Fig. 1.1
Increasing numbers of patients are undergoing allogeneic hematopoietic stem cell transplantation (HSCT) every year (Adapted from Pasquini and Zhu [1]; with permission)
Historical Perspective
Pioneering experimentation by Jacobson in the 1940s showed that mice were protected from the deleterious effects of radiation if their spleens were shielded with lead foils [2]. The cellular basis of this protection was proved when infusion from marrow and spleen cells conferred a similar protective effect from radiation. Barnes et al. [3] were the first investigators to report treatment of murine leukemia with high-dose radiation followed by infusion of healthy marrow from littermates. The first group to publish results of long-term survival of patients with acute leukemia was from the Seattle group led by Dr. E.D. Thomas [4]. Patients received total body irradiation (TBI) and cyclophosphamide (Cy)–based conditioning and bone marrow grafts from HLA-matched sibling donors. Only 13 of the initial 100 enrolled patients in this trial eventually survived on long-term follow up, but this initial study gave an indication that this form of therapy could be curative in a proportion of leukemia patients. Similar results published by Blume and Beutler from City of Hope [5] confirmed the initial results from Seattle. Another advance in the field was the introduction of calcineurin inhibitors in combination with methotrexate for prevention of GVHD [6, 7]. This combination was associated with significantly lower risk of Grades II–IV aGVHD and improved disease-free survival. Other advancements in the field have been the development of alternative conditioning regimens to TBI/Cy [8], the introduction of prophylactic ganciclovir for cytomegalovirus (CMV) pulmonary infection [9], and the introduction of allele-level HLA typing as a result of improvement in molecular typing techniques, resulting in better-matched transplants [10]. Introduction of RIC regimens has allowed elderly patients and patients with comorbidities who cannot tolerate fully myeloablative regimens to undergo HSCT from matched sibling and unrelated donors, thus expanding access to this curative modality for a wider patient population [11, 12].
Indications for Allogeneic Hematopoietic Cell Transplantation
Allogeneic HSCT is often the only potentially curative treatment for hematologic malignancies in an advanced stage or for relapsed disease. For early-stage disease such as acute myeloid leukemia (AML) or acute lymphoblastic leukemia (ALL) in first complete remission (CR1), the risk of relapse can be significantly reduced by allogeneic HSCT, but the medical decision is complex and has to be carefully balanced against the risk of transplant-related mortality. In general, allogeneic HSCT is considered for acute leukemia in CR1 with intermediate-risk or high-risk features, or cases that are beyond CR1. In the United States, AML/ALL and myelodysplastic syndromes (MDS) account for 65 % of patients who undergo allogeneic HSCT based on the most recent data provided by the Center for International Blood and Marrow Transplant Research (CIBMTR) [13]. With the introduction of targeted therapy with tyrosine kinase inhibitors for chronic myeloid leukemia (CML), HSCT is reserved only for patients with refractory disease. Refractory non-Hodgkin’s lymphoma, chronic lymphocytic leukemia (CLL), and nonmalignant diseases such as aplastic anemia, and paroxysmal nocturnal hemoglobinuria (PNH) comprise the remainder of indications for allogeneic HSCT.
The American Society of Blood and Marrow Transplantation (ASBMT) established a multiple-stakeholder taskforce to study role of HSCT in established disease states and identify emerging indications where HSCT may potentially be beneficial. This task force came out with a white paper in 2015 with clearly defined indications across disease states where HSCT has been shown to be of clinical benefit based on available clinical trial data. Published systematic evidence reviews or guidelines were used as the basis for recommendations to categorize indications for HSCT in pediatric and adult populations [13]; this comprehensive review of indications for HSCT based on currently available clinical evidence is highly recommended (Table 1.1) (Fig. 1.2) [14].

Table 1.1
Diseases for which allogeneic and autologous hematopoietic stem cell transplantation is used [13]
Malignant |
Leukemia/preleukemia |
Chronic myeloid leukemia |
Myeloproliferative syndromes (other than chronic myeloid leukemia) |
Acute myeloid leukemia |
Acute lymphoblastic leukemia |
Juvenile chronic myeloid leukemia |
Myelodysplastic syndromes |
Therapy-related myelodysplasia/leukemia |
Kostmann’s agranulocytosis |
Chronic lymphocytic leukemia |
Non-Hodgkin’s and Hodgkin’s lymphoma |
Multiple myeloma |
Solid tumors |
Breast cancer |
Neuroblastoma |
Sarcomas |
Ovarian cancer |
Small cell lung cancer |
Testicular cancer |
Nonmalignant |
Severe aplastic anemia |
Paroxysmal nocturnal hemoglobinuria |
Hemoglobinopathies |
Thalassemia major |
Sickle cell disease |
Congenital disorders of hematopoiesis |
Fanconi anemia |
Diamond-Blackfan syndrome |
Familial erythrophagocytic histiocytes |
Dyskeratosis congenita |
Schwachman-Diamond syndrome |
Severe combined immune deficiency and related disorders |
Wiskott-Aldrich syndrome |
Inborn errors of metabolism |
Acquired autoimmune diseases |

Fig. 1.2
Common indications for allogeneic and autologous HSCT (Adapted from Pasquini and Zhu [1]; with permission)
Sources of Hematopoietic Stem Cells
Hematopoietic stem cells (HSCs) can be found in a variety of human tissues, but for clinical purposes the most commonly used sources are peripheral blood, bone marrow, and umbilical cord blood. Each source has its unique advantages and drawbacks in clinical settings.
Peripheral Blood Mobilized Stem Cells
Peripheral blood is the most common source of hematopoietic progenitor cells (HPCs); 75 % of allogeneic HSCTs use mobilized stem cells in the unrelated donor setting [15]. The benefits of peripheral blood mobilized stem cells in the matched sibling setting are faster hematopoietic engraftment and fewer infectious complications due to higher numbers of CD34+ HSCs than in bone marrow grafts [16, 17]. Moreover, the procedure is less invasive than bone marrow harvest and more donor-friendly. In healthy donors, the concentration of CD34+ cells is 0.06 % in peripheral blood and 1.1 % in bone marrow [18]. This percentage can be readily augmented by growth factor priming and by chemotherapy in the setting of autologous stem cell transplantation. After four doses of granulocyte colony-stimulating factor (G-CSF) at 12 μg/kg per day, the mean peripheral blood CD34+ count increased from a baseline of 3.8 × 109/L to 61.9 × 109/L [19], allowing apheresis of CD34+ HPCs from the peripheral blood for infusion purposes. The peripheral blood stem cell (PBSC) graft has 10 times higher concentrations of T cells, monocytes, and NK cells compared with bone marrow graft, and the higher concentration of T cells in the PBSC grafts has been correlated with a higher incidence of acute and chronic GVHD in both related and unrelated HSCTs [15, 20]. The optimal CD34 dose is 2 to 5 × 106 cells/kg in the allogeneic HSCT setting; a CD34 dose exceeding 8 × 106 cells/kg has been associated with the development of chronic GVHD [21].
Bone Marrow Hematopoietic Stem Cells
Traditionally, bone marrow has been the source of HPCs used in the sibling and unrelated-donor settings. Five to 10 mL of bone marrow is typically obtained from healthy donors from the posterior iliac crest under general anesthesia, with a goal of 10–15 mL/kg of recipient weight, corresponding to a marrow volume of 700–1500 mL for an adult donor. The goal is to collect a minimum total nucleated cell dose of 2 × 108 cells/kg for successful engraftment. The bone marrow graft has lower numbers of T cells, correlated with a lower incidence of chronic GVHD in the unrelated-donor setting, although the graft failure rate is higher at 9 % [15].
Umbilical Cord Blood Stem Cells
Umbilical cord blood (UCB) contains high numbers of HPCs, and double cord stem cell transplants have been successfully used in adults for hematologic malignancies [22]. When mismatched UCB HSCT was compared with mismatched bone marrow HSCT for acute leukemia in the myeloablative setting, the transplant-related mortality, treatment failure, and overall mortality were similar [23], indicating that UCB is a viable source of HSCs for treatment of advanced hematologic malignancies. The major disadvantage to the use of UCB is the significant delay in neutrophil engraftment (average 27 days) and platelet engraftment (average 29 days) compared with bone marrow and PBSC graft sources. This delay in hematopoietic engraftment increases transfusion requirements and the risk of infectious complications. Graft failure is a major concern with UCB transplants (10 % in RIC and 20 % in myeloablative conditioning [24, 25]) because of the low numbers of passively transferred T cells.
In the HLA-matched sibling and unrelated-donor setting in adults, HSCT is most often performed using PBSCs as the source of stem cells (Fig. 1.3). In the pediatric population, bone marrow continues to be the preferred source of HSCs.


Fig. 1.3
(a, b) Increasing utilization of peripheral blood stem cells (PBSCs) as the source of hematopoietic stem cells in the sibling and unrelated donor settings in adults (Adapted from Pasquini and Zhu [1]; with permission)
Stem Cell Donors
Matched Related Donor
This type of allogeneic HSCT involves a donor who is an HLA-matched sibling of the recipient. The formula for calculating the chances of a particular person having an HLA-matched sibling is 1 − (0.75)N, where N denotes the number of potential sibling donors. In general, a patient with one sibling has a 25 % chance of having a match. The average American family size usually limits the success of finding a family donor to approximately 30 % of patients.
Matched Unrelated Donor
If the patient needs an allogeneic transplant and a donor cannot be found within the family, the identification of a matched unrelated donor (MUD) is accomplished by searching the computer files of the National Marrow Donor Program (NMDP) as well as other, international registries (Bone Marrow Donors Worldwide: BMDW). Through international connections, the NMDP searches more than 27.8 million potential donors worldwide as of 2016 (https://www.bmdw.org).
Haploidentical Stem Cell Donors
A recent source of hematopoietic stem cells that has emerged is from a haploidentical donor, which refers to complete half mismatch (4 of 8 HLA loci) from a related donor. This technique has greatly expanded the applicability of HSCT for patients with hematologic malignancies, as almost everyone will have a haploidentical donor (parent, child, or half sibling) who can potentially be used as an HSC donor source. The haploidentical donor is readily available and the cost of collection is lower than for MUD or UCB donors. Early attempts at haploidentical transplants were unsuccessful owing to severe GVHD in T-replete grafts and graft rejection in T-depleted grafts. The use of posttransplantation cyclophosphamide (Cytoxan), pioneered by a group at the Johns Hopkins Oncology Center [26], has allowed successful engraftment with low rates of acute and chronic GVHD. The risk of graft failure can be reduced by increasing the CD34+ stem cell dose, as shown by a group at the University of Perugia, Italy [27] (Fig. 1.4).


Fig. 1.4
Distribution of allogeneic HSCT based on various donor sources (Adapted from Pasquini and Zhu [1]; with permission)
In 2006, MUD HSCTs exceeded the number of sibling transplants for the first time, and the gap continues to widen. From 2003 to 2011, UCB transplantation increased steadily in popularity, but owing to the rapid adoption of haploidentical donors with posttransplantation cyclophosphamide, the number of haploidentical HSCTs exceeded UCB transplants in 2014 and now accounts for 11 % of all allogeneic HSCT in the United States [1]. The survival data for MUD transplantation is similar to sibling HSCT outcomes in certain disease states [28].
Conditioning Regimens
The conditioning regimen used in the allogeneic setting has a dual purpose: The therapeutic component is designed to eliminate tumor cells, and the immunosuppressive component is intended to prevent host immune responses from rejecting the transplanted donor cells. The doses of radiation therapy and chemotherapy employed take advantage of the steep dose-response curve that exists for many malignancies. These doses have been established on the basis of the limitations of other nonhematopoietic organs, such as the liver and lungs. The combined chemotherapy agents employed have a nonoverlapping toxicity profile. Based on their intensity, conditioning regimens are divided into myeloablative (MA) regimens, nonmyeloablative regimens, and reduced-intensity conditioning (RIC) regimens. The type of regimen chosen for any individual patient depends on a variety of factors such as age, performance status, disease status, the risk of relapse, and comorbidities.
Myeloablative Regimens
This is traditionally the most commonly used type of conditioning regimen. It can be administered with a combination of radiation therapy with total body irradiation (TBI) doses in excess of 5 Gy or a busulfan dose greater than 8 mg/kg. MA regimens are associated with profound pancytopenia within 1–2 weeks of conditioning, which is irreversible and requires infusion of autologous or allogeneic stem cells to restore normal hematopoiesis. Busulfan-based regimens are most commonly used worldwide for allogeneic transplantation, but the oral administration of busulfan leads to unpredictable absorption, which has been correlated with relapse (low absorption) and increased toxicity (increased absorption). Studies with an intravenous formulation of the drug have shown much more predictable pharmacokinetics, less toxicity, and good survival when used in the transplant regimen. Studies have shown that when an intravenous formulation of busulfan is used, it is associated with a decreased incidence of hepatic venoocclusive disease and the 100-day survival is significantly higher [29]. The advent of therapeutic drug monitoring using pharmacokinetic dosing strategy can further help in achieving steady-state drug levels in the desired range, thereby improving HSCT outcomes [30].
Reduced-Intensity Conditioning
Reduced-intensity conditioning (RIC) uses lower doses of chemotherapy, with or without TBI, and relies on immunosuppression to facilitate engraftment of donor stem cells. These conditioning regimens are associated with significant lymphopenia, allowing successful engraftment of donor HSCs in the recipient. The major therapeutic effect that results from this type of transplant is a graft-versus-tumor effect, because the nonmyeloablative regimen has limited long-term antitumor effect. Some disorders, such as CML, AML, and low-grade lymphoma, are particularly sensitive to this approach.
RIC transplantation is used primarily for patients who are older or who have comorbid conditions that might increase the risk of a fully ablative regimen. The most common regimens use fludarabine combined with either melphalan or busulfan or with a single fraction of TBI, followed by infusion of either donor bone marrow or peripheral blood–derived stem cells. All patients still require posttransplant immunosuppression similar to all other patients receiving non–T-cell–depleted transplants. For patients receiving either a matched sibling or fully matched unrelated transplant, the engraftment of donor cells after RIC is usually 100 % by day 30–60 after transplantation, and the immunosuppressive medications are tapered over a few months. Although the chemotherapy does have antitumor activity in this type of transplant, the major factor in eliminating the malignancy is the donor immune system. As in the fully ablative transplant setting, the presence or absence of GVHD influences the outcome.
Graft-Versus-Host Disease Prophylaxis and Treatment
GVHD can be broadly divided into acute and chronic, based on clinical manifestations rather than on the temporal relation to the time of transplantation. Acute GVHD includes classic acute GVHD (manifested by maculopapular rash, GI symptoms, or cholestatic hepatitis) and persistent, recurrent, or late-onset acute GVHD (occurring more than 100 days after HSCT). Chronic GVHD (cGVHD) comprises classic chronic GVHD, presenting with clinical manifestations attributable to GVHD alone, and overlap syndrome, which has diagnostic or distinctive cGVHD manifestations together with features typical of acute GVHD [31].
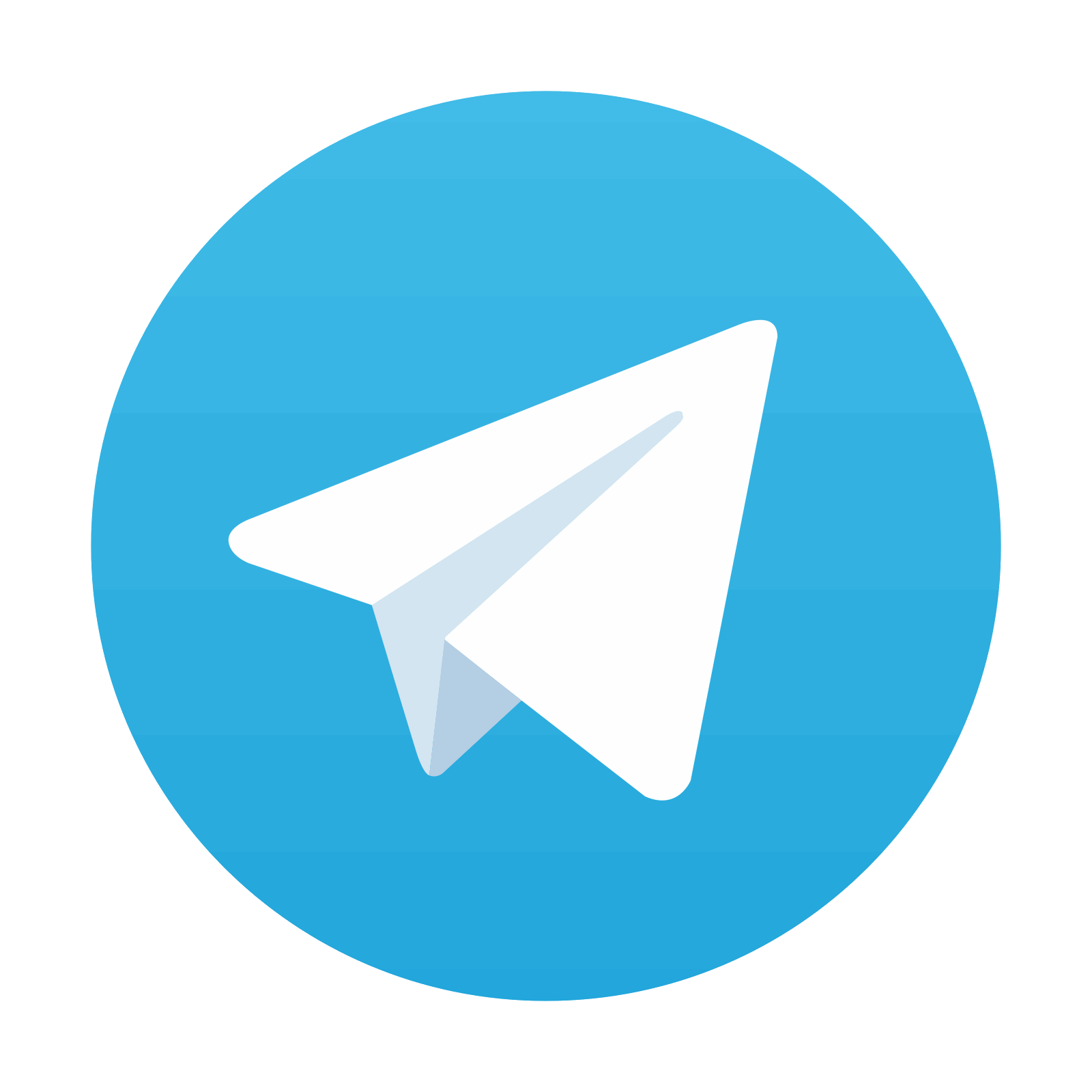
Stay updated, free articles. Join our Telegram channel

Full access? Get Clinical Tree
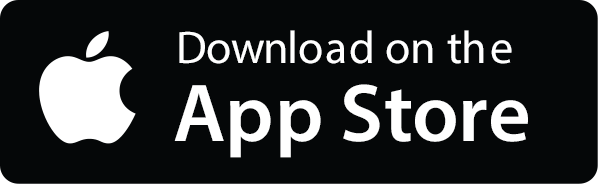
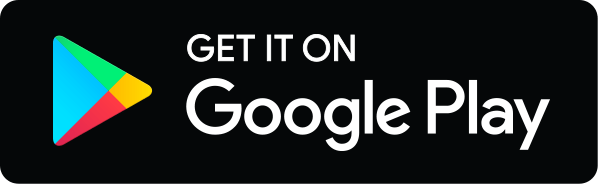