27 Principles and applications of tissue expansion
Synopsis
Tissue expansion is a time-tested, proven, simple technique to generate tissue to reconstruct defects.
The technique stretches skin and soft tissue that have the same color and texture as the adjoining skin where expanded tissue is needed.
In the breast, expansion is particularly useful in stretching existing anatomy to accommodate a permanent prosthesis.
Introduction
There are various methods for expansion of skin, bone, and other tissues. Placement of a prosthesis under soft tissues allows the surgeon gradually to add saline and expand subcutaneous and cutaneous tissues. External hardware is used by surgeons to expand fractured bone gradually through the application of distraction force. The techniques have been applied to the craniofacial skeleton as well as to most of the long bones of the body.1,2 Vacuum-assisted closure (VAC) uses this principle of applying force to the cells surrounding a wound to stimulate the induction of new tissue that will subsequently close the wound.3
Knowledge advances in tissue expansion principles are discussed below.
Historical perspective
In 1905, Codvilla applied the principles of using an external, distractive force to encourage tissue expansion in bone.4 Bone tissue regeneration was later documented in 1970 by Ilizarov and others, who provided roentgenographic and morphologic data from experimental distraction epiphysiolysis.2 Shortly thereafter, Matev reported the expansion of bony tissue after amputation of the thumb at the metacarpophalangeal joint.5
In the meantime, physicians began to recognize the induction of new soft-tissue growth adjacent to the bony structures undergoing gradual lengthening. In 1957, Neumann implanted a subcutaneous balloon to induce soft-tissue growth purposefully for the reconstruction of an external ear deformity.6 Unfortunately, his report was considered anecdotal, so progress in surgical, soft-tissue expansion was delayed until the 1982 reports of using implanted silicone balloons to expand overlying soft tissue were published.
Radovan7 and Austad and Rose8 simultaneously developed the concept of implanting silicone balloons as expanders. Austad’s prosthesis was a self-inflating device that used osmotic gradients driven by salt placed within the expander. His largely experimental work was critical to elucidating the physiology of tissue expansion.9
Radovan’s device contained a self-sealing valve through which saline was periodically injected to increase the size of the expander. His work was initially greeted with skepticism. Despite this, Grabb’s enthusiastic acceptance of Radovan’s technique stimulated its rapid and wide application to create new horizons in reconstructive surgery.10–15 Large studies subsequently confirmed the safety and efficacy of this technique.
Biology of tissue expansion
Extensive information is available regarding the biology of tissue expansion. After animal experiments were completed,9,16 studies on human tissue examined the process of tissue expansion that occurs both during the period of expansion and postoperatively.17 Studies of the effects of tissue expansion on nerve, muscle, and bone have also been published.
Skin
The increased area of skin over the expander includes not only normal skin recruited from adjacent areas but also new skin generated by increased mitosis.18 Hair follicles are not reproduced in humans, so individual follicles are distracted during expansion. Distractions between follicles are less noticeable in blonds than in dark-haired individuals. Melanocytic activity is increased during expansion but returns to normal levels within several months after completion of the reconstruction. Although hair follicles and accessory skin structures are compressed by tissue expansion devices, they show no evidence of degeneration.
During expansion, the dermis decreases rapidly in thickness over the entire implant. Thinning is most pronounced in the first several weeks after implant placement and persists for the entire period of expansion. Dermal thinning persists at least 36 weeks after expansion is completed in human tissue.19
Muscle
Muscle atrophies greatly during the process of expansion, whether the prosthesis is placed above or below a specific muscle. The effects on human muscle after expansion during breast reconstruction have shown occasional histologic ulceration. Focal muscle fiber degeneration with glycogen deposits and mild interstitial fibrosis has been noted. Some muscle fibers show disorganization of the myofibrils in the sarcomeres.20 Animal studies on the histomorphologic changes in skeletal muscle suggest that the expansion of skeletal muscle is not a stretching process but is rather a growth process of the muscle cell accompanied by an increase in the number of sarcomeres per fiber. Expanded skeletal muscle repairs normal muscle architecture, vasculature, and function after the prosthesis is removed.21 Muscle mass returns to normal levels after removal of the device in humans.
Bone
The effect of expansion on underlying cranial bone has been studied in the animal model.22 Decreases in both bone thickness and volume in cranial bone are evident beneath the expander, where osteoplastic bone resorption occurs. In contrast, there is an increase in these predominantly at the expander periphery, where a periosteal inflammatory reaction is seen. Bone density is unaffected.
Vascularity of expanded tissue
The robust vascularity of expanded tissue was clinically in evidence long before laboratory work measured it (Fig. 27.1). It has been clinically and histologically demonstrated that a large number of new vessels are formed adjacent to the capsule.23
Angiogenesis occurs in response to induced ischemia of the expanded tissues. The number of cells expressing vascular growth factor is significantly higher in expanded tissue than in nonexpanded, similar tissue.22 Expanded fascial flaps show a measurable increase in vascularity between the fascia and subcutaneous tissue. Increased perfusion to the distal and peripheral areas of the flap also increases the robustness of the flap23 and the possibility of harvesting a larger flap. Similar studies on prefabricated, expanded, pedicled flaps have shown increased vascularity within the pedicle, as well as in the surrounding, adjacent, random area.
The increase in vascularity affords the expanded tissue important functional benefits. Animal studies have shown that flaps elevated in expanded tissue have significantly greater survival areas than acutely raised and delayed flaps (Fig. 27.2).24 Similar studies employing labeled microspheres have demonstrated an increase of flap survival, as well as increased blood flow in the expanded tissue.15
Cellular and molecular basis for tissue expansion
The application of mechanical stress to living cells affects various cell structures and signaling pathways that are highly integrated (Fig. 27.3).25,26 These closely integrated cascades are theorized to explain the generation of new tissue through mechanical stimulation.26 Several in vitro stretching systems have been used to understand better the molecular events that occur.27
Mechanical deformation forces involve several cellular mechanisms including the cytoskeleton system, extracellular matrix, enzyme activation, secondary messengers, and ion channels. The cytoskeleton plays a critical role in mediating the transformation of extracellular mechanical force to intracellular events. A system of microfilaments within the cytoplasm not only maintains intracellular tension and cell structure but also transduces signals to adjacent cells and initiates transduction cascades within the cell (Fig. 27.4).26
Protein kinase C plays a pivotal role in signal transduction. Mechanical strain on cell walls activates inositol phosphatase, phospholipase A2, phospholipase D, and other messengers. Activation of these components leads to activation of protein kinase C, which is, in turn, associated with activation of proteins; presence of this protein activation cascade suggests that intracellular signals can be transmitted to the nucleus. Protein kinase C activation has been noted in human cells subjected to strain in vitro.26
Many growth factors, including platelet-derived growth factor and angiotensin II, play a role in strain-induced cell growth.28 Extensive laboratory studies now underway are attempting to quantify and determine the interrelationships of these two, complex molecules. Downstream of the cellular membrane molecules, several common pathways are activated by expansion via both growth factors and mechanical strain; these pathways affect both the cytoskeletal system and protein kinase families.
Implant types
Self-inflating expanders
Self-inflating expanders have become available largely through Europe; these contain osmotic hydrocolloids that cause migration of extracellular water through the silicone membrane of the device. The first such expander was devised by Dr. Austad and was used experimentally; however, it was not approved by the Food and Drug Administration and is not available in the US.8
An osmotically active hydrogel expander has been developed and used in expansion of the orbit for management of microphthalmia and anophthalmia.29 Chummun and associates recently reported their experience using the osmotic tissue expander in patients. Their implant draws sufficient water from the adjacent tissues, spontaneously expanding the prosthesis up to 10 times its original volume.30
Basic principles
Incision planning and implant selection
Choice of an implant with an external distal port may affect surgical planning. Cultures of implants with external valves revealed that 82% of these prostheses had colonized the expander capsule and had some infection present; this constitutes an infection risk that is slightly higher than that of totally buried prostheses.31 Although patients tolerate this colonization well and experience few complications, externalized ports are contraindicated when a permanent prosthesis or bone grafts are to be used after expansion is complete.
Implant inflation strategy and technique
Frequent small-volume inflations are better tolerated and are physiologically more suited to the development of adequate overlying tissue than are large infrequent inflations. For practical purposes, most prostheses are inflated at weekly intervals. On occasion, accelerated inflation schedules may be followed.32 In children who have devices with external ports, small-volume inflation at 2–3-day intervals is well tolerated. Individual inflations proceed until the patient experiences discomfort or blanching of the overlying skin. In hypoesthetic areas, objective changes in flap vascularity must be evaluated with particular care. Although a variety of devices such as pressure transducers and oxygen tension monitors are available to help determine proper inflation; an objective inspection of the patient’s response is usually a reliable indicator of appropriate inflation. Serial inflations proceed until an adequate amount of soft tissue has been generated to accomplish the specific surgical goal.
Tissue expansion in special cases
Burns
Skin that has suffered a partial-thickness burn or that has been scarred by adjacent burns is attenuated and is more amenable to expansion.33 Incisions can be placed in previous scars, but the scar should be mature and relatively thick so that extrusion does not occur. Using an access incision within normal tissue that is peripheral to the burned area further minimizes the risk of extrusion. The principle of using multiple, smaller-volume prostheses is especially appropriate in burn patients. Perioperative antibiotics and meticulous preparation are always used because the incidence of infection is higher in burn patients.
Tissue expansion in children
Skin and soft tissue are always thinner in children than in adults. These tissues are probably better vascularized but less resistant to trauma. Tissue expansion has a higher complication rate in children than in adults.34 Rather than using excessively large prostheses or expanding tissue aggressively, accomplishing serial repeated expansions may be helpful in children. This is done with the understanding that major complication risk – particularly extrusion – is more common at the second, third, and fourth serial expansion.35 This is particularly true in the head and neck (with the exception of the scalp). Expansion of the facial areas and neck can be particularly difficult.
Expansion of myocutaneous, fascial, and free flaps
Myocutaneous flaps are the standard of care for the treatment of large defects, particularly when bone and vital structures are involved. The territories of standard flaps are well described. These territories can be considerably enlarged by placing an expander beneath the standard myocutaneous flap, and an extremely large flap can be developed over a short period. Expansion increases the vascularity of the flap and allows a large, adjacent random area to be carried with the original flap.36 The vascular pedicle of such flaps remains intact and may in fact be elongated, thus allowing flaps to be transferred farther.
Myocutaneous flaps such as the latissimus dorsi and pectoralis can be expanded to almost double their surface area, allowing coverage of almost any defect on the abdomen or thorax.37 Expanders of up to 1000 mL can be placed beneath such flaps and rapidly expanded. For example, bilateral latissimus dorsi myocutaneous flaps can be expanded and moved to the midline to cover large meningoceles or the vertebral column. The expansion prostheses in these cases are placed under the latissimus dorsi muscle through incisions in the lateral margin of the muscle.
Fasciocutaneous flaps can be expanded either before or after transposition. When flaps are expanded before transfer, it is best to keep the prosthesis as far away from the pedicle as possible, thus preferentially expanding the random area of the flap. Within 6 months of transfer of these flaps, the random blood supply is usually sufficiently established to allow placement of the expander anywhere under the flap.38
Total facial reconstruction with an extraordinarily large flap has been accomplished using a pre-expanded bilateral parascapular free flap. Use of this strategy allows one large aesthetic unit to be moved.39,40
Reconstruction in the head and neck
The head and neck area contains many specialized tissues that must be matched appropriately to achieve optimal aesthetic reconstruction. Aesthetic reconstruction is maximized by mobilization of adjacent local tissues rather than by transfer of distant tissues with poor match of color, texture, or hair-bearing capability. Tissue expansion therefore allows optimal aesthetic reconstruction by use of a similar adjacent tissue area to reconstruct a defect without creation of a donor site.11,41
The skin of the face can be subdivided into five tissue-specific areas:
1. The scalp is unique in that it contains specific hair-bearing qualities that cannot be mimicked by any other tissue of the human body.
2. The forehead is a continuation of the scalp, but it is distinguished from the scalp by its thick skin, large number of sebaceous glands, and lack of hair.
3. The nose is embryologically related to the forehead, so it closely mimics the forehead in color, texture, and sebaceous gland content.
4. The lateral cheek areas, neck, and upper lip have fewer sebaceous glands; the skin is thinner, and the hair-bearing pattern is significantly different in quality and quantity from that on the remainder of the body.
5. The skin of the periorbital areas is extremely thin and pliable, containing a minimal number of sebaceous glands.
Scalp
Tissue expansion is the ideal procedure for the reconstruction of scalp defects (Fig. 27.5).42 Expansion of the scalp is well tolerated and is the only procedure that allows development of normal hair-bearing tissue to cover the areas of alopecia. The amount of scar and deformity generated is considerably less than with previous procedures such as serial reduction and complex multiflap procedures.
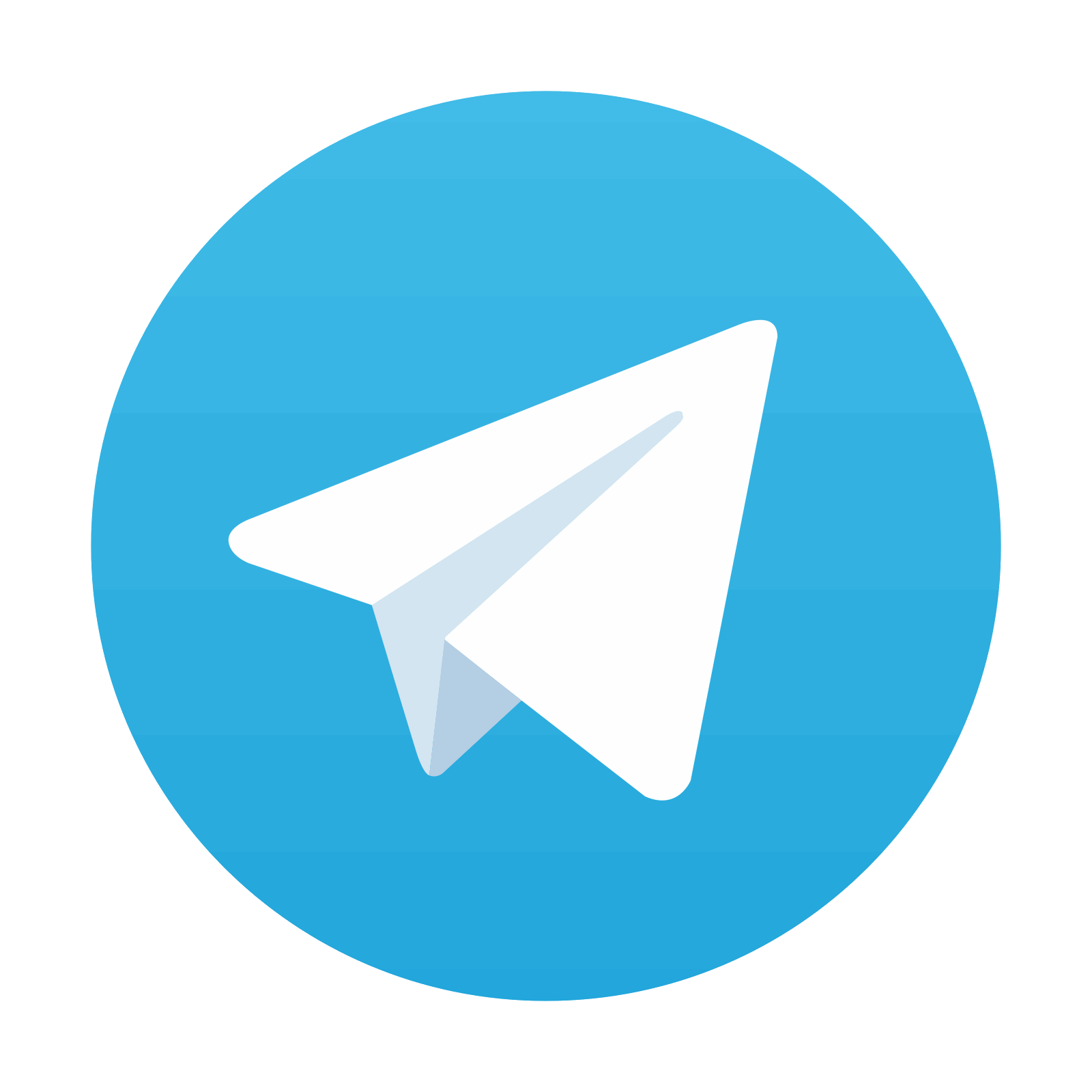
Stay updated, free articles. Join our Telegram channel

Full access? Get Clinical Tree
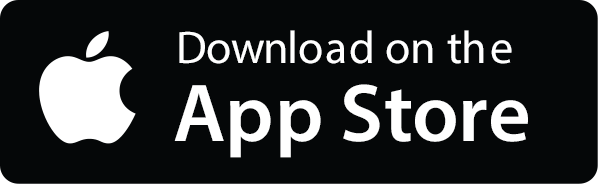
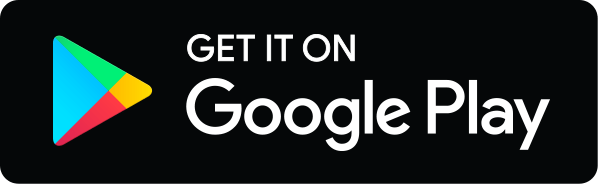