, Tea Soon Park1, 2, Vera S. Donnenberg3, 4, 5 , Elias T. Zambidis1, 2 and Albert D. Donnenberg6, 4, 5, 7, 8
(1)
Division of Pediatric Oncology, Johns Hopkins University, Department of Oncology, Sidney Kimmel Comprehensive Cancer Center at Johns Hopkins, 733 N. Broadway, MRB 772, Baltimore, MD 21205, USA
(2)
Institute for Cell Engineering, Johns Hopkins School of Medicine, Baltimore, MD, USA
(3)
Department of Cardiothoracic Surgery, University of Pittsburgh School of Medicine, Research Pavilion 2.42b, 5117 Centre Avenue, Pittsburgh, PA 15213, USA
(4)
University of Pittsburgh Cancer Institute, Hillman Cancer Center, Pittsburgh, PA, USA
(5)
McGowan Institute for Regenerative Medicine, Pittsburgh, PA, USA
(6)
Division of Hematology/Oncology, Department of Medicine, University of Pittsburgh School of Medicine, Research Pavilion 2.42c, 5117 Centre Avenue, Pittsburgh, PA 15213, USA
(7)
Hematopoietic Stem Cell Laboratory, University of Pittsburgh Medical Center, Pittsburgh, PA, USA
(8)
University of Pittsburgh Cancer Institute Cytometry Facility, Pittsburgh, PA, USA
9.1 Introduction
The perivascular niche acts as a reservoir of stem/progenitor cells that are readily available for cellular therapies. Bone marrow- and adipose-derived mesenchymal progenitor/stem cells possess great promise for regenerative medicine [1, 2], immunomodulation [2, 3], and cancer-targeting therapies [4]. Multipotent mesenchymal stromal cells (MSC) were originally derived from the bone marrow (BM) and defined in vitro as a plastic-adherent fibroblastic cell population, exhibiting a defined, uniform immunophenotype (e.g., expression of CD73, CD90, CD105, and lack of expression of hematopoietic/endothelial markers) and capable of clonal differentiation toward mesenchymal lineages (e.g., adipogenic, osteogenic, and chondrogenic lineages) [5, 6]. The in vivo precursors of in vitro-expanded BM-MSC have been identified as subendothelial reticular cells [7], a population of adventitial cells closely surrounding the BM sinusoids and the medullary equivalent of the ubiquitous pericyte.
Pericytes have been isolated from a variety of human fetal and adult tissues and have been shown to be a source of MSC [8, 9]. The scarcity of these MSC-generating cells in the BM [10] requires their in vitro expansion prior to use for most subsequent applications. Alternatively, other in vivo sources, such as adipose tissue, display a much higher content of mesenchymal progenitors [11, 12]. Fat is now recognized as an abundant source of multiple mesodermal progenitor/stem cells. These include endothelial progenitors and several mesenchymal progenitors, such as pericytes and supra-adventitial stromal cells (SA-ASC), a second layer of MSC-like cells that surround small blood vessels in an annular fashion [12]. Adipose tissue was originally used in regenerative therapy for soft tissue reconstruction over a century ago [13, 14]. However, the use of autologous fat transfer was hampered for decades due to its limited long-term volume retention and poor vascularization [15]. Cell-assisted lipotransfer [16–18] circumvents these issues by the addition of a mixture of stem/progenitor cells, roughly the whole concentrated adipose stromal vascular fraction (SVF), to the lipoaspirate material.
The emergence of autologous fat transplantation in the early 1890s by Neuber [13] and Czerny [14] was shortly preceded by the discovery of pericytes in the 1870s. While pericytes were first observed by Eberth in 1871 [19], they were formally described by Rouget in 1873 [20] as a contractile cell population surrounding microvessels. Concurrently in 1867, Cohnheim suggested that the bone marrow was a reservoir of non-hematopoietic stem cells [21], despite the fact that the term “stem cell” was only coined a year later by Haeckel [22]. Although the microvasculature wall is now recognized as a source of mesenchymal progenitors [23], the heterogeneity of the adventitia in high turnover tissues such as adipose [24] poses a challenge to the identification of individual stem/progenitor populations. Our recent work [25] supported both pericytic heterogeneity in human fat and the existence of a developmental hierarchy. This pericytic continuum includes a relatively quiescent stem cell-like population expressing MSC markers in vivo, as well as a transit-amplifying CD34+ subset which may give rise to the SA-ASC, the most prevalent and homogeneous mesenchymal progenitors in adipose tissue [12].
Although the developmental equivalence of pericytic stem/progenitor cells across disparate lineages has not yet been clearly elucidated, pericytes from various tissues partially share a common immunophenotype and differentiation potential. In most organs, pericytes contain heterogeneous populations that specialized to promote vascular contractility and stability, as well as a subpopulation of mesenchymal stem/progenitors, although these two subsets may overlap. Moreover, while pericytes were primarily defined as a component of the microvascular wall, their existence is now also accepted in larger vessels [26, 27]. However, the universality of pericytes and pericytic stem cell subsets throughout the small and large branches of the vascular tree (Fig. 9.1) is still subject of debate [23].
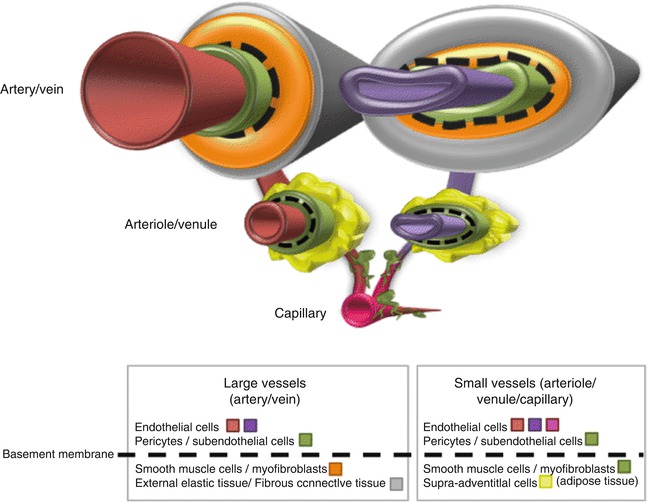
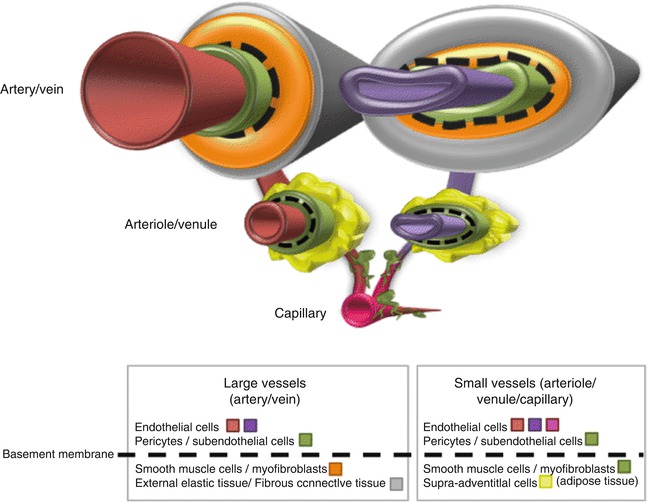
Fig. 9.1
Annular organization of the tunica throughout the vasculature tree. Luminal endothelial cells (red, pink, purple) populate the intima of small and large vessels and are enveloped by a subendothelial layer of pericytes (green). Both pericytes and endothelial cells are localized within the basement membrane, though the basal lamina continuity can be remodeled in the course of dynamic angiogenic events (e.g., during development or tumorigenesis) [27, 31, 32]. In small vessels, pericytes may be associated with a thin layer of other closely related vasoregulator cells, such as myofibroblasts. Supra-adventitial adipose stromal cells (SA-ASC, yellow) are a distinct feature of adipose tissue and loosely surround pericytes in an annular fashion [12]. SA-ASCs represent an alternate source of multipotent mesenchymal progenitors from pericytes [12, 75, 78]. Large vessels possess a specialized outer ring of smooth muscle cells/myofibroblasts (orange) and are surrounded by external elastic and connective tissue (grey). In large vessels, pericytes may also be associated with the vasa vasorum, a network of small blood vessels that branch off and supply large blood vessels. Although the phenotype of pericytes may vary among small and large vessels, multipotent perivascular progenitors have been successfully isolated from pre- and post-capillaries in the microvasculature [8], as well as arteries and veins [116–118]. The equivalence of all mesenchymogenic adventitial cells is still controversial
9.2 Toward a Working Definition of the Pericyte
Pericytes have been extensively reviewed in the literature by both neurovascular and stem cell biologists [26–28] and yet, their definition remains confused and challenging. Pericytes have been anatomically defined by their periendothelial location in the vascular wall [29] and their embedding within the basement membrane [30]. While their anatomical location respective to the endothelium is not sufficient to differentiate pericytes from other perivascular cell types such as smooth muscle cells and myofibroblasts, the more stringent criterion of inclusion within the basal lamina can only be established by electron microscopy. Furthermore, the basement membrane is actively remodeled by both pericytes and endothelial cells [31] during the active angiogenesis of development [27] and tumorigenesis [32]. During this time, pericytes may temporarily rearrange their contacts with both endothelial cells (e.g., peg and socket arrangements, adherent junctions, and gap junctions), as well as the basal membrane (e.g., adhesion plaques) [27]. While pericytes have been mostly identified in the microvasculature (e.g., capillaries, venules, and arterioles), they have also been described in subendothelial layer of larger veins and arteries (Fig. 9.1) [33, 34] that supply the vasa vasorum [35].
To circumvent the ambiguity of such location-based descriptions, the definition of pericytes has been further refined with phenotypic (e.g., morphology, gene/protein expression) or functional (e.g., vascular stability/contractility and degree of stemness) criteria. Pericytes can be identified in microcapillaries by their round-shaped nucleus that contrasts to the elongated nuclei of endothelial cells [28]. However, such morphological peculiarities may be obscured in postcapillary blood vessels, or among various vessel types [27] and distinct tissues. For instance, while brain pericytes exhibit a large size with multiple cytoplasmic expansions, kidney pericytes are compact with minimal abluminal surface [36]. Most importantly, although multiple markers have been proposed to identify pericytes, the nonspecific and nonuniform expression of these markers throughout the whole pericyte diversity confounds their identification.
9.3 Putative Markers that Identify Pericytes
The main limitation of currently acknowledged pericytic markers is their inability to differentiate pericytes from their lineage-related neighbors: myofibroblasts and smooth muscle cells. In fact, there is currently not a universal pan-pericytic marker that can specifically identify pericytes in all human tissues. Thus, most strategies to isolate pericytes should rely on employing a combination of markers. This prerequisite has become increasingly evident from several recent publications. For instance, the ganglioside marker 3G5 can identify pericytes in human infrapatellar fat pads [37], but is not in dermal tissue [38], where 3G5 expression specifies mast cells. 3G5 is also expressed by pancreatic lineages and melanocytes [39]. Furthermore, although 3G5 is expressed by dental pulp-derived MSC-generating cells, its expression is restricted in BM-derived MSC progenitors [40], thus limiting its value to identify mesenchymogenic pericytes. The regulator of G protein signaling 5 (RGS-5) is an early marker of pericytic development but its expression has been reported to be absent on normal brain pericytes [39].
A variety of pericyte markers were previously reviewed extensively [39, 41]. Thus, we will focus here only on the main markers that are commonly used to identify pericytes and in particular those that can generate MSC. Investigators should bear in mind that the specificity of each marker can vary, not only as a function of the tissue, but also as a dynamic feature during development.
9.3.1 Alpha Smooth Muscle Actin (αSMA) and Desmin Expression
αSMA expression was initially thought to uniquely identify smooth muscle cells, but its expression can also be detected in mesodermal cell types, including myofibroblasts and pericytes [42]. Furthermore, αSMA is not expressed uniformly throughout the vascular wall. While smooth muscle cells regulate blood flow in larger vessels, pericytes can similarly control vasoconstriction and vasodilatation of the microvasculature. This function is revealed by the pericytic expression of the contractile proteins αSMA, desmin, tropomyosin, and myosin [41]. Although αSMA is an intracellular marker, several studies have relied on its expression to identify and isolate mesenchymogenic pericytes with gene-specific reporters [43, 44]. Although capillaries are mostly devoid of αSMA expression [28], postcapillary expression of αSMA in pericytes is consistently observed in most tissues (although expression in the brain is restricted to arterioles). However, pericytes can upregulate αSMA expression to adjust capillary blood flow in response to the acute stress of vascular remodeling [45]. In highly vascularized tissues, such as the brain, only 5 % of freshly isolated capillary pericytes express αSMA [28], although expression can be acquired in vitro following TGFβ stimulation [46].
Desmin expression is present in the majority of pericytes populating the microvasculature [45], as well as in the cardiomyogenic and myogenic lineages [47]. However, desmin expression is absent from subendothelial pericytes that populate large vessels such as the saphenous vein [34], thus emphasizing the general disparities of contractile protein expression by various pericytes throughout the vascular tree.
9.3.2 Neuron-Glial 2 (NG2) and Nestin Expression
In the brain, pericytes express two markers of neurogenic progenitors: nestin and the chondroitin sulfate proteoglycan NG2 (also known as high molecular weight melanoma-associated antigen) [48]. NG2 expression has been reported in capillaries and arterioles, but not in venules of mesenteric, subcutaneous, and muscular tissue [49]. NG2 expression is also modulated during active vasculogenic, angiogenic, and tumorigenic events [50]. Feng et al. recently reported the nonexclusive contribution of NG2+ pericytes to the pool of MSC during tooth development using an inducible NG2 reporter mouse model [51]. Furthermore, nestin has been recognized as a pericyte marker, mainly in the nervous system, but also in other tissues like the pancreas, though nestin preferentially marks pancreatic endothelial cells [52].
9.3.3 Expression of Platelet-Derived Growth Factor Receptors (PDGFR) α and β
Although PDGFRβ is essential to endothelial-pericyte crosstalk and pericyte expansion during development [53], expression of the platelet-derived growth factor receptors α and β (also known as CD140a and CD140b) can be retained by adult pericytes [39, 54]. PDGFRβ- and PDGFβ-null mutations are lethal and result in severe pericyte deficiency, leading to microvascular leakage and dysfunction [55, 56]. PDGFRβ signaling is particularly important for kidney pericytes (also known as mesangial cells) for development of the glomeruli [57]. PDGFRβ+ brain pericytes have been shown to co-express few MSC markers natively, but nonetheless have been proposed to be a source of mesenchymal stem cells [58]. Additionally, circulating blood-derived and endometrial PDGFRβ+ cells were recently shown to be a source of mesenchymal progenitors [59, 60]. PDGFRα also marks the mature neuronal lineage [61], is broadly expressed in the mesenchyme, and is required for the development of nonneuronal neural crest derivatives in the cranial and cardiac regions [62]. Furthermore, PDGFRα signaling may be critical for the survival and migration of neural crest derivatives [62] including neural crest-derived pericytes that populate the cranial region [63]. For example, PDGFRα + pericytes have been shown to actively participate in the sealing of spinal cord injuries [54].
9.3.4 Expression of CD146 and CD106
The melanoma cell adhesion molecule (Mel-CAM or MCAM, also known as MUC18 and CD146) and the vascular cell adhesion molecule-1 (VCAM-1, also known as CD106) are expressed by pericytes, endothelial cells [64, 65], as well as few other lineages. In murine models, CD106 expression has been used to positively enrich MSC-generating cells within the STRO-1+ bone marrow populations [66], whereas CD146 has been extensively used to identify pericytes from various fetal and adult tissues across mammalian species [7–9]. Depending on the tissue, CD146 expression should be combined with other markers to eliminate endothelial and hematopoietic subsets.
9.3.5 Expression of MSC Markers and the Mucosialin CD34
The co-expression of MSC markers by CD146+ pericytes has been previously documented following brief in vitro culture of human adipose, bone marrow, pancreas, placenta, and skeletal muscle tissues [8, 9, 67]. However, BM-MSC can also express pericyte markers (CD140b, CD146) after short in vitro expansion [68]. Crisan et al. were able to detect native expression of single MSC markers (CD44, CD73, CD90, CD105) by both immunofluorescence and flow cytometry in CD146 + CD34-CD56-CD45- pericytes [8] from human adipose, placenta, and skeletal muscle tissues. However, these studies did not clearly establish any discrete MSC-like pericyte population, since some of these markers (e.g., CD44 and CD90) were expressed by all pericytes, while others (e.g., CD73, CD105) were restricted to smaller subpopulations. The elimination of CD34+ cells from the pool of pericytes in these studies may also be too stringent to define pericytes in a tissue like the adipose, because CD34 expression is an early marker that identifies perivascular fat precursors in the mouse adipose tissue [69, 70].
The stromal vascular fraction (SVF) of adipose tissue displays a high content of multipotent mesenchymal progenitor populations [12] that are organized closely around microvessels in an annular fashion (Fig. 9.2). This mixture of adipose progenitors was prospectively isolated by adherence in 1976 by two independent groups [71, 72] and was later characterized as multipotent adipose-derived stromal cells (ASC) [73], the fat equivalent of BM-MSC. The definition of ASC and BM-MSC shares both their mode of selection (adherence) and their multipotentiality toward mesenchymal lineages. Yet, ASC only come to resemble BM-MSC in vitro after several passages, when their immunophenotype stabilizes [74]. The initial phenotypic discrepancies (mainly the expression of the mucosialin CD34 in ASC) are likely due to the multiple populations giving rise to ASC as compared to the probably unique subendothelial origin of BM-MSC. In human fat, both pericytes and the outer supra-adventitial layer of CD34+ cells (termed supra-adventitial stromal cells or SA-ASC) possess high adipogenic potential in vitro [12, 75] and may contribute together to constantly replenish the pool of adipocytes in vivo. As also reported by others [76, 77], we detected a subpopulation of CD34+ adipose pericytes [10, 12, 23], unique to our knowledge to adipose/subcutaneous tissues, that we hypothesized to be a developmental intermediate between pericytes and SA-ASC. While the presence of CD34 + CD146- SA-ASC-like cells has also been detected in a recent study in fetal muscle, lung, and bone marrow [78], the failure to exclude CD31+ endothelial cells does not preclude the possibility that the authors isolated a CD146- subpopulation of CD34 + CD31+ endothelial progenitors, which may also possess some limited mesenchymal potential according to our results [12]. This study also confirmed that elusive CD34+ pericytes cannot be easily detected within the vascular wall by immunofluorescence and require a more stringent rare-event strategy for their detection and isolation by flow cytometry [10]. Despite these challenges, the existence of rare CD34 + CD146 + CD31- pericytes has been independently confirmed [79]. Using high throughput multiparametric flow cytometry, we recently detected simultaneously the expression of three MSC markers (CD73, CD90, and CD105) in human adipose pericytes (CD146 + CD31-CD45- adipose SVF cells) and SA-ASC (CD34 + CD146-CD31-CD45- adipose SVF cells) [25]. This analysis allowed us to decipher distinct subpopulations (Fig. 9.3) among non-endothelial, non-hematopoietic CD146 + CD31-lineage-CD45- pericytes. Only a third of adipose pericytes co-expressed in situ all three MSC markers CD73, CD90, and CD105. Among these MSC-like pericytes, two populations were discerned on the basis of CD34 expression: a low proliferative CD34- population and a highly proliferative CD34+ subset. While the first phenotype may be typical of multipotent mesenchymal pericytes in adipose and most other tissues, the latter may represent a transit-amplifying intermediate between stem-like adipose pericytes and the low proliferative highly prevalent adipogenic SA-ASC. Human preadipocytes are considered to belong predominantly to the CD34 + CD31- compartment of the adipose SVF [80], which bridges the phenotypes of SA-ASC and rare CD34+ pericytes and is also the accepted ASC phenotype after initial plating [81] and prior to extensive expansion. It is interesting that CD34+ SA-ASC can generate robust multipotent mesenchymal progenitors in vitro [75, 78, 82] and revert in vitro to a pericytic phenotype [78, 82], which may indicate a high degree of plasticity or the persistence in vitro of a rare CD34+ mesenchymogenic population, which has also been isolated from the fetal [83, 84] and adult [85, 86] bone marrow.
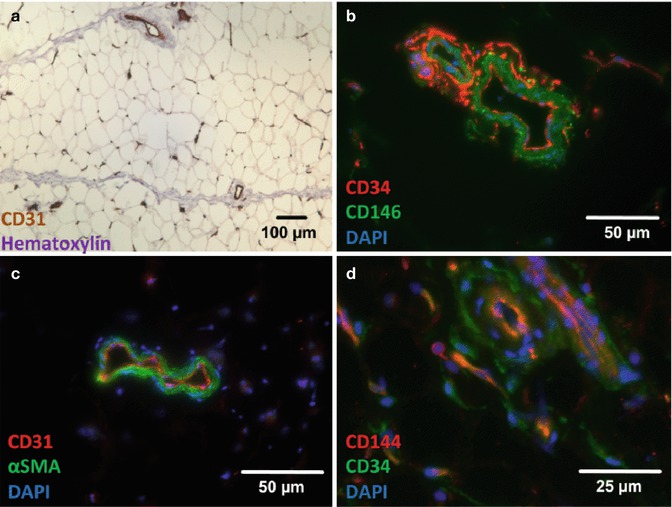
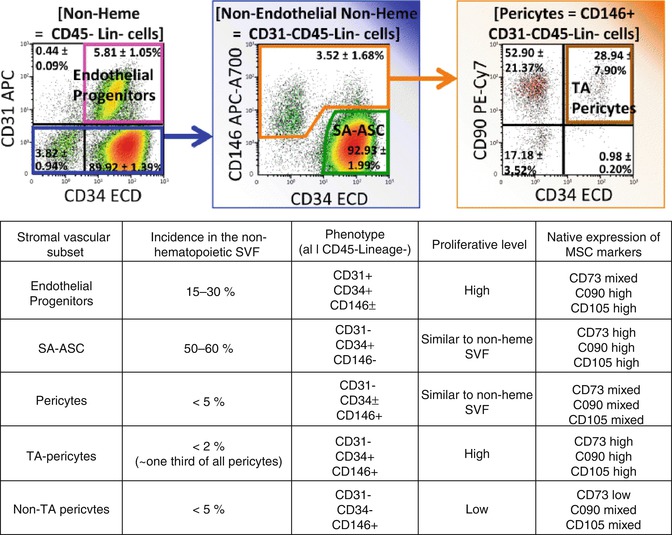
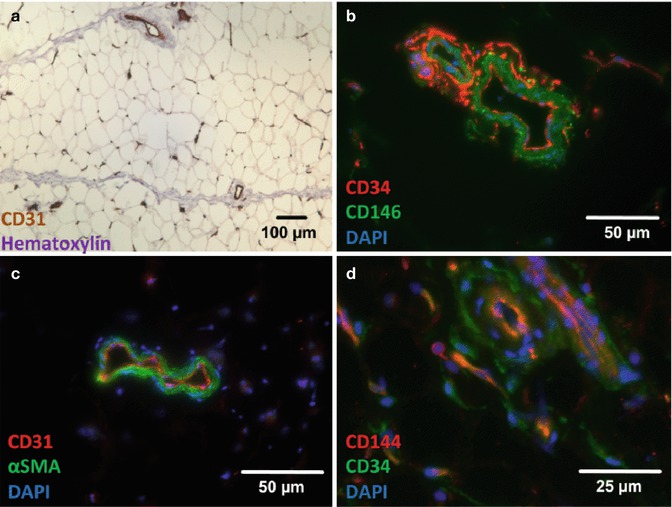
Fig. 9.2
Photomicrographs of immunostains of human fat microvasculature. (a) Immunohistochemical staining of CD31 (DAB, brown) at low magnification (10× objective). Human fat is a highly microvascularized tissue showing numerous CD31+ capillaries and small vessels. (b–d) Immunofluorescent stainings of CD31 (c, red), CD34 (b, red; c, green), CD144 (d, red), CD146 (b, green), and α-SMA (c, green) of human fat microsections. Although CD31 (a, c) and CD144 (VE-cadherin, d) specifically mark intimal endothelial cells in capillaries, arterioles, and venules, CD34 is expressed by a distal population of mesenchymal progenitors, termed SA-ASC (b, d). Scattered SA-ASC encircle pericytes, positively marked by CD146 (b) and αSMA (c) expression. Note that CD146 antibodies can also stain endothelial cells, and capillaries are mostly negative for αSMA staining. As the expression of CD34 by a subset of adipose pericytes is probably a dynamic and transitory process, its detection remains challenging by immunohistostaining. This issue can be resolved using high throughput techniques, such as flow cytometry [10, 12], although some elusive CD34+ periendothelial cells can rarely be detected in tissue microsections (d). Detailed staining procedures are provided in [12]
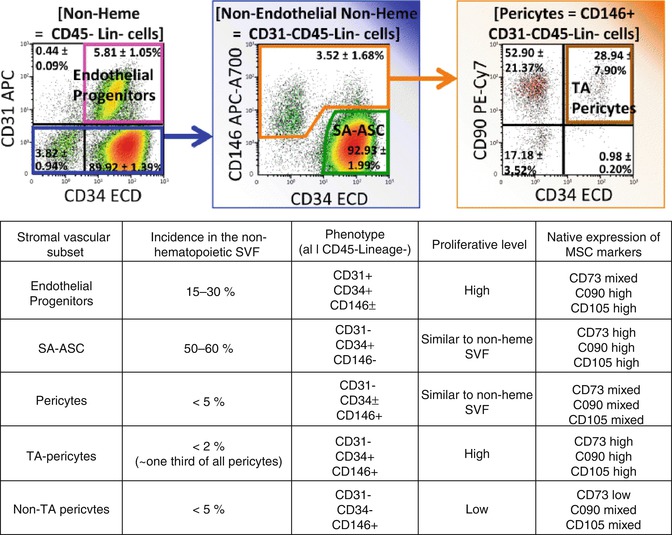
Fig. 9.3
Flow cytometric analysis of 10 independent adipose stromal vascular fraction samples. Among CD45- (Lin- selects CD14-CD33-CD235a- cells) non-hematopoietic cells, three progenitor populations can be detected: endothelial progenitors (pink), pericytes (orange), and SA-ASC (green). Pericytes and SA-ASC are two sources of mesenchymal progenitors in human fat [23]. Pericytes can be subdivided in individual fractions based on the expression of CD34 [10, 12, 23, 25, 79]. CD34+ pericytes are a rare (less than 2 % of nonheme stromal vascular cells) highly proliferative population expressing natively the MSC markers CD73, CD90, and CD105 [25]. We have speculated that the CD34+ pericytes represent a transit-amplifying intermediate between rare quiescent pericytes and prevalent adipogenic SA-ASC [23, 25]. The table percentages and expression/proliferation levels summarize data are presented in [12] and [25]
9.4 Developmental Origins of Pericytes
During early development, pericytes are considered to be derivatives of mesenchymal precursors which are recruited during nascent angiogenesis to self-assemble around preformed endothelial tubes [87, 88]. Most mesenchymal tissues, including differentiated lineages [89, 90], but also mesenchymal stem cells [91, 92] and pericytes [26], are believed to originate from both the neural crest and the mesoderm (Fig. 9.4). In the head and the thymus, pericytes derive mostly from the neural crest [26]. In epithelial tissues, including the gut, the lung, and the liver, pericytes, smooth muscle cells, and myofibroblasts are believed to derive by epithelial-to-mesenchymal transition (EMT) from the mesothelia [26]. Similarly, the CD34+ epicardial mesothelium [93–96] has been suggested to supply cardiac pericytes. However, the exclusivity of the mesothelial origin of pericytes in these tissues has not been clearly elucidated. In fact, in most organs, mesenchymal lineages and pericytes may arise from multiple origins.
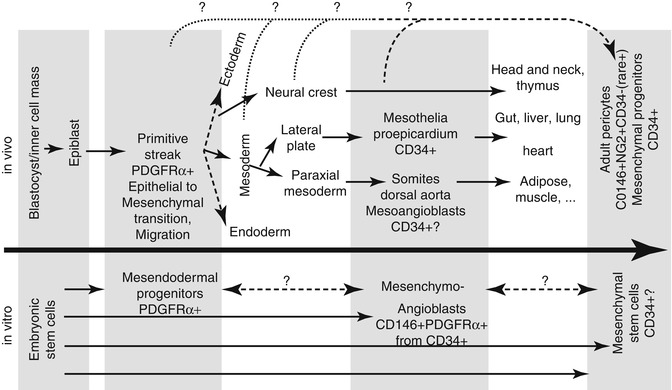
Get Clinical Tree app for offline access
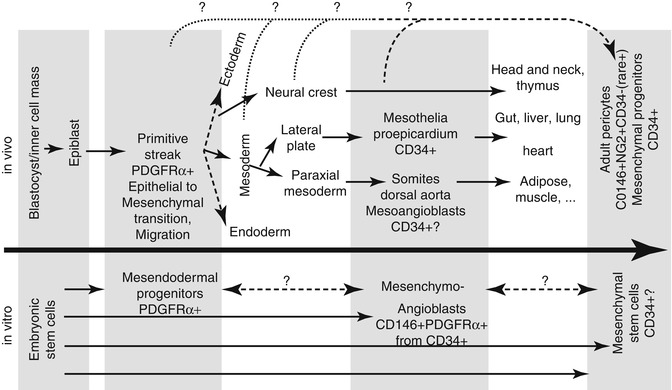
Fig. 9.4
Developmental origins of mesenchymogenic pericytes. Adult pericytes have been shown to be a source of mesenchymal stem cells [8]. As all mesenchymal lineage populations, pericytes can be derived from either the neural crest (head and neck, thymus) or the mesoderm [26]. Heart and epithelial tissue-resident pericytes derive mostly from mesothelia (gut, liver, lung) or CD34+ pericardial cells [26, 93–95]. In the mouse, mesoangioblasts have been shown to be CD34+ multipotent cells [97, 98] populating the dorsal aorta, which may represent ancestors of pericytes populating paraxial mesoderm derivatives like the adipose and muscular tissues. Human embryonic stem cells have been used as a model to generate a multipotent precursor of the mesenchymal and endothelial lineages, the mesenchymoangioblast [104]. The dual neural crest/mesoderm origin of pericytes hinders a clear elucidation of their ontogeny. It remains unclear if multipotent pericytes derived from the neural crest and mesoderm represent two distinct entities or if pericytes become specialized during the development or, alternatively, if they escaped early lineage specification. Pluripotent progenitors resembling the primitive streak can be derived from human embryonic stem cells and possess meso-endodermal differentiation potential, including elevated endothelial, smooth muscle, skeletal muscle, and cardiac potentials [105]. The primitive streak is a highly dynamic tissue, characterized by massive migratory and phenotypic changes. During gastrulation, epiblast cells undergo an epithelial-to-mesenchymal transition, which will generate the mesoderm and participate to the neural crest. While human primitive CD34+ MSC and multipotent pericytes have been successfully derived from human pluripotent stem cells [106, 107
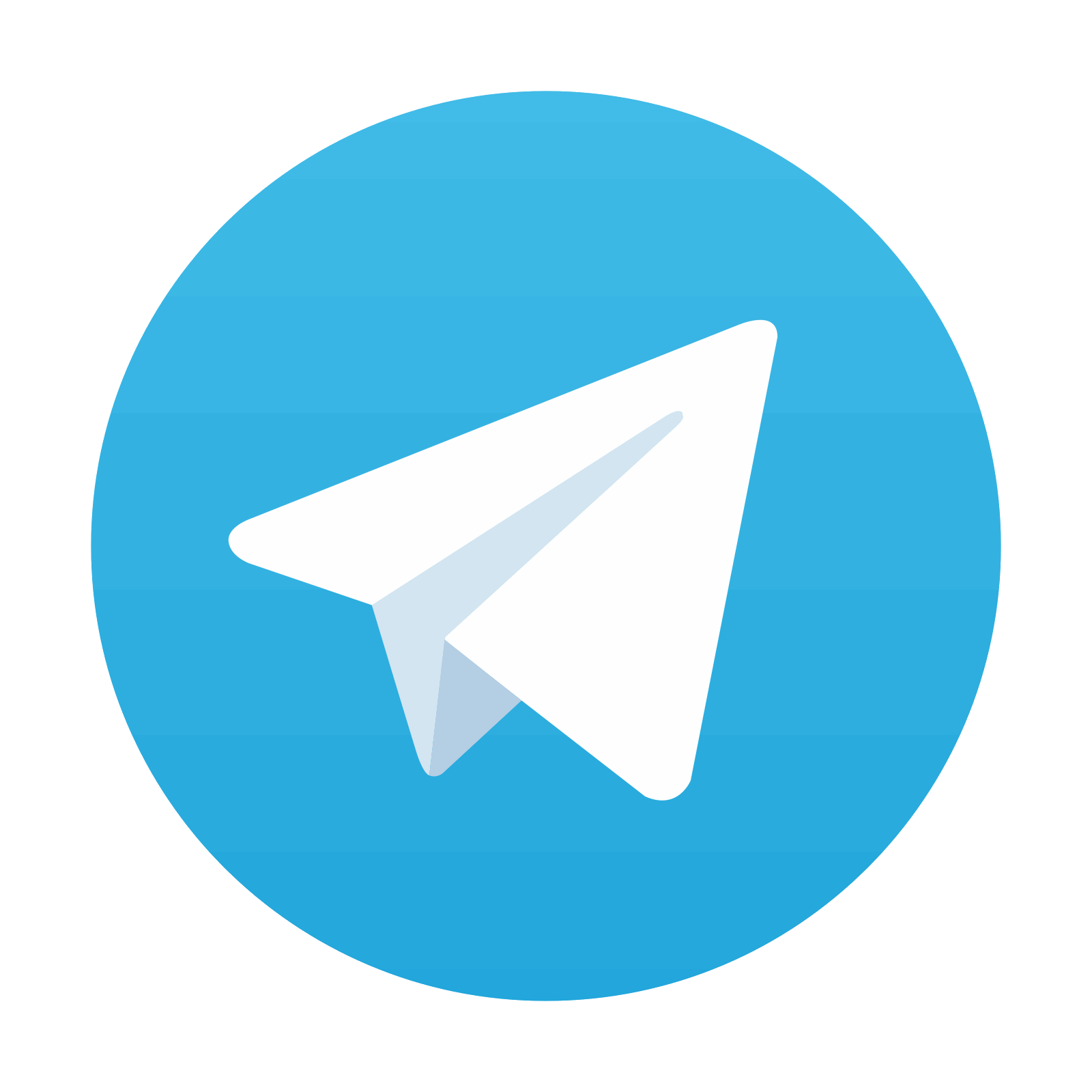
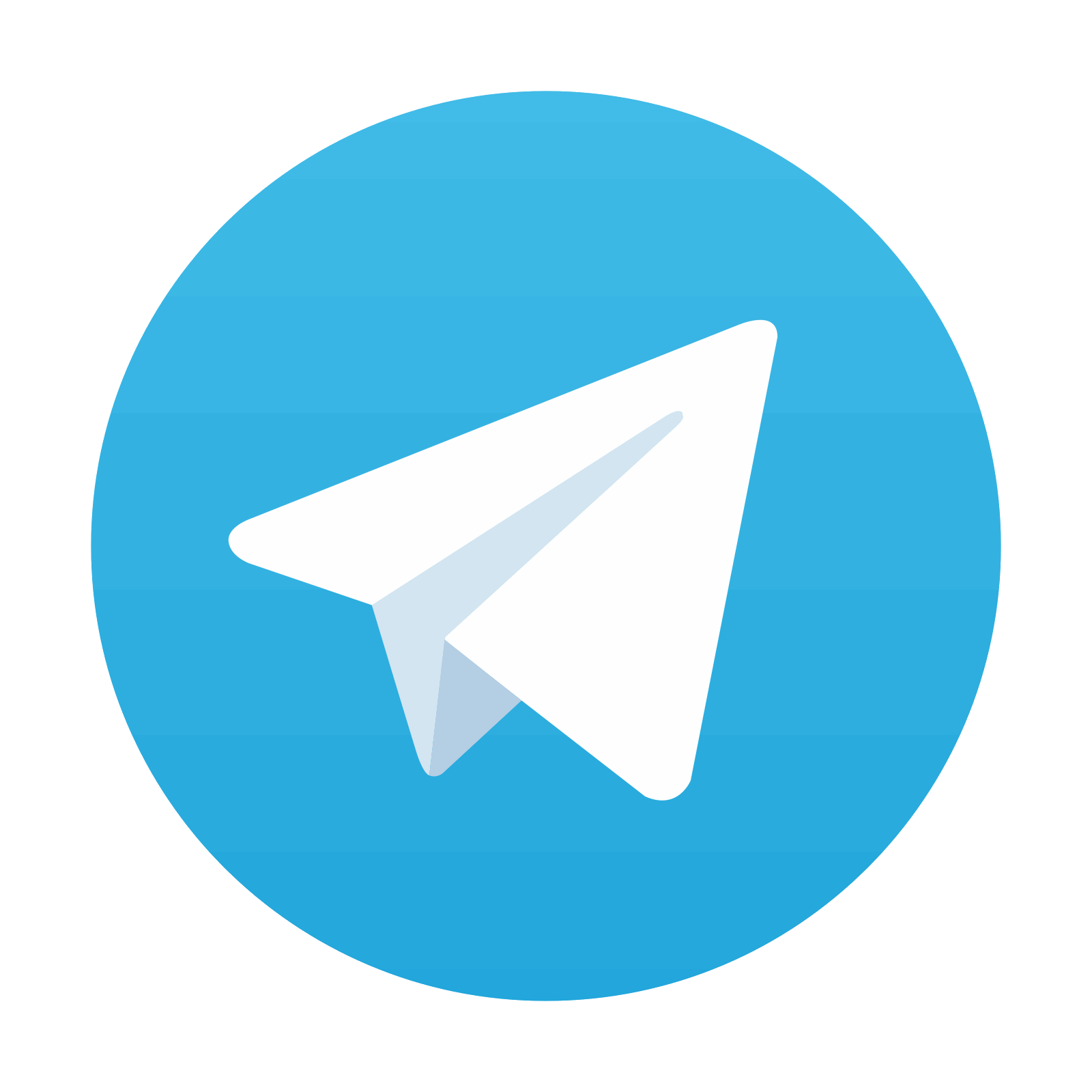
Stay updated, free articles. Join our Telegram channel

Full access? Get Clinical Tree
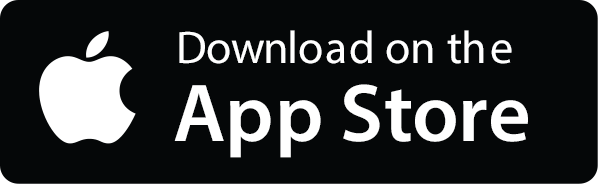
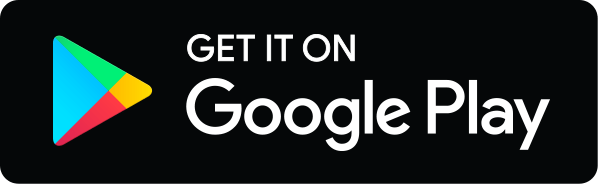
