36 Robotics, simulation, and telemedicine in plastic surgery
Synopsis
This chapter describes the latest innovations in nonbiological technologies – robotics, simulation, and telemedicine – and their integration into plastic surgery practice.
Robotics provides plastic surgeons with custom prostheses and robotic limbs, both of which help patients achieve cosmesis and function; it also provides surgical tools that reduce human error and increase surgical visibility, precision, and safety.
Simulation is used to train plastic surgery residents and fellows in all procedures, and to train senior surgeons on new procedures. Like robotics, simulation provides an increase in safety and reduction in medical errors, since trainees will effectively “make mistakes” on a simulator, rather than on live patients.
Telemedicine can make use of both robotics and simulation to deliver medical care to a distant population, whether during everyday life, in wartime, or following a disaster.
This chapter will provide plastic surgeons with a basic understanding of how to apply current technologies to enhance their armamentarium with solutions to clinical and surgical problems.
Introduction
Surgeons can enhance their practice with biological technologies, such as transplantation and tissue engineering, covered in Chapters 13, 19, 32, 34, 35, or with nonbiological technologies. This chapter discusses the latest innovations in nonbiological technologies – robotics, simulation, and telemedicine – and their widespread integration into plastic surgery practice. Plastic surgeons can implant custom prostheses that are fabricated by robots; outfit amputee patients with robotic limbs; and employ surgical robots that reduce human error and increase surgical visibility, precision, and safety. Simulation is used to train plastic surgery residents in common procedures, and to train senior surgeons on new procedures. Like robotics, simulation promotes safety, as trainees will make initial errors on a simulator before operating on live patients. Finally, telemedicine can utilize both robotics and simulation to deliver medical care to a distant population, whether during everyday life, in wartime, or following a disaster.
This chapter will provide plastic surgeons with a basic understanding of how to apply current technologies to enhance their armamentarium with solutions to clinical and surgical problems. Technological tools can resolve needs that are not met with current standards of care (for example, a robotic limb that restores function following an amputation with suboptimal limb salvage). However, it is critical that both outcomes and costs be considered whenever we evaluate new technology; the outcomes should be demonstrable, and the cost–benefit ratio considerable. (See section on robotic surgery: the evidence base for improved outcomes, below, and also Ch. 10.)
Robotics
Historical perspective
The term “robot” was coined in 1921, when a play by Karel Capek introduced machines called robots: forced laborers that simulated human beings.1 More recently, Merriam-Webster’s Collegiate Dictionary2 describes a robot as: (1) a machine that looks like a human being, and performs various complex acts (such as walking and talking) of a human being; (2) a mechanism guided by automatic controls.
Modern robots have proved to be useful tools in exploration and industry since the early 1960s. Robots and machines now do all sorts of tasks that used to require a human touch, such as entertaining children,3 vacuuming floors,4 exploring hazardous areas like the surface of Mars,5 driving a car,6 searching for victims at disaster sites such as the World Trade Center,7 or potentially doing dangerous tasks such as hazmat cleanup for disasters.8 A robot called “big dog” has four legs and can cover rough terrain better than humans.9 Androids, or human-looking robots, can now sing, act, provide human assistance and companionship, or replicate famous individuals, living or deceased.10–13
The use of robots in medicine and surgery is increasing. Mechanical hands were initially conceived in the 16th century, when Ambroise Paré, a military surgeon for the King of France, designed a mechanical hand with rods and gears (Fig. 36.1). Roughly 400 years later, in the 1960s, the first artificial computer-controlled robotic arm, the Rancho arm, was designed as a tool for the handicapped. The end of that decade saw the Stanford arm, the first electrically powered, computer-controlled robot arm. Robots are now used in some hospitals to deliver medicines, meals. and other materials.14 They help rehabilitate stroke patients and provide company to Alzheimer’s patients.15 Robotic surgical assists, like the da Vinci system, brought robotics into the arena of surgical technology, with benefits shared by surgeons and patients alike.
The role of robotics in plastic surgery: planning and performance
Robots are now used in plastic surgery in three applications, all of which involve planning and performance. First, industrial robots can fabricate medical products using computer-aided design and manufacturing (CAD/CAM),17 including custom prosthetics for contouring and filling cranial defects. In the future, robots may fabricate an entire prosthetic face. Second, high-end artificial arms and hands18–25 contain enough intelligence and autonomy to be considered robots themselves. Third, surgical robots are now used in endoscopic and image-guided surgery such as transoral partial glossectomy, and may be adapted for future use in minimally invasive plastics procedures.
The use of robotics in plastic surgery: surgical tools and prosthetics
Surgical robots in the operating room
Robot-assisted surgery is routinely practiced across specialties in the operating rooms of large hospitals and centers of excellence. Intuitive Surgical’s da Vinci® system, the first US Food and Drug Administration (FDA)-cleared robotic surgical system, is now cleared for use in cardiac, general, thoracic, urologic, gynecologic, pediatric and transoral otolaryngologic surgery (Fig. 36.2).26 The US FDA has not cleared the da Vinci system for use in plastic surgery. As this system is cleared for ear, nose, and throat (ENT) surgery, we believe it may have potential future application in plastics procedures. The da Vinci system’s tremor filtration feature, for example, would make it very useful in ultramicrosurgery, allowing plastic surgeons to sew together even smaller vessels than we can now achieve. Plastic surgeons have begun preliminary studies to investigate the use of robotic surgery for two techniques. Patel et al. tested robotic rectus abdominus muscle harvest, first on 2 cadavers and then on one 30-year-old woman.26a They found the technique possible and said that it may also “provide an approach to minimally invasive transperitoneal reconstruction.” Also, Selber tested robotic latissimus dorsi muscle harvest using 10 cadavers, and concluded that, “robotic harvest of the latissimus dorsi muscle is feasible and reproducible. It offers technical advantages over endoscopic harvest and cosmetic advantages over the open technique”.26b
Design features and usage of robotic surgery
In robotic surgery, the surgeon does not directly manipulate the endoscopic tools, but instead remotely controls a robot that manipulates the tools. In the da Vinci system, the surgeon holds on to a device that mimics his or her hand motions – like a highly precise version of the Wii™ home video game. The da Vinci system provides surgeons with: (1) the range of motion and fine tissue control available in open surgery; (2) many advantages of endoscopic surgery, including small incisions and less blood loss; and (3) improvements, such as tremor filtration, and 3D magnified vision of the operative field via paired stereoscopic robotic eyes. Among surgical robots, da Vinci is the market leader, with over 2000 systems installed worldwide.26
Another surgical robot system, the ViKY™, is made by EndoControl Medical in France.27 The ViKY, compared to da Vinci, is “simpler, smaller and specialized” according to its manufacturer’s chief executive officer, and costs under $200 000 for four components, including a compact robotic endoscope holder and a uterus manipulator.28 One surgeon performed a hysterectomy single-handedly, using two ViKY components to control a camera and support the uterus, effectively replacing the function of two surgical assistants.28 In plastic surgery, a similar application might be a breast reconstruction with a transverse rectus abdominis myocutaneous flap, which uses an assistant. Recently a surgeon used both the da Vinci and ViKY systems in a total laparoscopic hysterectomy and bilateral salpingo-oophorectomy, which required only four incisions.29
Advantages and disadvantages of robotic surgery versus endoscopic surgery
Robotic surgery has many benefits, including 3D viewing at high magnification, filtering of involuntary hand tremors, digitized recording, improved surgical ergonomics, and (in the case of da Vinci) miniature articulating instruments with seven degrees of freedom. (According to da Vinci literature, there are three external degrees of freedom – external yaw, external pitch, and external insertion (moving the instrument up or down along the vertical axis) – and four internal degrees of freedom – internal yaw, internal pitch, roll, and grip.) In contrast, endoscopic surgery suffers from a reduced range of motion, two-dimensional viewing, and the fulcrum effect. These robotic features provide greater precision and dexterity; smaller incisions; easier tissue dissection, suturing and tumor resection; less trauma and blood loss; and improved quality and safety. A robotic blade can be programmed to cut only a certain depth into tissue, and to avoid wrong-side surgery. Thus, robots reduce human error and promote “slower but safer” surgery. Note Isaac Asimov’s first law of robotics: “a robot may not harm a human being.”30,31
Drawbacks of surgical robots include high price and maintenance costs; uncertain outcomes and litigation; and suitability of the systems for surgeons, including training curve and lack of tactile or force feedback (i.e., the surgeon can’t feel how deep he or she is penetrating into tissue, or how tight a stitch is).32–34 Also, surgical robots are designed to work on single small regions, and the current instruments are too large for plastic surgery. As with any new technology, the benefit–risk ratio should improve with time, experience, and new designs.
Future use of surgical robots in plastic surgery
The fact that robots save on human labor may be controversial (as was the case when robots replaced workers on assembly lines), but ultimately, such savings might offset and justify the purchase of such robots. Surgical robots are available 24/7; and while they need periodic maintenance, they do not require sleep, food, or vacation. They enhance human abilities: one surgeon likened the da Vinci system to operating with four arms simultaneously.35
Robotics in limb and hand surgery
In general, the goal of reconstructive plastic surgery is to maximize both function and cosmesis. Although autologous flaps and grafts are the usual raw materials for these endeavors, robotic parts may sometimes yield better function and cosmesis, while minimizing both convalescence and psychosocial trauma. When salvaging a limb, there may be several expensive operations, after which the patient may be left with a minimally functional limb, multiple donor site morbidities, and months of lost work.36 Therefore, surgeons may consider robotic parts along with reconstructive options from the beginning of a clinical evaluation to ensure the best outcome.
Robotics as a rung on the reconstructive ladder: upper limb example
Plastic surgeons use the concept of a “reconstructive ladder” to describe surgeries of increasing complexity (Fig. 36.3). For example, following an amputation, autogenous tissue reconstruction progresses from secondary healing and primary closure to skin grafting, local flap coverage, distant flap coverage, and free tissue transfer. A robotic prosthesis can be added at any point in the reconstructive ladder. A limb stump may require simple closure or skin grafting for soft-tissue coverage before fitting the patient with a prosthesis. Or the surgeon might perform a free-tissue transfer with vascularized bone for limb lengthening to optimize the final function of a robotic prosthesis.
Current upper extremity prosthetic options
There are seven categories of arm prostheses that vary by complexity and function (Figs 36.4, 36.5):
1. A passive arm prosthesis serves a cosmetic role, and may provide a passive function such as stabilizing, pulling, wedging, and balancing (Fig. 36.5A).
2. A body-powered arm prosthesis has a cable-and-pulley system driven by the patient’s muscle movements to provide active grip and release (Fig. 36.5B,C). Body-powered devices can provide elbow control as well.
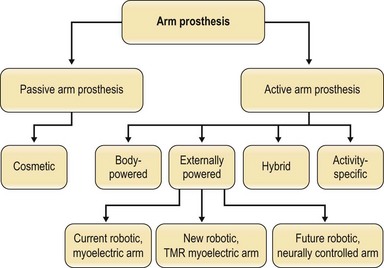
Fig. 36.4 There are seven categories of prostheses that vary by complexity and function. (1) Passive arm prostheses serve a cosmetic role. The 6 types of active arm prostheses include the following: (2) body-powered arm prostheses. (3–5) Externally powered arms, including: (3) current robotic arms with myoelectric control; (4) current robotic arms with targeted muscle reinnervation; (5) future robotic arms with neural control; (6) hybrid arm prostheses, typically combining body-powered and robotic components; and (7) activity-specific arm prostheses. For photos of the prostheses classified here, see Fig. 36.5.
(Courtesy of Joseph Rosen, MD and Erin Donaldson, MS.)
(Reproduced from Burdette TB, Long SA, Ho O, et al. Early delayed amputation: A paradigm shift in the limb salvage time line for patients with major upper-limb injury. J Rehabil Res Dev 2009; 46:38–94.)
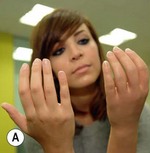
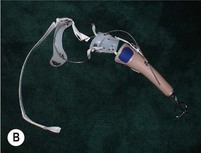
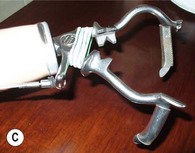
An externally powered (robotic) arm prosthesis can be one of three types:
3. A robotic/fully myoelectric arm – in this arm, the user contracts muscles in the residual limb, causing surface electromyogram electric signals that are translated into controlled movements of the elbow, wrist, or hand.
4. A robotic arm based on a surgical technique called targeted muscle reinnervation (TMR) is currently the most modern attempt at prosthetic control. Surgeons (plastic, general, or neurological) transplant residual nerves from the amputated arm to alternative muscle sites, which then produce electromyogram signals on the surface of the skin that can be computer-processed to control prosthetic arms (Fig. 36.5D).19
5. A robotic, neurally controlled arm – in this design, the user thinks to initiate movement, as with a biological arm, and the brain’s neural signals travel from implants placed in the patient’s brain to external computer software that translates them into controlled movements of the elbow, wrist, or hand (Fig. 36.5E–G). This is a future robotic arm design, now in development.
6. A hybrid arm prosthesis typically combines a lightweight, inexpensive, body-powered proximal arm and elbow with distal robotic components for the wrist and hand to articulate and grasp objects precisely (Fig. 36.5H,I). New robotic prostheses can perform advanced, active functions, such as wielding a fishing rod by flexing the arm while rotating the wrist and grasping with the hand. However, robotic prostheses do not replace the need for the sixth category of prosthesis:
7. An “activity-specific” nonrobotic arm can allow the user to hold a guitar pick, release a bowling ball, or participate in sports such as surfing, golf, swimming, and rock climbing (Fig. 36.5J–L). For example, a surfing prosthesis looks like an arm fin, and can be completely submerged in water.
Robotic hand prostheses and neural arm–hand prostheses
The current state of the art for robotic hands is the i-LIMB ultra, made by Touch Bionics, a myoelectric prosthetic hand with five individually powered digits (Fig. 36.6).20 Because of the independent motors, the hand has six gripping patterns – an advance over the single-grip pattern available in its immediate predecessors. The i-LIMB ultra hand looks and acts like a real human hand in many ways; is lightweight, robust and appealing to both patients and clinicians; and represents a generational advance in bionics and patient care.20 When a partial hand is needed due to a midhand amputation, or several missing fingers, Touch Bionics has a product called i-LIMB digits which contains up to five individually powered (myoelectric) fingers that provide greater grasping ability for partial-hand patients (Fig. 36.6C).20 Touch Bionics also provides a very lifelike cosmetic hand, with functional aesthetic restoration and precise skin-tone matching, called livingskin™ (Figs 36.5A and 36.7). Another bionic hand on the market is the bebionic prosthesis, a fully articulating myoelectric hand with compliant surface and multiple grip patterns that allow precise grasping. The bebionic glove product is a realistic cosmetic cover for the hand (Figs 36.8).21
The Defense Advanced Research Projects Agency (DARPA) undertook a 6-year, $49 million collaborative research project, called Revolutionizing Prosthetics, whose goal was to produce two extremely advanced arm prostheses that would restore motor and sensory capabilities to upper extremity amputees, and would look, feel and perform like the native limb. One device could involve invasive technology, and one had to be completely noninvasive.22 The noninvasive design task was assigned to DEKA Research and Development23 and the invasive task went to a consortium led by Johns Hopkins University Applied Physics Laboratory (APL). DEKA’s “Luke” arm, now in clinical trials, is modular in design, and contains a hand that is similar in function to the i-LIMB, with six grip patterns, and myoelectric (not neural) control.23 The Johns Hopkins consortium collaborated with many academic and corporate partners in the difficult task of extracting motor neural signals and sensory feedback to control an arm and hand solely by the intent of the user.24 The project specified a five-fingered hand, an opposable thumb, a wrist, elbow, and shoulder, which together could generate over 22 ranges of motion, and resulted in the modular prosthetic limb (MPL) design (Fig. 36.5E–G). Following the Revolutionizing Prosthetics program, DARPA, APL and consortium members have continued the research, and the arm is now in FDA Phase III testing in human subjects. This arm may even allow quadriplegic patients to recapture the use of limbs, potentially bypassing spinal cord injury by connecting their thoughts directly to a bionic limb.25
Choosing a robotic arm: benefits and limitations
A robotic limb will benefit a patient when it provides more function and cosmesis than a surgically reconstructed limb. Generally, for an injury that is more severe, and closer to the midarm, a robotic arm will be an appropriate choice (Fig. 36.9). In the appropriate patient, a prosthetic arm can be the difference between a dependent existence and an independent return to society.
The plastic surgeon’s role, besides helping the patient choose and order the right prosthesis, is to follow the patient through rehabilitation, with the help of an anaplastologist and/or prosthetist, who can be found via the International Anaplastology Association and the American Academy of Orthotists and Prosthetists.37,38 The surgeon should contact a prosthetist soon after the trauma, before final wound closure, to discuss socket and terminal device design and fabrication. The prosthetist will inform the surgeon that sometimes more length is a detriment to including prosthetic components, but not enough length will conversely hurt suspension of the socket. The surgeon will need to give the prosthetist a prescription for the prosthesis, and they will discuss the patient’s treatment plan together. If the patient needs a replacement limb with a lifelike covering, an anaplastologist will collaborate with the prosthetist to provide cosmesis as well as function, creating a lifelike skin to cover the prosthesis.
Lower limb prostheses
Lower limb prostheses that employ computerized, robotic, or active technology are in development, including both leg and foot designs.39 The Massachusetts Institute of Technology has designed a myoelectric ankle–foot prosthesis that can walk on level ground or climb stairs; in the future they hope to have neural signals automatically trigger terrain-appropriate, local prosthetic leg behaviors.40 The C-leg® by Otto Bock Healthcare has a robotic knee that employs a microprocessor, software, and feedback from 50 sensors to anticipate the user’s movements and adjust motion in real time, to keep the knee stable as the user walks and maintain support even if he or she stumbles.41
Robotics in craniofacial surgery and ear, nose, and throat surgery
Dr. Paul Tessier of France pioneered craniofacial reconstruction surgery, developing many operations to correct mid-facial defects in children in the 1900s.42,43 The field was reinvented in 2005, when Dr. Jean-Michel Dubernard in France performed the first partial face transplant. Dr. Maria Siemionow and a surgical team at Cleveland Clinic performed the first US near-total human facial transplant in 2008;44 Dr. Bohdan Pomahac and a team at Brigham and Women’s Hospital conducted the second US facial transplant in 2009;45 and others have followed around the world, progressing to full facial transplants in 2010. Composite tissue transplantation advances the craniofacial surgeon’s skill set, and robotics has the potential to advance the field further. Major craniofacial remodeling operations might be done in a minimally invasive fashion, using only a few small incisions, if the miniature, articulated, remotely controlled instruments from the da Vinci system were adapted to perform such procedures.
Other surgical specialists are exploring this area. Selber et al. conducted a preclinical investigation to explore the feasibility of robotic reconstruction of oropharyngeal defects; they stated that, “transoral robotic free flap reconstruction is the next logical step in transoral robotic surgery.”46
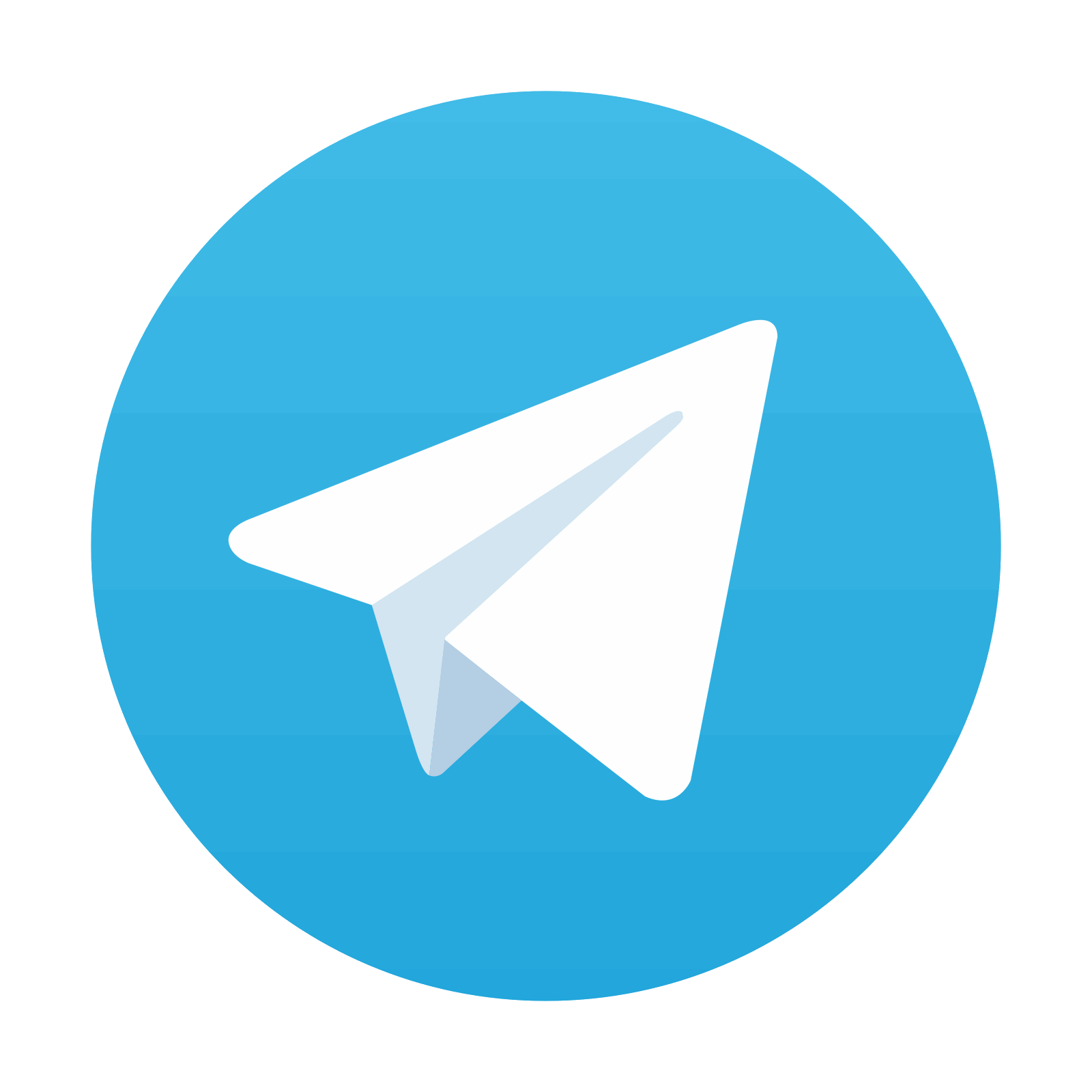
Stay updated, free articles. Join our Telegram channel

Full access? Get Clinical Tree
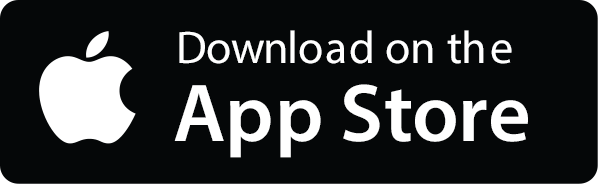
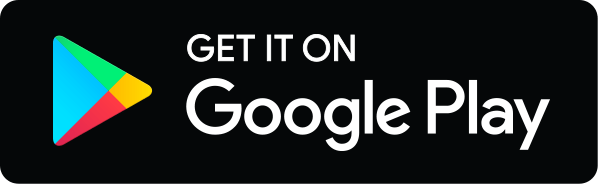