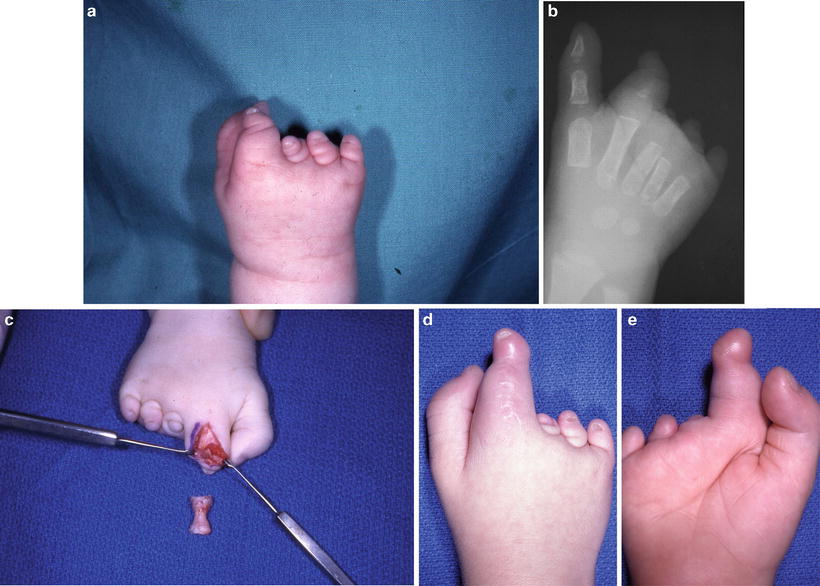
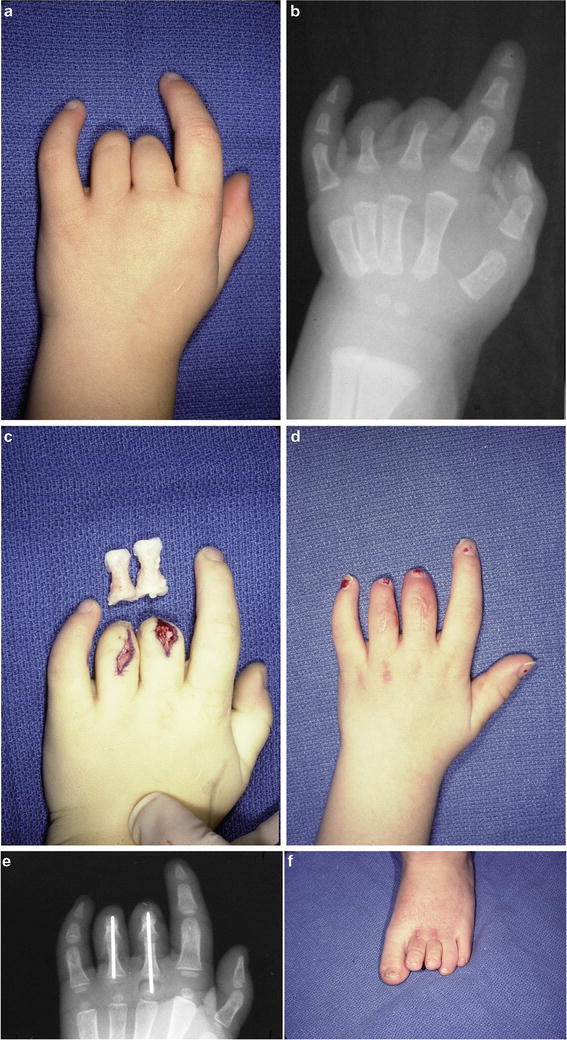
Fig. 11.10
Dorsal and palmar photographs and radiograph of a 4-year-old girl with a transverse failure of formation of her left middle and ring fingers at the level of the proximal phalanges (a, b). The soft tissue envelopes of these two fingers were stabilized and elongated using nonvascularized bone grafts from the proximal phalanges of the left second and third toes (c). Resultant lengthening of the left middle and ring fingers with the nonvascularized toe phalangeal bone grafts (d, e). The donor site in the left foot (f). Published with kind permission of Neil F. Jones ©2014. All rights reserved
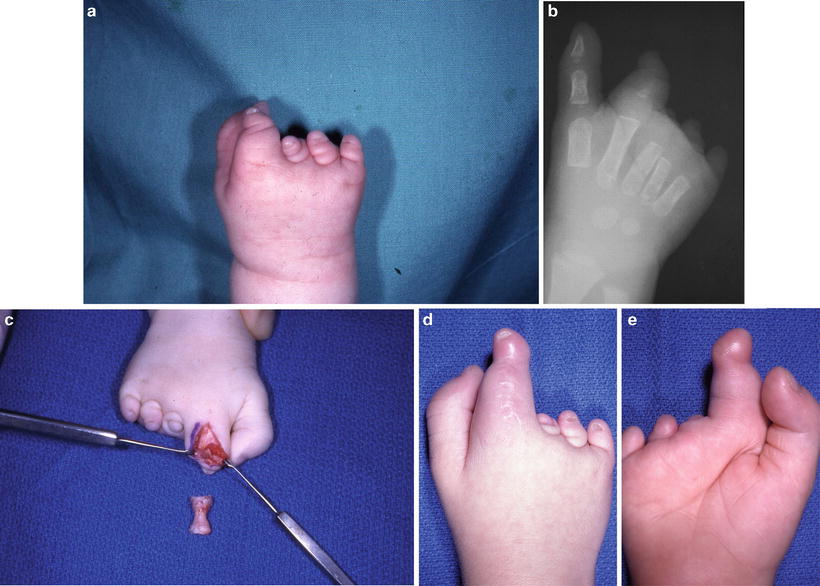
Fig. 11.11
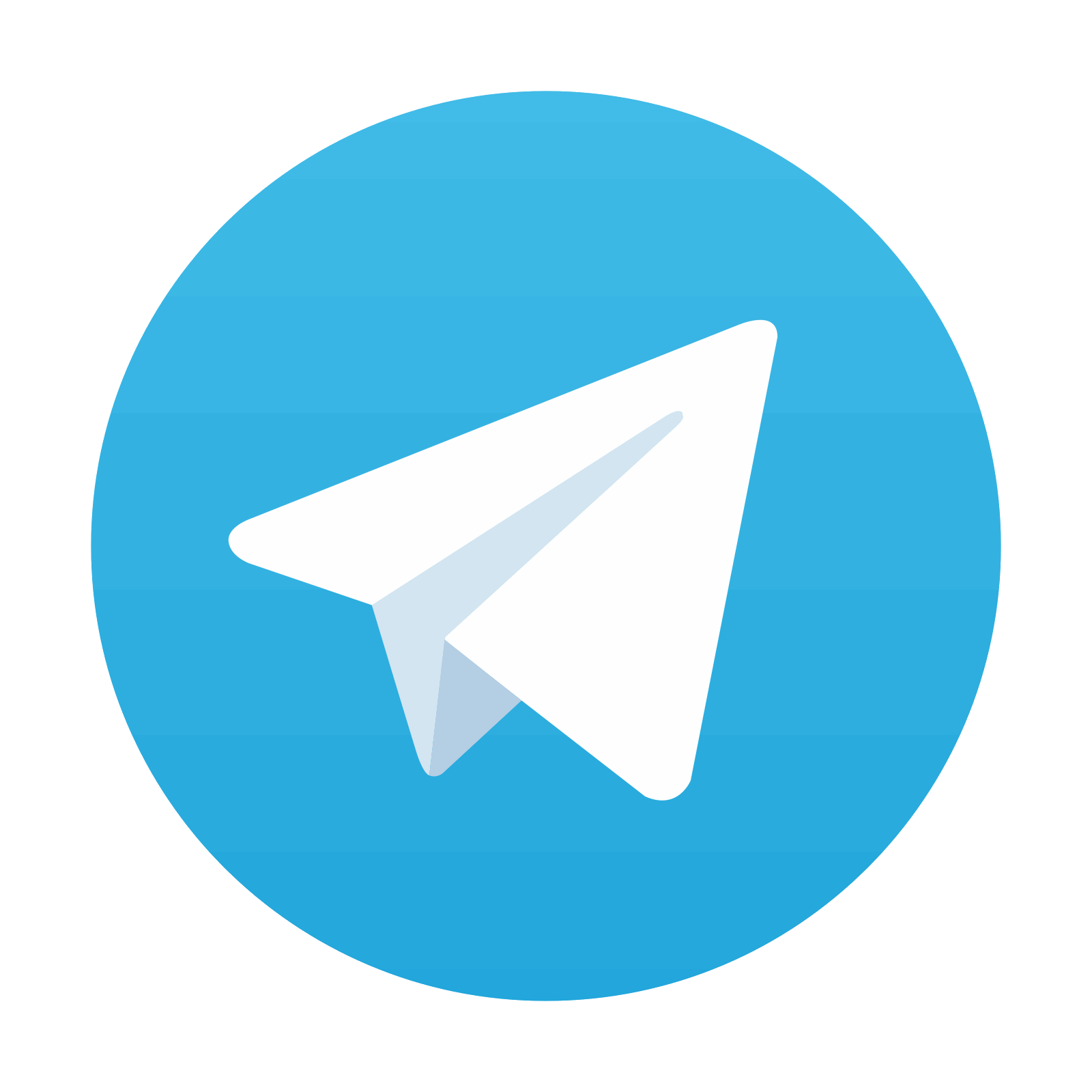
One-year-old boy with monodactylic type III symbrachydactyly affecting his right hand, classified as a U4R1 hand (a, b). His parents initially refused a microsurgical toe-to-hand transfer. Therefore he underwent a nonvascularized toe phalangeal bone graft from the right third toe to elongate and stabilize the right index finger (c). The postoperative result after nonvascularized toe phalangeal bone grafting of the right index finger (d, e). Published with kind permission of Neil F. Jones ©2014. All rights reserved
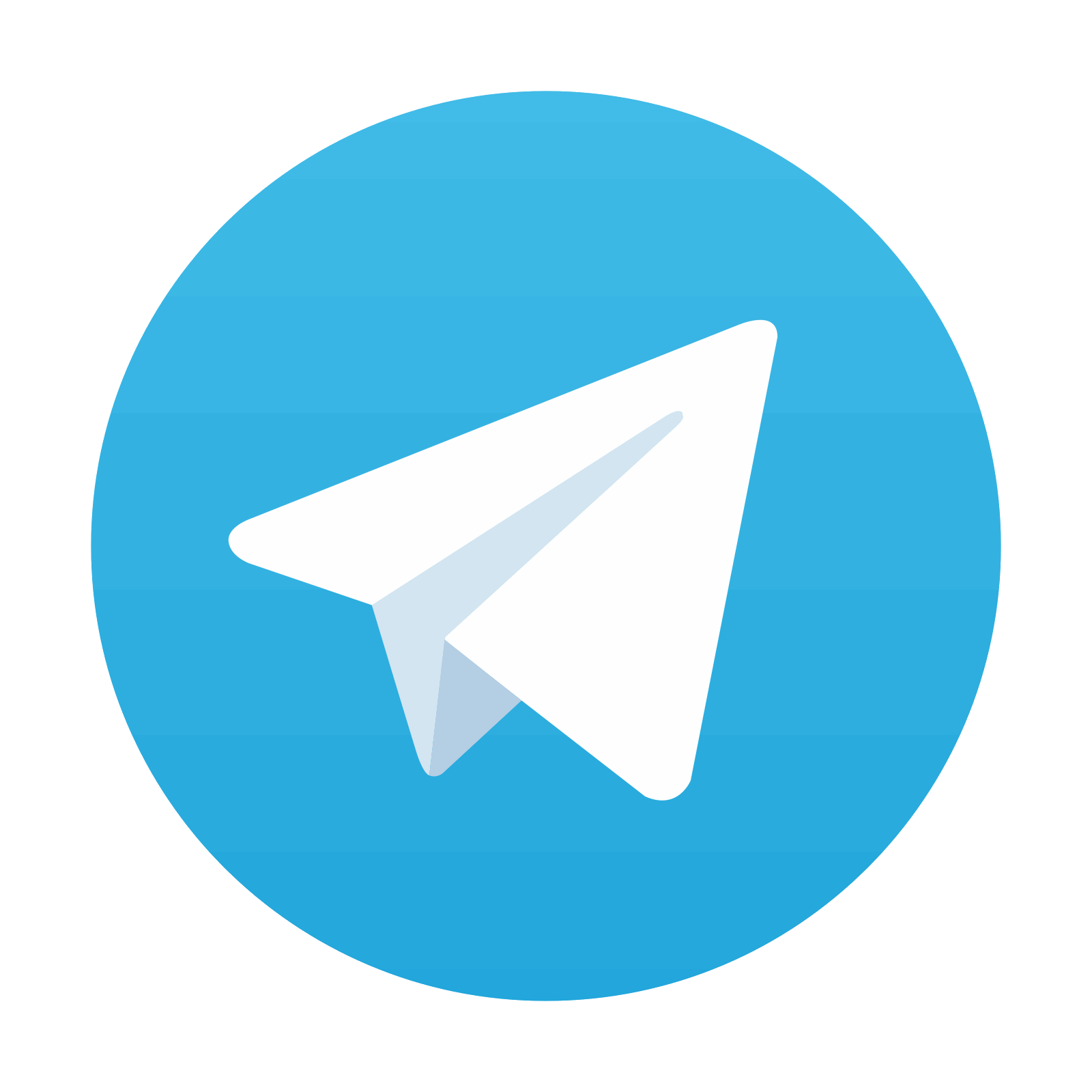
Stay updated, free articles. Join our Telegram channel

Full access? Get Clinical Tree
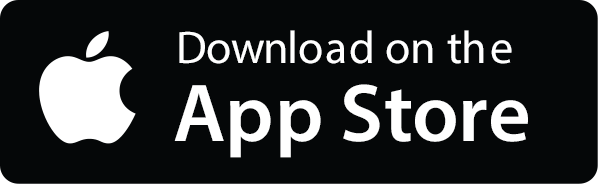
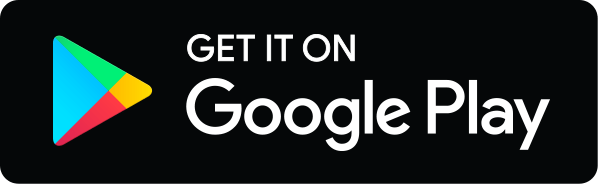
