32 Peripheral nerve injuries of the upper extremity
Synopsis
Nerve regeneration after injury is orchestrated by delicate processes in neurons, nonneural cells, and other cells at various levels from the periphery to the central nervous system.
The clinical effects of a nerve injury include not only impaired sensory and motor function, but also symptoms such as impaired dexterity, sensitivity to cold, and pain. Therefore, they have a severe impact on activities of daily living.
Correct diagnosis and classification of the injury are crucial for appropriate treatment.
The surgical approach and technique, as well as timing of surgery, are factors of detrimental importance for outcome.
The type of injury, condition of the wound, and the vascularity of the wound bed are key factors influencing surgical decision-making.
Various techniques for nerve repair are described, including epineurial, fascicular, and end-to-side repair, and techniques for nerve reconstruction.
Factors of importance for outcome, such as age, delay before repair, and level of injury are discussed, as well as means of evaluation after surgery.
Introduction
A nerve injury may have a profound impact on the patient’s activities of daily living, with subsequent effects on professional life and leisure time. Patients may have to change profession, or be left with a permanently impaired ability to work. Nerve injuries may, therefore, generate not only costs for society within the healthcare system for treatment and rehabilitation, but also outside the healthcare system resulting from lost production.1 Nerve injuries most commonly affect the upper extremity. The incidence of injuries to the hand has been calculated as 7–37/1000 inhabitants/year in Europe.2 Similar data are available for children (incidence 2.7/1000 children/year). Most hand injuries are minor, but injuries to the nerves (that account for about 3% of hand injuries) usually impair hand function.2,3 Few reports exist about the specific incidence of nerve injuries, but the incidence has been reported to be 13.9/100 000 person/year.4 Most of the injuries to the hand (10%) and the wrist (63%) required less than a week in hospital. However, few inpatient and outpatient data have been reported. The incidence of a digital nerve injury is estimated to 6.2/100 000 inhabitants/year. The general pattern of distribution of both hand and nerve injuries between the sexes is that it is usually young (median age 29 years) men (up to 75%) who are injured. The incidence of a radial nerve injury associated with a fracture of the shaft of the humerus is around 0.12/10 000 inhabitants and year.5 Based on these reports one may estimate that 70 000 and 29 000 patients in the European Community and in the US, respectively, injure a nerve trunk annually.
The costs of treatment and rehabilitation, including those for loss of production, after a nerve injury, may be substantial. The costs for injuries to a median and an ulnar nerve are roughly $70 000 USD and $45 000, respectively1; 87% of these costs are the result of lost production. Costs are higher for patients with coexisting tendon injuries (≥4 tendons). In addition, costs within the healthcare sector are higher for patients who had to change jobs after the injury and in patients in whom both the median and the ulnar nerves were injured. Up to 69% of patients with an ulnar or median nerve injury are back in fulltime work by 1 year after the nerve injury.1,6,7 Age is one important factor for outcome after nerve repair,1,8 which is probably based on better cerebral adaptation to the injury, particularly in young children. The clinical effects of a nerve injury include not only subjective impaired sensory and motor functions, but also subjective complaints such as impaired dexterity, cold sensitivity, and pain9; thus, having a severe impact on activities of daily living. Altogether, nerve injuries can cause considerable problems for individual patients and costs for society. In the present chapter the principles for repair and reconstruction of nerve injuries are outlined, with emphasis on the fact that such injuries should be treated promptly.
Basic science and natural history
Anatomy
Gross anatomy: the upper extremity
The brain and the spinal cord (central nervous system) are connected to targets by the peripheral nervous system, which consists of cranial nerves, spinal nerves with roots and rami, and peripheral nerve trunks with the peripheral components of the autonomic nervous system. The anterior and posterior nerve roots (that emerge from rootlets attached to the spinal cord) merge into the spinal nerve, where the anterior primary rami form the brachial plexus in the upper extremity (Fig. 32.1). The posterior primary ramus runs posteriorly, and supplies the muscle and skin of the posterior part of the neck and trunk; it does not enter the extremity. The anterior primary rami of the cervical nerve roots C5–8 and the ramus of T1 form the brachial plexus, from which the upper extremity receives its innervation through various branches.
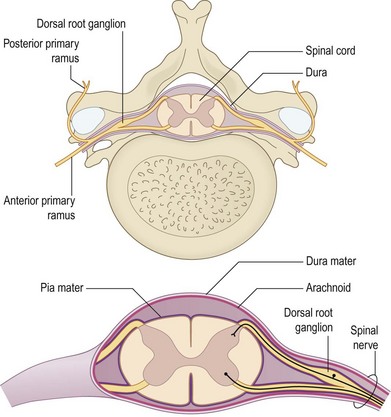
Fig. 32.1 The anterior and posterior nerve roots, which emerge from rootlets attached to the spinal cord.
C5–6 form the upper trunk of the brachial plexus, C7 forms the middle trunk, and C8–T1 form the inferior trunks. These trunks divide into anterior and posterior divisions in which the lateral and medial cords are formed from the anterior division while the posterior divisions form the posterior cord, which mainly innervates the extensor muscles in the upper extremity. In contrast, the lateral and medial cords innervate the flexor muscles in the extremity. The musculocutaneous nerve originates from the lateral cord. The median nerve is formed by the joining of the lateral and medial cords, whereas the ulnar nerve branches from the medial cord together with the medial cutaneous nerves to the arm (brachial) and forearm (antebrachial). The radial and axillary nerves originate from the posterior cord. There is a segmental pattern of sensory and motor innervation (Fig. 32.2).
The anatomy of the plexus and the nerve trunks may vary considerably from person to person. Variations are seen in the forearm, where a “Martin Gruber” anastomosis may be found in as many as 15%, particularly between the anterior interosseous nerve and the ulnar nerve. A similar anastomosis may also be present more distally – Riche–Cannieu (branch between the motor branch of the ulnar nerve and the thenar branch of the median nerve in the thenar region in the hand: Fig. 32.3).
The neuron and supporting cells
The cell bodies of the motor and sensory neurons are located in the spinal cord and in the dorsal root ganglia, respectively, with their axons extending out to their respective targets in the periphery. The sensory neuron is pseudounipolar, with one branch of the axon extending into the posterior part of the spinal cord. The transition from the central to the peripheral nervous system takes place in the rootlets (or less often, in roots of the nerves) of the transitional zone, which are almost cone-shaped.10 In the central part, the neurons are surrounded by oligodendrocytes and astrocyte processes, whereas in the peripheral nervous system axons are closely associated with Schwann cells. In myelinated nerve fibers, each axon is embraced by a series of continuous Schwann cells that create the myelin sheath. The border between individual and enwrapping Schwann cells is called the node of Ranvier (Fig. 32.4). Each nerve fiber is enclosed in a basal lamina (or basement membrane). In contrast, several thinner axons run in troughs in one Schwann cell – nonmyelinated nerve fibers. Each Schwann cell therefore embraces several axons. The diameter of nerve fibers varies, and extends from 0.4 to 1.25 µm (nonmyelinated) and from 2 to 22 µm (myelinated).11 The number of nerve fibers also varies with nerve trunks. In addition, the number of nerve fibers decreases with age: as many as 26% of the nerve fibers may disappear between the second and eighth decades.
The nerve trunk
A number of nerve fibers are enclosed in bundles around which there are flattened supporting cells and layers of collagen that form the perineurium. These bundles of fibers surrounded by the perineurium are called fascicles, which are embedded in loose connective tissue (called epineurium); this is composed of collagen fibers and fibroblasts (Figs 32.4 and 32.5). The space inside the fascicles is called the endoneurial space, in which the pressure is slightly positive (endoneurial fluid pressure); it consists of collagen fibrils, and cells such as fibroblasts and occasional macrophages and mast cells. Lymphocytes (CD4+ and CD8+) and macrophages are not generally found in the endoneurium (except after injury), but may be present in the epineurium. The amount of connective tissue varies among nerve trunks, and also within the same nerve trunk. More abundant connective tissue is therefore located in the nerve trunk at sites where extra protection is needed (such as joints and superficial nerve trunks). The term “mesoneurium” refers to the additional loose areolar tissue that is located superficially to the epineurium. This tissue allows for the gliding of the nerve trunk (excursion) during movements of the extremity.
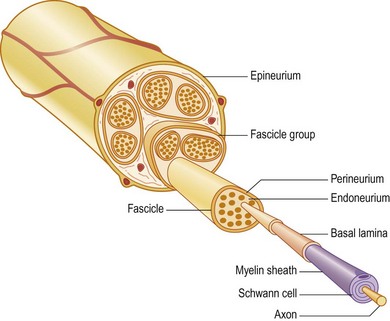
Fig. 32.5 Schematic presentation of a peripheral nerve. Colors for identical structures are the same as in Figure 32.4.
There is topographical segregation of fascicles and nerve fibers in the nerve trunks, particularly in their peripheral parts.12,13 The latter anatomical feature permits dissection of a bundle of fascicles that can be used in nerve transfers.
Blood supply
Nerve trunks receive their blood supply from segmental blood vessels (Fig. 32.6), which branch into plexuses in the epineurium, perineurium, and in the endoneurial space. The blood vessels inside the fascicles consist mainly of capillaries, which are provided by circulation through blood vessels that pierce the perineurium obliquely. The epineurial blood vessels are more susceptible to trauma than the endoneurial vessels and so epineurial edema develops more easily than endoneurial edema. Because of the extensive reserve capacity of the intraneural blood supply it is possible to mobilize a nerve over an extended length without disturbing the blood supply. In addition, the blood vessels adjacent to the nerve trunks have a coiled appearance that allows excursion of the nerve trunk. In addition to the vasa nervorum, there are also nervi nervorum, such as free nerve endings in the epineurium, perineurium, and endoneurium.
Physiology
To allow for propagation of the action potential, ions are exchanged between the axon and the extracellular space at the nodes of Ranvier (Fig. 32.4). As this exchange occurs only at the nodes of Ranvier in myelinated nerve fibers, the conduction velocity jumps from node to node. In myelinated nerve fibers this saltatory conduction induces high conduction velocity. In contrast, the propagation of impulses is more continuous in nonmyelinated nerve fibers. After a nerve has been injured the process of Wallerian degeneration (see below) takes some time in humans, which explains why it is possible to record propagation of an impulse in an injured nerve by electrical stimulation at neurography for many days after the injury. It is generally agreed, therefore, that neurography should be done no earlier than 3–4 weeks after the injury if signs of degeneration are to be found.
The distal nerve segment
The timing of nerve repair is of crucial importance for a successful outgrowth of axons after injury,14,15 which can be related to a large number of factors that are up- and downregulated by Schwann cells after nerve injury. After the Schwann cells have been activated they proliferate along the inside of their basal lamina, and form the bands of Büngner.
Apoptosis (programmed death) of various cells, such as macropahges and Schwann cells, an event that is initiated by release of proapoptotic molecules from the mitochondria (intrinsic pathway), can be activated through the death cell surface receptors (extrinsic pathway; like Fas and tumor necrosis factor receptor 1). The number of apoptotic Schwann cells in the distal nerve segment is increased if the transected nerve is repaired after a delay.16
All these changes in the injured neuron and its axon and in the Schwann cells have the common goal of regrowth of the axons into the distal nerve segments down to their targets. Regrowth is a delicate process in which numerous sprouts emerge from the distal part of each axon. On the tip of each sprout there is a growth cone, which is formed as a small hand on which the “finger-like” filopodia palpate the environment with the purpose of finding guidance structures (Fig. 32.7). Intricate mechanisms direct rate and direction of extension of the growth cones, where actin filaments are polymerized and organized.17,18 Microtubules are polymerized in the growth cones. The growth is a dynamic process, where the filopodia/growth cone is repulsed or attracted depending on the local mechanisms and the structure of the environment.
Diagnosis and presentation
Formal classification of injury
Nerve injuries can be divided into two main groups: those that will temporarily block nerve conduction without loss of axonal architecture, and those with severe damage that will result in axonal degeneration distal to the injury (Fig. 32.8). This may be one useful clinical way of approaching nerve injuries, but more formal classifications have been presented to describe the complexity of the lesions further, in particular the Seddon classification,19 in which injuries were divided into three groups of increasing severity (neuropraxia, axonotmesis, and neurotmesis). This classification was modified by Sunderland20 and by Mackinnon21 to include six degrees of injury. In Table 32.1 we summarize the differences between the three classifications, and the specific neural changes are illustrated in Figure 32.9.
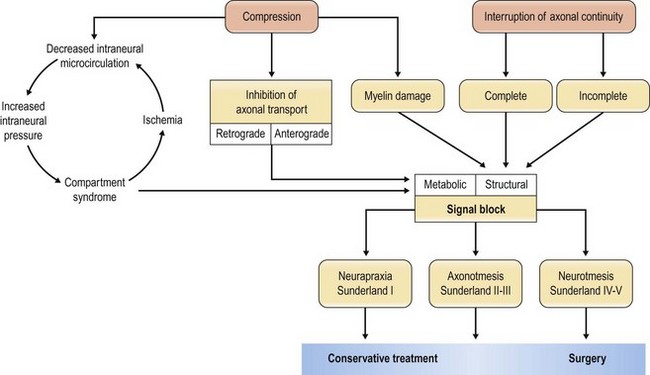
Fig. 32.8 Schematic flowchart of neuronal response to nerve compression and interruption of axonal continuity.
Neurotmesis
Sixth-degree injury
The sixth-degree injury was added by Mackinnon to take account of mixed injuries,21 for example those that occur after closed traction damage to a nerve, or gunshot or stab wounds that cause partial injuries to a nerve. This type of injury is often referred to as a neuroma in continuity, in which all degrees of nerve injury, from normal to neurotmesis, may coexist within the scarred nerve. A neuroma in continuity typically results from failure of the regenerating nerve growth cone to proceed beyond the injury to reach its peripheral targets. It occurs within an intact nerve with continuity in response to internally damaged axons and fascicles, resulting in a distal portion of the nerve that no longer, more or less, functions properly. Surgical exposure is often necessary and intraoperative electrodiagnostic studies can help to distinguish fascicles with signs of recovery from those without (grades IV and V).
Clinical examination
A correct diagnosis is crucial. Confirmation of a nerve injury is usually not hard, even though every nerve injury is unique in its character, and highly variable in its symptoms. Lack of active movement, such as against resistance, because of paralysis of the muscles supplied by the nerve, can be seen immediately after the injury if the nerve is completely transected. However, when motor defects are minor or overshadowed by more striking sensory deficits, the diagnosis can sometimes be overseen, particularly in an incoherent patient. The patient’s histories, and understanding of the mechanism of the injury, are therefore important for the evaluation of the injury and identification of those that will be improved by operation. It is important that every patient with a dysfunctional nerve and a wound overlying the course of a nerve is regarded as having a transected nerve until proved otherwise (Fig. 32.10).
Electromyography/neurography
In case of confusing diagnostic signs, electrophysiological studies can help to establish the diagnosis and to identify injured nerve branches, although the results must be interpreted with caution during the first 3–4 weeks. It may take up to 4 or even 6 weeks after the injury before clearly detectable signs of nerve degeneration and muscular denervation can be detected.22 It is stressed that waiting for an electrophysiological examination should not delay exploration of an injured nerve if indication for surgery is strong.
Intraoperative conduction studies can be of help, particularly in the case of a previously closed injury that has failed to show satisfactory recovery at follow-up. Intraoperative examination enables a higher grade of specificity as the observed nerve or even individual nerve fascicles can be anatomically isolated, and action potentials across the scarred area can be evaluated.23 If electrical recovery is recorded over the area of interest the patient can be classified as Sunderland grade II or III, in which case spontaneous full recovery is expected. If no electrical recovery can be seen, the patient should be graded Sunderland grade IV and V and will most likely benefit from nerve resection and grafting. However, in the case of a neuroma in continuity (sixth-degree injury), the picture is more complex. Some fascicles will need resection and grafting, whereas others are actually grade II–III and will recover spontaneously. In these cases postoperative outcome may be uncertain and the decision to operate is hard as intervention may result in a tedious and potentially damaging dissection with internal and external neurolysis and resection of damaged sections. However, it may sometimes be possible to distinguish injured fascicles that require nerve grafting from intact healthy fascicles preoperatively. Preoperative electrophysiology is therefore warranted and extremely helpful in decision-making during the procedure.
Patient selection
All patients with a suspected nerve injury should be judged individually as a number of factors must be considered before the decision to explore is made (Fig. 32.10). When one is considering sharp lacerations, traction injuries, blast injuries, or a combination, some key factors must be considered that will be of decisive importance in the timing of operation and the approach, including the type of injury and condition of the wound, and the vascularity of the wound bed.24,25
Type of nerve injury
In cases where there are clinical signs of nerve injury but there is no wound, the treatment should be conservative for the first 4–6 weeks, when clinical signs of recovery of function can be investigated. If at this point there has still not been a complete spontaneous recovery, electrophysiological examination can be done. This may be repeated if there is only partial, but insufficient, recovery before any operation is considered. In case of a closed injury where no recovery has been seen at all after 3 months, clinical and electrophysiological examination should be considered.26 If there are no signs of spontaneous recovery during this period, exploration of the injured nerve is indicated. However, if there is partial recovery during the first 3 months, regular re-evaluation may be indicated for up to another 3 months.27 The important point is to see if any recovery at all is present.
Nerve injuries with open wounds should always be explored. If the nerve is in continuity it should be treated as a closed injury, and the clinical and electrophysiological function should be evaluated for up to 3 months. In contrast, a clear-cut injury with the nerve ends closely approximated is suitable for immediate repair, whereas a crush injury usually is not. In the latter case, there might be difficulties in distinguishing between viable and nonviable tissue, which would call for careful debridement before any nerve repair is tried. In addition, when the patient presents with signs of an incomplete injury for which there is a chance that spontaneous recovery can yield a better outcome than immediate repair, reconstruction is preferred at a later stage only if the clinical results of conservative treatment are not satisfactory. Therefore, one of the most challenging aspects of a nerve injury will be the decision-making regarding timing of nerve exploration and reconstruction in complex cases, such as open crush injuries or gunshot wounds. In these cases the primary goal should always be to go for careful debridement and viable tissue coverage. Nerve reconstruction can thus only be considered when the surrounding tissue provides a viable environment for the nerve (see Condition of the wound, below). In case of an open injury, where the patient presents with a sensory and/or motor function deficit, but with a macroscopically intact nerve upon surgical exposure, a close follow-up is essential for assessment of the nerve function. Such cases, which include open wound crush injuries and gunshot wounds, should, as the surrounding tissue envelope has healed, be treated the same way as closed traction injuries (Table 32.2). Neurological examination is performed regularly, sometimes together with recurrently performed neurophysiological testing, and surgery is usually performed if no signs of regeneration or reinnervation occur (e.g., for some injuries, exploration after 3 or 4 months). It is, however, important to stress that all open wounds, where the patient presents with a sensory or motor loss, should be explored in the acute setting, in order to obtain the best outcome.
Treatment and surgical techniques
Immediate compared with delayed nerve repair
General principles
Any nerve injury may be repaired immediately, or after a certain delay. If the conditions are favorable, immediate primary suture is the method of choice, resulting in the most favorable outcome,61 as based on the neurobiological temporally diverse events outlined above. One of the most important prerequisites for primary repair is that it must be completed with little or no tension,29,30 otherwise a nerve graft should be considered.31 Several authors have argued that more than 80% of all nerve lesions can be sutured immediately with a primary end-to-end coaptation.32,33 In these studies, it had to be possible to adapt the nerve endings with a single 9/0 nylon suture, without either separating the endings from each other or tearing the epineurium, to qualify for a primary end-to-end suture.
Primary immediate repair is technically easier than delayed repair, as approximation by rotation of the distal and proximal ends is more obvious when the epineurial vessels are present and clearly visible.34 The elastic recoil of nerve endings that inevitably occurs as the nerve is injured is also easier to overcome in the acute phase. As time passes, the nerve endings will become embedded in fibrous scar tissue and end-to-end coaptation will be more difficult without tension or more extensive mobilization.
Timing
Immediate repair is preferable within the first 48 hours, but some authors have suggested that it may also be done successfully during the first 1–3 weeks35; however, recent experimental neurobiological data have clearly pointed out the advantage of early nerve repair. This implies that patients who present with uncomplicated open nerve injuries that happened more than 48 hours previously benefit from nerve repair that is delayed in the sense that it is done after the wound has healed completely. Practically, the implication is that the nerve should be repaired as soon as possible, preferably within 2–3 weeks of the injury. The rationale for this approach is to reduce the risk of iatrogenic infection secondary to operation on a contaminated wound. A longer delay will also cause difficulties in doing primary repair due to scar formation and retraction of nerve endings, but most importantly, injured parts of the nerve ends should be resected to obtain viable nerve ends for optimal coaptation.
Surgical approach
The incisions follow the general principles of plastic surgery in that the main objective is to prevent contractures and scars in areas of maximum sensory perception (Fig. 32.11). Wide exposure is often recommended to obtain a detailed anatomical overview, and it offers a safer dissection as the area of injury can be approached from healthy tissue outside the zone of injury. This often means that the traumatic wound needs to be extending proximally and distally, which is particularly important in secondary reconstructions.
Principles of nerve repair
General principles
Firstly, the injured nerve ends must be identified. Nonviable tissue must be resected, preferably with a surgical blade or using microsurgical scissors, to allow visualization of healthy fascicles that help the adaptation in primary nerve repair (Figs 32.12–32.14). When repairing a nerve it is preferable to align the nerve according to its fascicular pattern, which may help topographical arrangement and improve outcome. If later reconstruction is planned (secondary reconstruction), the nerve ends may be tagged with a nonresorbable suture, and sutured to other structures in the wound. This is primarily to prevent the retraction of the nerve that inevitably happens after transection, but also to facilitate identification at a secondary procedure. If the nerve is allowed to retract, it will become tethered in fibrous scar tissue and lose its elasticity and normal excursion. The normal longitudinal excursion of the nerve needs to be taken into consideration, as the immediate demand for suitable length will depend on it. The normal excursion of the median and ulnar nerves is thought to be around 1.5 cm at the wrist when taking the wrist from full extension to full flexion.36,37 This length should preferably be added to the nerve deficit when estimating the total defect and assessing whether a nerve graft is needed or not. In a primary repair, the longitudinal extension is retained and the nerve can therefore be approximated with minimal tension.
Secondly, the ultimate aim is a tension-free repair to enable the nerve to heal in optimal conditions. Usually, some dissection of the nerve trunk is necessary to achieve this. Even a small elongation through longitudinal traction will have a potentially devastating effect on the vascular supply to the nerve endings, as stretching of a nerve trunk has been shown to impair intraneural microcirculation.38 Nerve blood flow decreases approximately 50%, with substantial recovery in 30 minutes after 8% elongation, whereas 15% elongation produced approximately an 80% reduction in blood flow with minimal recovery.29 Some dissection of the nerve trunks proximal and distal to the injury is therefore often needed to alleviate tension, and this can be done without interfering with the segmentally approaching blood vessels. Other means of alleviating tension, such as transposing the ulnar nerve anteriorly, and therefore increasing excursion over the elbow, can be considered to enable a tension-free direct suture. It is, however, important to bear in mind that mobilization by dissection can theoretically impair the blood supply to the nerve endings. This issue has been an issue of debate,39–41 although clinical experience from ulnar nerve transposition points to the fact that intraneural microcirculation can resist considerable mobilization, and that gentle handling of the nerve can be acceptable.40,41
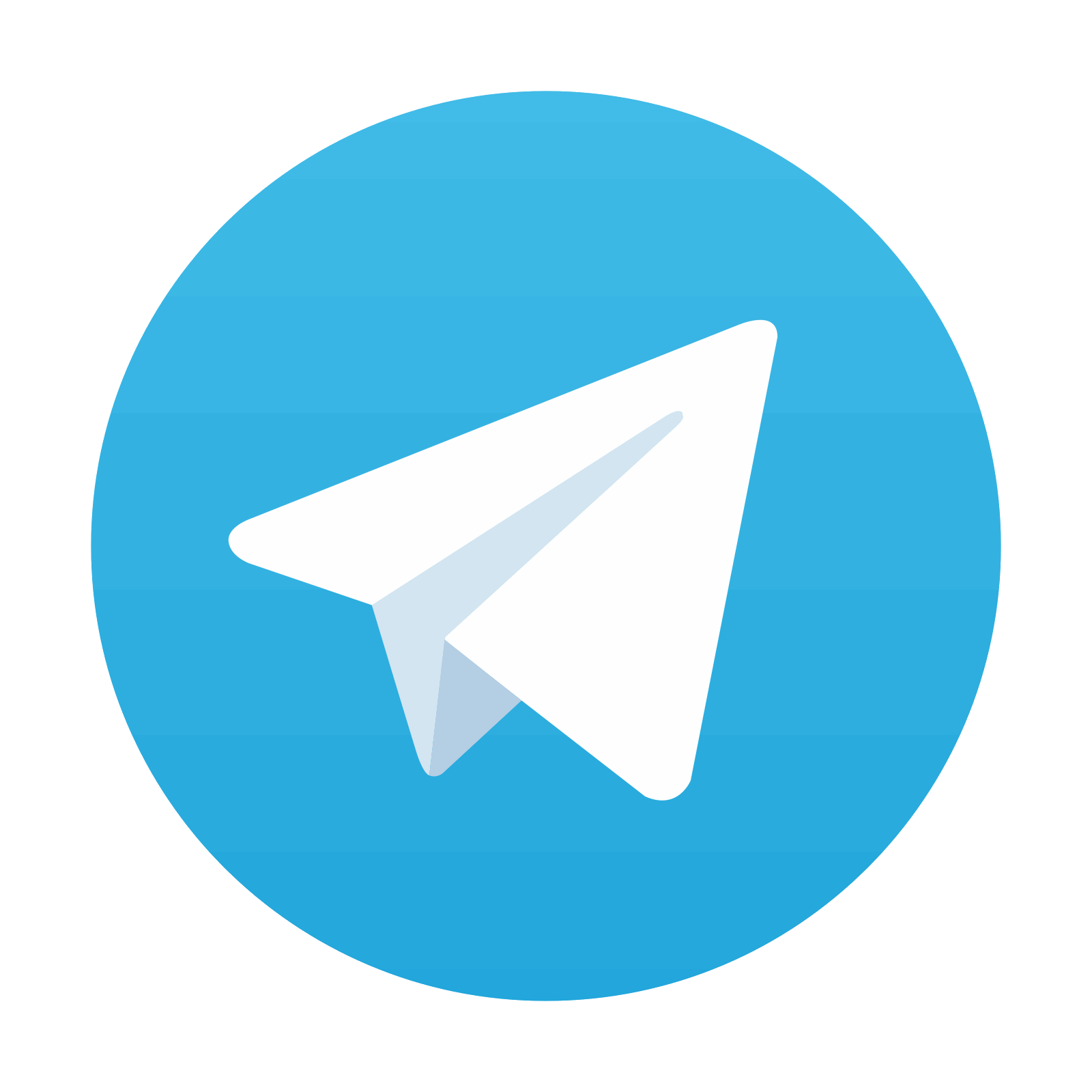
Stay updated, free articles. Join our Telegram channel

Full access? Get Clinical Tree
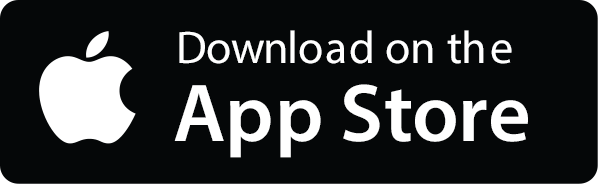
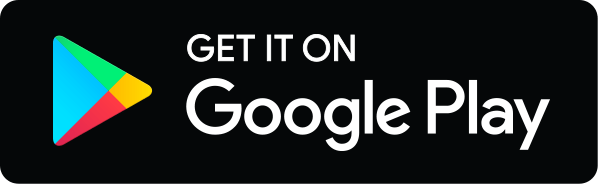