Eosinophilic
Neutrophilic
Stimulus for inflammation
Allergen
Microorganism
Cell-mediated immunity
Th2 predominant
Th1 predominant
Key cytokines
IL-4, IL-5, IL-9, IL-13
IL-1β, IL-6, IL-8, TNF-α
Granulocyte-specific inflammatory products
Major basic protein → epithelial desquamation, mucus-cell hyperplasia, and mucosal edema
Proteases and superoxides → mucociliary dysfunction and mucin hypersecretion
Other pathogenic features
Superantigen production
Increased fibrosis
Eosinophil-Dominant Inflammation
Chronic rhinosinusitis with predominantly eosinophilic inflammation refers to a group of entities including allergic fungal rhinosinusitis, eosinophilic mucin rhinosinusitis, and nasal polyposis. In this group of patients, inflammation is predominantly driven by immunoglobulin E (IgE).
The inciting event is the exposure to an antigen, which can arise from the environment or be produced by organisms residing in the nasal mucosa. Antigen-processing cells process the allergen into a peptide, which is then presented to type 2 T-helper (Th2) cells within major histocompatibility complex II (MHC II). Antigen-processing cells include dendritic cells, mast cells, macrophages, eosinophils, and natural helper cells [1]. An activated Th2 cell produces the cytokines interleukin (IL)-4, IL-13, IL-5, and IL-9. IL-4 and IL-13 stimulate the production of IgE by B-cells. IL-13 also assists in eosinophil migration from blood vessels to the nasal mucosa [2]. IL-5 facilitates eosinophilic chemotaxis and prolongs their survival by reducing apoptosis, while IL-9 leads to the propagation of mast cells [3].
Ongoing allergen exposure leads to cross-linking of IgE, now bound to the surface of sensitized mast cells. Activated mast cells release their contents, including histamine, leukotrienes, tumor necrosis factor (TNF-α), and cytokines. The resultant effect is an inflammatory and edematous state with increased vascular permeability and mucus secretion, as well as mucociliary function impairment. In addition, the mediators released by mast cells are chemotactic for eosinophils, which are the dominant inflammatory cells in the late allergy phase. Eosinophils contain major basic protein (MBP), a granule that contains cytotoxic material that when deposited causes epithelial desquamation, thickening of the basement membrane, mucus-cell hyperplasia, and edema [4]. Repeated exposure to allergens perpetuates the above pathways, causing ongoing eosinophil deposition and the associated inflammation and injury of sinonasal epithelium. Figure 13.1 outlines the common features of the eosinophilic inflammatory response.
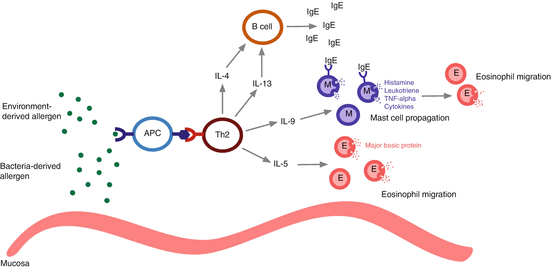
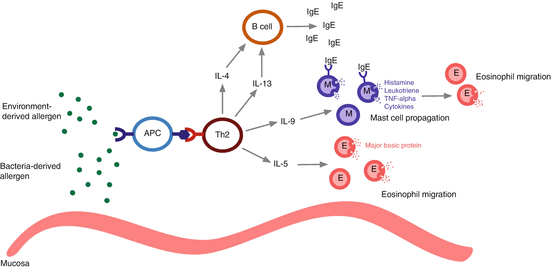
Fig. 13.1
Mechanism of eosinophilic inflammation. APC antigen-presenting cell, Th2 type 2 helper T-cell, IL interleukin, IgE immunoglobulin E, M mast cell, E eosinophil, TNF–α tumor necrosis factor alpha
A subset of patients with eosinophilic disease has negative atopy testing. The working hypothesis is that inflammation in these patients may be driven by allergen exposure; however, IgE production occurs locally, and there is a lack of systemic symptoms and serum measures of atopy. This is supported by studies that have shown an increase in nasal lavage IgE levels following allergen provocation [5–7]. Therefore, the local allergic state plays an important role in CRS and may not be reflected at the systemic level. The presence of local allergy in the absence of corresponding systemic atopy is referred to as ‘entopy’ [8]. Another possible explanation for the eosinophilic sinus mucosal disease is leukotriene. This is further discussed in Chap. 18.
In some eosinophilic CRS, the mucosa is frequently colonized by Staphylococcus aureus, with carrier rates up to 63.6 % in CRS with nasal polyps (CRSwNP) [9]. This is compared to a carrier rate of 27.3 % in CRS without nasal polyps (CRSsNP). Staphylococcus aureus can produce pathogenic exotoxins, otherwise known as superantigens by their ability to mass activate T cells [10]. The superantigens bind to antigen-presenting cells as well as T lymphocytes. The activation of T lymphocytes leads to T-cell proliferation, the production of IL-5, and subsequent eosinophil infiltration and associated inflammation. Superantigens also stimulate B cells to produce superantigen-specific IgE [9]. Finally, superantigens are associated with the ongoing activation of mast cells. This amplification of the inflammatory response associated with bacterial colonization is becoming a recognized phenomenon in the pathogenesis of CRS.
Neutrophil-Dominant Inflammation
Neutrophil-dominant inflammation is commonly seen in CRSsNP. It is associated with bacteria, ciliary dyskinesis, cystic fibrosis, or a foreign body [11]. Once triggered, various inflammatory pathways can continue despite the removal of the pathogen from the mucosa, resulting in a chronic inflammatory state.
Initially, there is exposure of the nasal mucosa to microorganisms. In response, macrophages are stimulated to produce IL-1β and TNF-α. This subsequently stimulates epithelial cells to secrete the cytokines IL-6 and IL-8, as well as granulocyte macrophage colony-stimulating factor (GM-CSF). Mucosal epithelial cells can also be directly stimulated by bacteria to secrete IL-8 [12]. IL-8 stimulates transendothelial migration of neutrophils by increasing adhesion molecules on both the neutrophils as well as the vasculature [12, 13]. Neutrophils, once migrated to the nasal mucosa and sinus exudate, also secrete IL-8, as well as leukotriene B, thereby propagating the inflammatory response. It has been proposed that IL-8 may play a role in the formation of neutrophil-dominant polyps that are distinct from the typical eosinophil-dominant polyps seen in the case of allergy-associated CRS [13].
In addition to secreting IL-8, neutrophils also produce proteases and superoxides, leading to mucociliary dysfunction and the stagnation of sinus drainage [12]. These proteases and superoxides also trigger hypersecretion of mucin and ongoing production of proinflammatory cytokines [14].
An upregulation of transforming growth factor beta (TGF-β) is also seen in CRSsNP. TGF-β plays a key role in collagen deposition and fibrosis by stimulating extracellular matrix protein production as well as inhibiting the breakdown of these proteins. TGF-β contributes to the regulation of matrix metalloproteinases (MMPs). MMPs are a group of proteolytic peptidases that control tissue remodeling and help recruit immune cells. Conversely, TGF-β is downregulated in CRSwNP. This increase in fibrosis is another differentiating feature of CRSsNP from CRSwNP [15, 16]. Figure 13.2 outlines the common features of the neutrophilic inflammatory response (see Table 13.1).
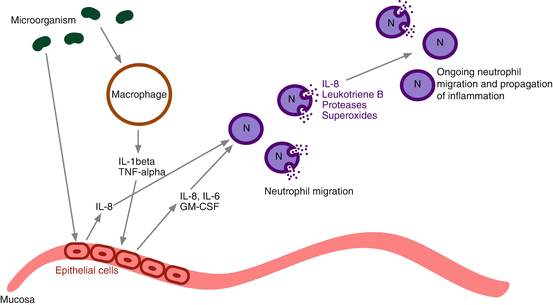
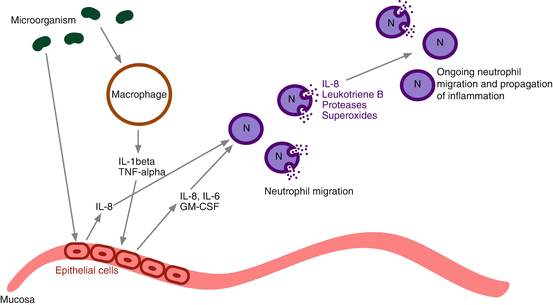
Fig. 13.2
Mechanism of neutrophilic inflammation. IL interleukin, TNF–α tumor necrosis factor alpha, GM–CSF granulocyte macrophage colony-stimulating factor, N neutrophil
Antibiotics as Anti-inflammatories
The primary goal of CRS management is the reduction of sinonasal mucosal inflammation in order to promote normal physiologic functioning. Common first-line treatment options include high-volume sinonasal saline irrigations, topical corticosteroid therapies, and systemic therapies such as short-course oral corticosteroids and oral antibiotics. Endoscopic sinus surgery (ESS) is an important treatment modality for patients with refractory CRS as it provides open and accessible sinus cavities in order to facilitate the delivery of topical anti-inflammatory therapy [17]. In the setting where the above mainstay therapies fail to alleviate symptoms, second-line therapies can be considered. These therapies include prolonged anti-inflammatory antibiotics, antihistamines, anti-IgE monoclonal antibody (omalizumab), and anti-IL-5 monoclonal antibody (mepolizumab). However, the evidence for several of these second-line agents in the setting of CRS is still preliminary.
Antibiotics have been used liberally in CRS as bacteria are frequently cultured in these patients [18]. However, their exact therapeutic role is not well established. There is growing evidence that certain antibiotics have action beyond being bacteriostatic or bactericidal. Studies suggest that certain antibiotics may exert anti-inflammatory effects, thereby addressing the underlying pathologic state in CRS. For this reason, certain antibiotics can be added to the list of second-line agents when standard therapies fail. The following sections will discuss the antibiotics that have been used as anti-inflammatory agents in the management of CRS.
Macrolides
First discovered in 1952, macrolides are derivatives of polyketides, which are metabolites produced by bacteria, fungi, plants, and animals [19]. They exert their bacteriostatic effects through the disruption of bacterial ribosomal function. This class of antibiotics includes erythromycin, clarithromycin, roxithromycin, and azithromycin.
Macrolides are most effective against Gram-positive bacteria and are most commonly employed in upper respiratory tract infections. Having efficacy for Streptococcus pneumoniae, Haemophilus spp., Moraxella catarrhalis, and Mycoplasma pneumoniae, macrolides remain as the mainstay for the treatment of community-acquired pneumonia.
While conventionally they are used for their antibacterial effects, macrolides are increasingly being investigated for their anti-inflammatory and immunomodulatory effects [19–22]. Specifically, macrolides have been hypothesized to have a role in regulating cytokine production, neutrophil apoptosis, and mucociliary clearance.
Macrolides as Anti-inflammatories
In vitro studies have shown that a primary mechanism by which macrolides act as an anti-inflammatory is through its ability to inhibit nuclear factor kappa B (NFκB). NFκB facilitates the transcription of the inflammatory genes that when expressed leads to the production of IL-2, IL-6, IL-8, TNF-α, GM-CSF, and other inflammatory mediators [23]. Various viruses and bacteria can activate the NFκB pathway of inflammation, and exposure to macrolides leads to a decrease in the expression of these inflammatory genes. The downregulation of the expression of certain inflammatory genes has been confirmed in vivo, where several studies have demonstrated a decrease in local sinonasal mucosal levels of IL-8 following macrolide therapy [13, 24, 25].
Macrolides have several other beneficial effects in the setting of CRS. First, it improves mucociliary function of the sinonasal cavity. This was shown in two animal models, whereby 2 weeks of roxithromycin increased mucociliary clearance [26, 27]. Second, macrolides have been found to normalize mucus production and consistency [28, 29]. Third, macrolides decrease neutrophil accumulation and encourage their apoptosis, which reduces the damage caused by the release of cytotoxic substances [24, 30, 31]. Finally, macrolides interfere with TGF-β function by blocking its receptor [32]. This decreases collagen deposition and the subsequent fibrosis that is seen in TGF-β upregulation. Figure 13.3 outlines the mechanisms of action for macrolides as anti-inflammatories.
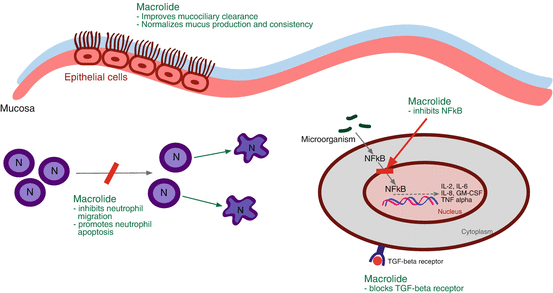
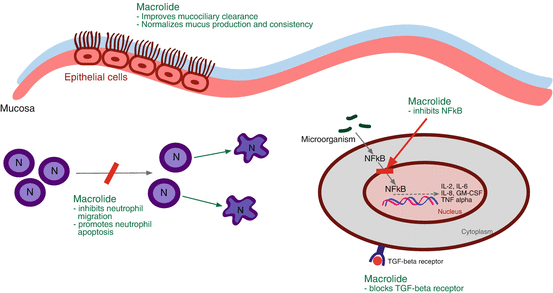
Fig. 13.3
Macrolide anti-inflammatory mechanism of action. N neutrophil, NFκB nuclear factor kappa B, IL interleukin, GM-CSF granulocyte macrophage colony-stimulating factor, TNF-α tumor necrosis factor alpha, TGF-β transforming growth factor beta
Clinical Efficacy
Various studies have investigated the utility of macrolide use in the management of CRS; however, there are only two randomized controlled trials evaluating the effectiveness of prolonged macrolide therapy in patients with CRS. In 2006, Wallwork performed a randomized, double-blind placebo-controlled trial evaluating oral roxithromycin 150 mg daily for 3 months in CRSsNP [20]. The results demonstrated that macrolide therapy provided significant improvements in both quality of life (QoL) and endoscopic findings at the end of the treatment period. Quality of life, however, was no longer significantly improved at 3-month follow-up after stopping macrolide treatment. Additionally, they identified that patients with decreased serum IgE levels experienced greater benefit from macrolide therapy. In 2011, Videler et al. performed a randomized, double-blind placebo-controlled trial evaluating oral azithromycin 500 mg daily for 3 days followed by 500 mg once a week for 11 weeks [21]. In contrast to the study by Wallwork et al., their results failed to demonstrate a significant improvement in both QoL and endoscopic findings [21]. A possible explanation for the lack of significant results obtained by Videler et al. is that 500 mg given once a week was an underdose of the amount that would be required to improve symptoms.
There are ten observational studies that have investigated symptom improvement with macrolide therapy [33–42]. All studies report symptom improvement in over 50 % and endoscopic improvement in 40–70 % of study subjects; however, none used a validated instrument to evaluate sinus symptomatology. There are two studies that retrospectively examined the role of macrolide antibiotics following endoscopic sinus surgery. In both studies, a significant improvement in symptoms was found in the group that was treated with long-term macrolides [38].
In 2013, an evidence-based review with recommendations by Soler et al. summarized the evidence on prolonged macrolide therapy in CRS [43]. They identified 17 studies, both retrospective and prospective. They concluded that because a consistent benefit was seen in numerous observational studies and one controlled study, macrolide antibiotics would be a reasonable option in patients with CRS. Furthermore, macrolides should be especially considered in patients with low serum IgE levels.
Complications
Complications of macrolide antibiotics include hypersensitivity reactions, hepatotoxicity, and gastrointestinal upset. Other reported complications include ototoxicity and prolonged QT interval with subsequent dysrhythmia. There is also a risk of adverse drug-to-drug interactions with statins, antidepressants, antiepileptics, and methotrexate. Finally, there is the theoretical risk of antibiotic resistance; however, none of the abovementioned studies reported cases of bacterial resistance as a result of prolonged macrolide use.
Summary: Macrolides
At this time, available evidence suggests that a long course of at least 12 weeks of low-dose macrolide antibiotics may help alleviate symptoms of CRS that are refractory to conventional therapy. The evidence suggests that macrolide therapy is most effective in patients who have nonallergic CRS with low serum IgE levels. Those who have allergic disease and predominantly eosinophilic inflammation are less likely to experience clinical improvement.
In patients with refractory CRS, it is reasonable to consider trial therapy with a macrolide [44]. Furthermore, it may be prudent to obtain a serum IgE level to identify those individuals who would most likely benefit from macrolide therapy. Table 13.2 summarizes the macrolide antibiotics that have been used in CRS, their dosages, and contraindications.
Table 13.2
Summary of macrolide treatment options
Macrolide | Dose (mg) | Frequency | Duration (weeks) | Contraindications |
---|---|---|---|---|
Clarithromycin | 250 or 500 | QD | 12 | Hypersensitivity to macrolide antibioticsa |
Concurrent statin therapy | ||||
Azithromycin | 500 | Once a week dose after daily dose × 3 | 12 | Previous hepatic dysfunction secondary to azithromycin use |
Erythromycin | 250 | BID | 12 | Concurrent use of pimozide, cisapride, ergotamine, and statin |
Roxithromycin | 150 | QD | 12 | Porphyria |
Tetracyclines
Tetracyclines are a group of mostly bacteriostatic antibiotics. They inhibit bacterial protein synthesis by binding to the 30S ribosomal subunit. The first tetracycline discovered was chlortetracycline. Five more tetracyclines have been developed since that time, including doxycycline, tetracycline, minocycline, oxytetracycline, and demeclocycline.
They are broad-spectrum antibiotics with action against various organisms, including Gram-positive and Gram-negative bacteria, as well as atypical pathogens such as Rickettsia spp., Chlamydia spp., Mycoplasma pneumoniae, and Treponema spp.
Tetracyclines as Anti-inflammatories
There has been great interest in studying the non-antimicrobial properties of tetracyclines. One of the most well-known properties is its ability to inhibit matrix metalloproteinases (MMPs). Doxycycline decreases MMP activity by both direct inhibition of MMP molecules as well as the downregulation of their production [45]. MMPs mediate proteolysis of the extracellular matrix and play an important role in connective tissue remodeling. As such, MMPs are involved in the pathogenesis of wound healing, tumor invasion, and inflammation [46–50]; therefore, inhibiting MMPs may reduce the inflammatory response in the mucosa of patients with CRS.
Tetracyclines exert an anti-inflammatory effect through other mechanisms, including the stabilization of reactive oxygen specifies, inhibition of nitric oxide synthesis, and reduction of neutrophil chemotaxis [45, 51–53]. Additionally, tetracyclines have been implicated in decreasing IgE production, while levels of IgA, IgM, and IgG are unaffected [54, 55]. A possible explanation for this is that it interferes with terminal B lymphocyte differentiation and class switching [56]. By virtue of decreasing IgE, it also decreases IgE-mediated responses such as the mast cell-dominant late allergy phase [54]. Figure 13.4 outlines the mechanisms of action for tetracyclines as anti-inflammatories.
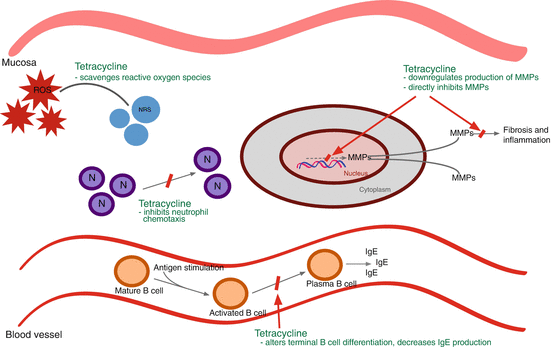
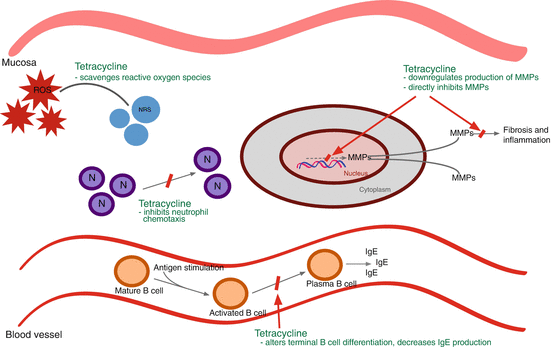
Fig. 13.4
Tetracycline anti-inflammatory mechanism of action. ROS reactive oxygen species, NRS nonreactive species, N neutrophil, MMP metalloproteinases, IgE immunoglobulin E