Patients and clinicians use skin color attributes such as color uniformity, color distribution, and texture to infer physiologic health status. Normalization of skin color, surface texture, and height are important treatment goals in the treatment of scars. Skin color, structure, and response to trauma, vary with ethnicity. The incidence of hypertrophic and keloid scar formation is influenced by these inherent skin attributes. Skin type influences the response to various modalities including laser therapy and surgical intervention, and skin differences must be considered in treatment planning to achieve optimal results.
Key points
- •
Patients and clinicians use skin color attributes such as color uniformity, color distribution, and texture to infer physiologic health status.
- •
Normalization of skin color, surface texture, and height are important treatment goals.
- •
Skin color, structure, function response to trauma, and scar formation vary with ethnicity.
- •
The incidence of hypertrophic and keloid scar formation is influenced by these inherent variations.
- •
Skin type influences the response to various modalities including laser therapy and surgical intervention, and skin differences must be considered in treatment planning to achieve optimal results.
Introduction
Deep tissue wounds, burns, and surgical incisions can result in the formation of scars, that is, erythematous, firm, pruritic, raised fibrous masses that remain within the boundaries of the original wound, and may regress over time. Scars frequently have significant morbidity and psychological, cosmetic, and functional outcomes as well as overall quality of life. Patients seek treatment to improve the color, texture, surface roughness, pliability, range of motion functionality, and pain.
This paper discusses the impact of skin color and ethnicity on the physiologic characteristics of scars and on the evaluation of treatment efficacy. The implications of ethnic diversity in the selection of treatment strategies are examined.
Introduction
Deep tissue wounds, burns, and surgical incisions can result in the formation of scars, that is, erythematous, firm, pruritic, raised fibrous masses that remain within the boundaries of the original wound, and may regress over time. Scars frequently have significant morbidity and psychological, cosmetic, and functional outcomes as well as overall quality of life. Patients seek treatment to improve the color, texture, surface roughness, pliability, range of motion functionality, and pain.
This paper discusses the impact of skin color and ethnicity on the physiologic characteristics of scars and on the evaluation of treatment efficacy. The implications of ethnic diversity in the selection of treatment strategies are examined.
Skin color
Clinical judgments of scar progression and treatment effectiveness depend on perception of the skin surface. Humans use skin color attributes such as color uniformity, color distribution, and texture to infer physiologic health status. In one study, subjects were asked to adjust the red color of high-resolution facial images to achieve a “healthy appearance”. All of them increased the red color, regardless of the inherent pigmentation, but dark skin subjects increased the red color of dark skin photos more than lighter skin photos. Visual responses to facial images standardized for shape and surface features were measured. Images with more uniform skin coloration were perceived to be younger than those with greater color variability. These factors can reduce the perceived age by as many as 20 years. The perception of age may vary however depending on the viewer’s ethnicity. High-resolution images of facial skin (cheek region) of Japanese women aged 13 to 80 years were perceived to be older by Japanese observers if the skin was darker (L value) and more yellow (b*).
Perceived skin color occurs when visible light interacts with skin components such as constitutive pigments melanin (yellow to brown), oxygenated hemoglobin (red), deoxyhemoglobin (blue-purple), bilirubin (yellow), and carotene (yellow). Observed color arises from the interplay of light with components of the stratum corneum (SC), epidermis, and dermis and by diffuse reflection, scattering, and absorption of light inside the skin. About 5% of incident light is reflected back to the eye, whereas the remainder is absorbed, scattered, or transmitted within the SC, epidermis, dermis, and subcutaneous tissue. The SC transmits light, the epidermis and dermis absorb some light due to melanin and hemoglobin, and the subcutaneous fat scatters light. The Vancouver Scar Scale (VSS), commonly used to evaluate scars, infers vascularity from color descriptors, pigmentation from color due to melanin, height relative to the surrounding tissue, and pliability by palpation.
Skin structure, function, color, and ethnic diversity
Skin color is commonly described by 2 systems:
- 1.
Fitzpatrick skin type
- 2.
Von Luschan skin coloration
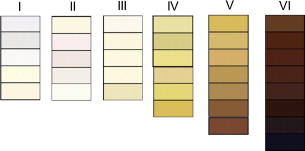
Fitzpatrick Type | Description | Effect of Light Exposure | Von Luschan’s Schema |
---|---|---|---|
I | Pale, fair, freckles | Always burns, never tans | Very light |
II | Fair | Usually burns, sometimes tans | Light |
III | Light brown | May burn, usually tans | Intermediate |
IV | Olive brown | Rarely burns, always tans | Mediterranean |
V | Brown | Moderate constitutional pigmentation | Dark or brown |
VI | Black | Marked constitutional pigmentation | Very dark or black |
Melanin, water, hemoglobin, and other chromophores absorb the incident light to varying extents, depending on the wavelength. Melanin synthesis occurs in the basal layer of the epidermis and is transferred to the keratinocytes throughout the epidermis. Melanin levels are higher in dark versus light skin and the latter has greater amounts of light melanin including pheomelanin and dihydroxyindole-2-carboxylic acid–enriched eumelanin. Additionally, African skin is characterized by significantly larger melanosomes than Indian, Mexican, Chinese, and European skin and size is greater in Indian than in European skin. Oxygenated blood in the dermal capillaries and vascular plexus and deoxygenated blood (blue-purple) in the dermal venules contribute to skin color. Bilirubin (yellow) in the epidermis results from precipitation in phospholipid membranes and leakage as a complex with albumin into extravascular regions. Skin coloration for a given individual varies with season due to differences in the amount of light exposure.
Melanin (M) content can be approximated from measurements of red reflectance using the equation M = log 10 (1/% red reflectance), and erythema (E) can be determined from the equation E = log 10 (1/% green reflectance) − log 10 (1/% red reflectance). Narrow band reflectance spectroscopy and CIE L*a*b* tristimulus color have been used to measure erythema, melanin, L*, and a* in various ethnic skin types ( Fig. 2 ). For subjects with lesser pigmentation, E and M are relatively independent and can be used to estimate erythema and melanin content. However, because E and M are correlated, they cannot be used to assess redness or melanin content for dark-skinned subjects.
Most of the published reports on ethnic skin comparisons have focused on the SC and epidermis rather than on exploring dermal and subcutaneous features. The vascular response to a topical corticosteroid (clobetasol) using laser Doppler technique was decreased in African Americans versus Caucasians and paralleled the reduction in erythema in earlier studies by the same investigators. The mechanism responsible for this effect was not discussed. Evaluations of three-dimensional reconstructed dermal equivalents and human tissue samples found the dermal-epidermal junction from African skin to have more epidermal projections, greater convolution, and to be more uniform than in Caucasian skin. African tissues had lower amounts of laminin 5, nidogen proteins, type IV collagen, and type VII collagen than Caucasians. The syntheses of keratinocyte growth factor and monocyte chemotactic protein-I were higher in African papillary fibroblasts.
Scars and skin type
Tissue injuries can result in significant inflammation including increases in cells eg, neutrophils, macrophages, monocytes, growth factors, and cytokines that stimulate fibroblast migration and proliferation, excessive collagen and extracellular matrix deposition culminating in the formation of a hypertrophic scar. These scars have fine, well-organized type III collagen bundles, containing fibroblasts, numerous myofibroblasts, endothelial cells, and a higher density of microvesssels. Examples of hypertrophic scars are provided in Fig. 3 .
The incidence of hypertrophic scars from burn injury ranges from 32% to 78%, and the risk factors are dark skin, female gender, age (younger), time to heal, injury severity, neck or upper limb site, and a higher number of surgeries. For pediatric patients undergoing reconstructive and release surgeries, scarring at the donor site can be more severe than in the original injury. An evaluation of the effect of ethnicity on hypertrophic scar from cleft lip repair found the highest incidence in Asian patients (36.3%) followed by Hispanics (32.2%) and Caucasians (11.8%). There were no African American patients in the study population.
Keloid scars are uniquely different from hypertrophic scars in that they grow beyond the boundaries of the original wound area. Keloids can develop in all races; however, individuals with darker pigmentation (Fitzpatrick IV, V, and VI) are more susceptible. In African American, Hispanic, and Asian populations, keloids can occur in up to 6% to 16% of individuals. Predilection for keloid development is found in patients who have cutaneous disorders resulting in a prolonged inflammatory response from a foreign body reaction or cellulitis. Histologically, keloid scars have an acellular collagen core with hypoproliferative fibroblasts, bundles of hyalinized collagen, and nodular shape. Immune cells have also been identified in keloid tissue. There is a strong genetic susceptibility for keloid scar formation but specific genes have not yet been identified. Increased levels of the cytokine transforming growth factor β have been implicated in keloid formation but multiple mediators are most likely involved. An example of a keloid scar is shown in Fig. 4 A .
Clinically, it is difficult to predict if a scar will develop into either a keloid or hypertrophic scar. When closing a wound, increased skin tension increases the risk of keloid formation. Sites on the body prone to keloids are the earlobes, mandibular angle, upper back, shoulders, posterior neck, upper arms, and anterior chest. In the differential diagnosis of keloids, scars that are ulcerative, bleeding, or fixed to its underlying tissue should be sent for pathologic analysis. Scar-appearing lesions may actually be dermatofibroma, scar sarcoidosis, keloidal scleroderma, morpheic or pigmented basal cell carcinoma, metastatic skin nodules, and dermatofibrosarcoma protuberans.
Scar management
Optimization of coloration is an important goal of scar treatment. Multiple modalities have been used for scar management, including glucocorticoid injections, retinoic acid, application of silicone gels, pressure garments, and simple massage. Application of intense pulsed light (IPL), photothermolysis with pulsed dye laser (PDL), and ablation with a fractional CO 2 laser are used to normalize scar color, size, and pliability. In randomized within-subject controlled study, one-half of newly healed skin grafts received PDL treatments at 6-week intervals in patients from a pediatric burn facility. The entire scar was treated with compression per the standard of care. Decreases in quantitative scar erythema and height and an increase in tissue elasticity were observed after 2 or 3 treatments for the PDL plus compression treatment versus compression alone. VSS scores showed improvement for vascularity, pliability, pigmentation, and height for PDL plus compression versus compression alone. Scars improved (VSS scores) following PDL treatment of linear operative scars 3 times beginning on the day of suture removal.
Clayton and colleagues reported on laser-based treatment of 95 patients with burn scars beginning 6 months post-injury. A combination of laser modalities was used to optimize outcome. In general, the initial treatment was with a 595-nm flashlamp-pumped PDL (7 mm spot, cryogenic cooling 30-msec spray, 20-msec delay, pulse duration 1.5 msec, overlapping 30%) beginning at fluencies of 5.0 to 8.0 J/cm 2 . After mitigation of hyperemia, the protocol used a fractional CO 2 laser (density 15%, frequency 600 Hz, 12.5–17.5 mJ pulse) to decrease thickness and reduce stiffness. Superficial ablation (frequency 150 Hz, 70–90 mJ per micropulse) was applied to improve texture. An IPL laser (filter 515–590 nm, fluence 18–24 J/cm 2 ) was used to normalize pigmentation and treat folliculitis. The incidence of hypopigmentation and hyperpigmentation was 12% and 2%, respectively. Adverse events were observed to a greater extent in patients with higher Fitzpatrick scores. Hypopigmentation and blister formation occurring more frequently compared with patients with lower Fitzpatrick scores.
Laser treatment of scars, particularly in darker-skinned patients, is confounded by the presence of melanin because it absorbs energy in region of 250 to 1200 nm ( Fig. 5 ). The pigment melanin absorbs some of the laser energy, up to 40% in very dark skin and, therefore, may diminish the desired effect on the scar. Compensation by increasing the power increases the heat to the tissue and can result in thermal damage, including blister formation and scarring. Laser treatment with cooling is frequently used to mitigate the increases in tissue temperature but post-inflammatory hyperpigmentation has been noted as a side effect of cooling. Melanin absorption can be reduced by using lasers with longer wavelengths where its absorption is lower. Longer wavelengths penetrate deeper into the dermis (the 810-nm diode laser and the 1064-nm Nd:Yag laser).
