div class=”ChapterContextInformation”>
8. Comparison of Four Different Fuller’s Earth Formulations in Skin Decontamination
Keywords
4-CyanophenolAdsorption capacityFuller’s earthSkin decontaminationFuller‘s earth suspensionAbbreviations
- 4-CP
4-Cyanophenol
- ATR-FTIR
Attenuated total reflectance Fourier transform infrared
- CWA
Chemical warfare agents
- FE
Fuller’s earth
- SC
Stratum corneum
- TIC
Toxic industrial chemicals
- TS
Tape stripping
- UV
Ultraviolet
Introduction
The first chemical warfare occurred during First World War with the use of chemical warfare agents (CWA) causing dramatic short-term health effects and long-term effects in survivors. Worldwide disasters are always relevant with the potential use of chemical, biological, radiological and nuclear agents.
CWA include (i) haemoglobin-binding agents (i.e. blood agents), (ii) choking agents (e.g. chlorine) [8], (iii) vesicating agents (e.g. sulfur mustard) [26] and (iv) organophosphorous compounds (COP) (i.e. nerve agents) [24]. Furthermore, toxic industrial chemicals (TIC) can be released such as in the world’s worst industrial disaster [3], at Union Carbide, Bhopal, in December 1984, involving the poisonous methyl-isocyanate (MIC), causing thousands of deaths and abysmal long-term consequences after exposure [2]. After a chemical explosion on July 10, 1976, in Seveso, Italy, 2,3,7,8-tetrachlorodibenzo-para-dioxin (TCDD), a widespread contamination induced increased cancer incidence in the exposed population more than 30 years later [39]. Toxicity of CWA and TICs is associated with (i) acute epithelial reactions and (ii) long-term morbidity depending on exposure time. Acute effects including irritation, inflammation, oxidative stress and necrosis especially affect respiratory tract, skin, eyes and upper digestive tract mucosa causing dramatic damage.
Oxidative stress in skin injuries, caused by vesicating agents, such as sulfur mustard, a highly reactive alkylating agent, and subsequent DNA lesions are leading to the acute toxicity producing massive inflammation in case of skin contact as well as to the long-term carcinogenicity [1]. Similar long-term effects have been described for other CWA. Acute exposure to organophosphorous compounds involves an acute cholinergic crisis in addition to signs of neurotoxicity that could develop neurocognitive deficits and structural brain damage over the long term. Although [25] cancer incidence after industrial accidents such as Seveso continues to be investigated, chloracne appears to be the only effect linked with sufficient certainty to dioxin exposure [14].
Whereas inhalation of toxics may be harmful for the breathing system, skin is the most external and relatively large (~ 2 m2) organ primarily exposed to toxic or harmful chemical [18, 19].
Skin decontamination in victims is thus an essential phase of action aimed to (i) prevent the local damage, transcutaneous absorption and subsequent local and systemic toxicity, (ii) limit skin damage and (iii) facilitate skin repair.
At present, water is largely proposed for mass decontamination [7], for it is easily provided, e.g. from fire trucks. The alternative to such “wet” decontamination is “dry” decontamination based on adsorptive properties of powders, e.g. talcum, flour or clays [21].
Fuller’s earth (FE) [23], an alumina silicate, chemically inert, abundant in nature, known for its adsorptive properties, provides decontamination opportunities for chemical, biological, radiological and nuclear agents [22, 36]. FE, currently used in firefighter decontamination processes in France [34], is provided as powder in container and prefilled gloves. In the present study we investigate the FE as furnished by our official supplier (i) in its original form, (ii) dispersed in water, in order to optimize the skin decontamination process after short exposure to water-soluble chemicals.
Therefore, 4-CP [33] ranged in aqueous solutions is used as tracer model to assess adsorption capacity of the fuller’s earth in vitro and its efficiency on porcine skin decontamination ex vivo. The 4-CP was used as a model contaminant for several reasons: (i) its IR signal allows a rapid and noninvasive quantification of uptaken chemical agent onto the skin; (ii) it has been used to model cutaneous absorption of chemical both in vitro and in vivo [28] and (iii) it possesses a similar hydrophobicity to most CW agents and TICs, as indicated by its logPoct/water (table 0 supplement data) while its toxicity is much lower, thus allowing simple, safe and short-duration studies with diverse possible exposure scenarios.
Firstly, Fuller’s earth adsorption capacity is carried out in vitro for three 4-CP aqueous solutions in function of time ensuring that the resulting benefits have taken into account constraints challenging operational realities (i.e. time from 5 min to 120 min).
The first study is performed with a fixed amount of FE (1 g) in function of contact time ranging from 5 to 120 min, whereas we assessed the impact of amount of FE on adsorption capacity for the 4-CP after both contact times 15 and 60 min.
Then, contamination is designed ex vivo by exposing porcine skin to 4-CP in aqueous solution during a short time exposure followed by water showering or by FE’s application (i) as free powder, (ii) loaded on adhesive tape, (iii) on powdered glove or (iv) as a suspension. Extent of 4-CP skin penetration and subsequent efficiency of FE-based decontamination methods are investigated by tracking skin 4-CP content using attenuated total reflectance Fourier transform infrared spectroscopy (ATR-FTIR). Fifteen-minute exposure was defined (i.e. minimal delay expected in case of a real-life incident before initiation of the decontamination procedure).
Materials and Methods
Materials
FE (ρ = 0.7 kg.m−3) is presented as a free powder (stored in bottle) with adsorptive properties available for firefighter decontamination process (NBC-Sys 42,408; St-Chamond, France) [17]. 4-Cyanophenol (4-CP) [MW 119.1 g.mol−1, Log P 1.6, water solubility 8.98 g.L−1], with purity of 95%, was purchased from Aldrich (St-Quentin-Fallavier, France). Aqueous solutions of 4-CP were extemporaneously prepared with purified water (0.055 μS.cm−1) produced by PURELAB Option before each set of measurements at 24–25 °C and sonicated for 5 min to ensure 4-CP total dissolution.
Instrumentation
UV spectrophotometer (Jasco V-630 spectrophotometer) was used to measure 4–CP absorbance at the wavelength 246 nm with specific disposable cuvettes (7591 50) of 1.5 mL (BRAND GMBH, 97861 Wehrheim, Germany). Quantitative analysis data were treated by software Spectramanager2.
Centrifuge sigma 3-16P (Fisher Scientific, Illkirch, France), ultrasonic equipment 89878 (Biobloc scientific, Illkirch, France), pH meter HI 991002 (Hanna ORP Meter, Sigma-Aldrich, France), magnetic stirrer (RH Basic 2 IKA, Brumath, France).
ATR-FTIR analyses were conducted from 400 to 4000 cm−1 using an infrared spectrometer (Perkin Elmer UATR Spectrum two, Waltham, MA 02451, USA) equipped with a triglycine sulfate (TGS) pyroelectric thermic mid-detector. The sample compartment is a horizontal internal reflection accessory holding a diamond crystal (θ = 42°) with a refractive index 2.4. Therefore, an evanescent wave at the reflective surface was entering for a path of 1–2 μm into the sample in contact with the diamond producing a spectrum collected on database. This mono reflection system requires a spectrum acquisition time of 2 min [38].
Methods
Kinetic Adsorption Studies (In Vitro)
- (i)
4-CP aqueous solutions C 0 (10 mg.L−1, 100 mg.L−1, 500 mg.L−1) were treated by 1 g of FE and left in contact (t = 5, 15, 30, 45, 60, 90 and 120 min).
- (ii)
The 4-CP aqueous solution (C 0 = 10 mg. L−1) was treated by (0.01, 0.1, 1) g of FE, whereas (C 0 = 100 mg. L−1) and (C 0 = 500 mg. L−1) by (0.1, 1) g of FE (t = 15, 60 min).
These obtained suspensions were agitated by magnetic stirrer for a determined time t (min) ranging from 5 to 120 min, taken into microcentrifuge tubes (02 681-291, 10 mL, Fisher Scientific, Illkirch, France), centrifuged at 3000 g for 12 min, in which supernatant was filtered (pores ∅ 0,1 μm, ANOTOP™ 10 filter; Whatman GmbH Dassel, Germany). Absorbance of residual 4-CP in the filtered supernatant (C L) was measured by UV spectrometer at 246 nm at 25 °C and pH 7. Calibration was established for 4-CP aqueous solutions ranged from 2.5 to 12.5 mg.L−1 [31]. The tests were performed in triplicate.
C L (mg. L−1) measured in function of time was used to determine the adsorbed amount of 4-CP (mg) per gram of FE in real-time kinetic and at equilibrium (C FEmax mg.g−1), whereas the impact of FE’s amount on adsorption capacity was assessed by percentage removal of 4-CP (%) for each treated solution for both times 15 and 60 min [37].
Furthermore, the data were tested to fit the rate constant of adsorption (k 1, k 2) of 4-CP onto FE, with the first-order kinetic model of Lagergren [40] and the second-order kinetic model of Ho and Mac Kay [11].
Porcine Skin, Contamination: Decontamination
Unscalded porcine ears, obtained from a local slaughterhouse, were washed with purified water, softly wiped and stored in sealed plastic bags at – 20 °C within 12 hours after animal sacrifice. On the day of experiment, porcine ears were slowly thawed at 4 °C, and hair were clipped; 1.54 cm2 explants were cut with a biopsy punch. Porcine skin is advantageously easily prepared and the whole skin structure is conserved, as the skin is not detached from the subcutaneous tissues, which helps maintain skin hydration. All experiments were performed on appropriate laboratory equipment , easy to clean and anti-slip (silicone holder).
Contamination by 4-CP Aqueous Solution
Preliminary calibration , for reference 4-CP, powder (depot, 50 mg; 8 scans) and its aqueous solutions (21–105 mM, depots 100 μL; 8 scans) were analysed by ATR-FTIR spectroscopy.
Then, accuracy of 4-CP detection method in the skin was tested by applying 100 μL of 4-CP aqueous solutions (21–105 mM) onto porcine skin explants (skin area, 1.54 cm2; n = 4). The volume of 100 μL allowed adequately covering skin disc surface (1.54 cm2) with contaminant. In most cases, solution remained on the skin surface and, if any liquid run off the skin, the experiment was reconducted with a new specimen. After 15 min of contact unabsorbed 4-CP solution was gently wiped from the skin surface and skin explants were immediately analysed by ATR-FTIR (8 scans). ATR-FTIR spectroscopy was successfully exploited for 4-CP tracking within the SC layers by integration of the cyanyl (C ≡ N) stretching vibration absorbance peak (i.e. 2231 cm−1), as in this spectral region (2250 and 2200 cm-1.), low interference with SC enables accurate evaluation of skin 4-CP content [28, 35].
Next, 100 μL of 105 mM 4-CP saturated solution was applied onto porcine skin explants (skin area, 1.54 cm2; n = 4) for 1, 5 and 15 min, in order to appreciate 4-CP penetration as a function of time as described previously [20].
Finally, after 15 min of skin exposure to 105 mM 4-CP aqueous solution, the profile of 4-CP penetration was assessed by successive tape stripping (n = 4) (pre-cut tape strips, 19 mm × 50 mm; Scotch 3M, Cergy, France) and concomitant ATR-FTIR analysis of skin surface (8 scans) [15, 32]. SC at the treated site was progressively removed by repeated adhesive tape stripping (time 5 seconds). Adhesive layer of the tape was applied uniformly with the same low pressure for each strip by the same trained operator. The adhesive tape carefully adhered to the disc surface (1.54 cm2) covering the treated surface, and the application pressure was uniform on this small area completed by a constant velocity for removal (duration 5 seconds).
Porcine Skin Decontamination
Decontamination methods were performed in the same conditions as those used in firefighter processes and five different decontamination methods were investigated at room temperature (25 °C) and compared with each other. In method I, FE powder (0.16 g.cm−2) was applied onto skin explant contaminated with 4-CP (n = 30) for 1 min and then gently removed from the skin surface by using absorbent paper (Precision Wipes Kimtech Science, Surrey, UK). In method II, FE powder (0.03 g.cm−2) was stuck onto adhesive tapes. FE loaded adhesive tapes were applied for fifteen seconds and then stripped from the contaminated skin explant surface (n = 30). In method III, FE powder (0.02 g.cm−2) was stuck onto the palmar face of cotton wash gloves (14 × 21 cm; GT-1000, LCH medical products, Paris, France) by using glue spray (BI2L, Villeneuve-d’Ascq, France). FE powder loaded on the palmar face of a glove was applied to a contaminated skin explant (n = 30) for 30 seconds; after that skin surface was cleaned with the posterior face of the glove. In method IV, FE suspension was prepared by dispersing 10 g of FE in purified water (100 mL) and was applied (0.65 g.cm−2) onto 4-CP-contaminated skin explants (n = 30) for 1 min, and then skin surface was gently dry-wiped. In method V, water decontamination by the rinsing of contaminated skin explants (1.54 cm2; n = 30) was performed with 3 mL of demineralized water for 15 seconds (23 mL.cm−2.min−1) using pipette Pasteur (Fontenay-sous-Bois, France).
In methods I–V, residual 4-CP content within the SC was assessed after decontamination by integration of cyanyl stretching vibration absorbance peak obtained with ATR-FTIR (8 scans), as described in the previous section.
Statistical Analysis
Statistical analysis was performed to study the effect of decontamination methods on percentage of decontamination. Percentage of decontamination distribution normality was checked by normality AGOSTINO test for the four decontamination methods. Homoscedasticity of data across the four decontamination method groups was investigated with Bartlett test. In case of heteroscedasticity (i) multiple pairwise comparisons between variances were performed with Fisher test to compare the variability of results between groups and (ii) non-parametric Kruskal-Wallis tests was used to evidence a potential effect of decontamination methods on percentage of decontamination. In the case of a significant method effect on percentage of decontaminating six pairwise comparisons between means for the four methods were conducted with the Welch test to take into account heteroscedasticity.
A significant level of 5% was selected for single comparison. The p-value was adjusted for multiple comparisons with Bonferroni method.
Statistics were performed using the R language , version 3.0.2., available on the website http://cran.r-project.org/ [17 December 2015].
Results
Results highlight adsorption capacity of the fuller’s earth for 4-CP from aqueous solutions; therefore, quantification is analysed by both kinetic studies.
Decontamination efficiency of four FE‘s methods has been performed ex vivo on contaminated porcine skin and compared with water decontamination.
Adsorption Capacity of the Fuller’s Earth

Adsorption Capacity of 1 g Fuller’s Earth for the 4-CP from Aqueous Solution


Effect of time on the 4-CP adsorption by FE for three C0 4-CP concentrations: 10 mg.L−1 (○), 100 mg.L−1 (□), 500 mg.L−1 (◊). Data is fitted with the second-order kinetic model (─)


First- and second-order kinetic models for adsorption of 4-CP from aqueous solution by FE (estimation made using KaleidaGraph 3.6)
Parameters | First-order kinetic model log(C FE − C FEt) = log C FE − k 1 t/2.303 | Second-order kinetic model t/C FEt = 1/(k 2 C FE 2) + t/C FE | ||||||
---|---|---|---|---|---|---|---|---|
C 0 | C FE (exp) | C FE (cal) | k 1(min−1) | r 2 | C FE | k 2 (min.g.mg−1) | r 2 | t plateau phase (min) |
10 | 0.0729 | 0.0722 | 0.8314 | 0.9868 | 0.0723 | 24.851 | 0.9972 | 14 |
100 | 0.5129 | 0.4884 | 0.2101 | 0.9479 | 0.5233 | 0.6106 | 0.9805 | 37 |
500 | 1.5225 | 1.4653 | 0,0779 | 0.9584 | 1.6278 | 0.0735 | 0.9637 | 58 |
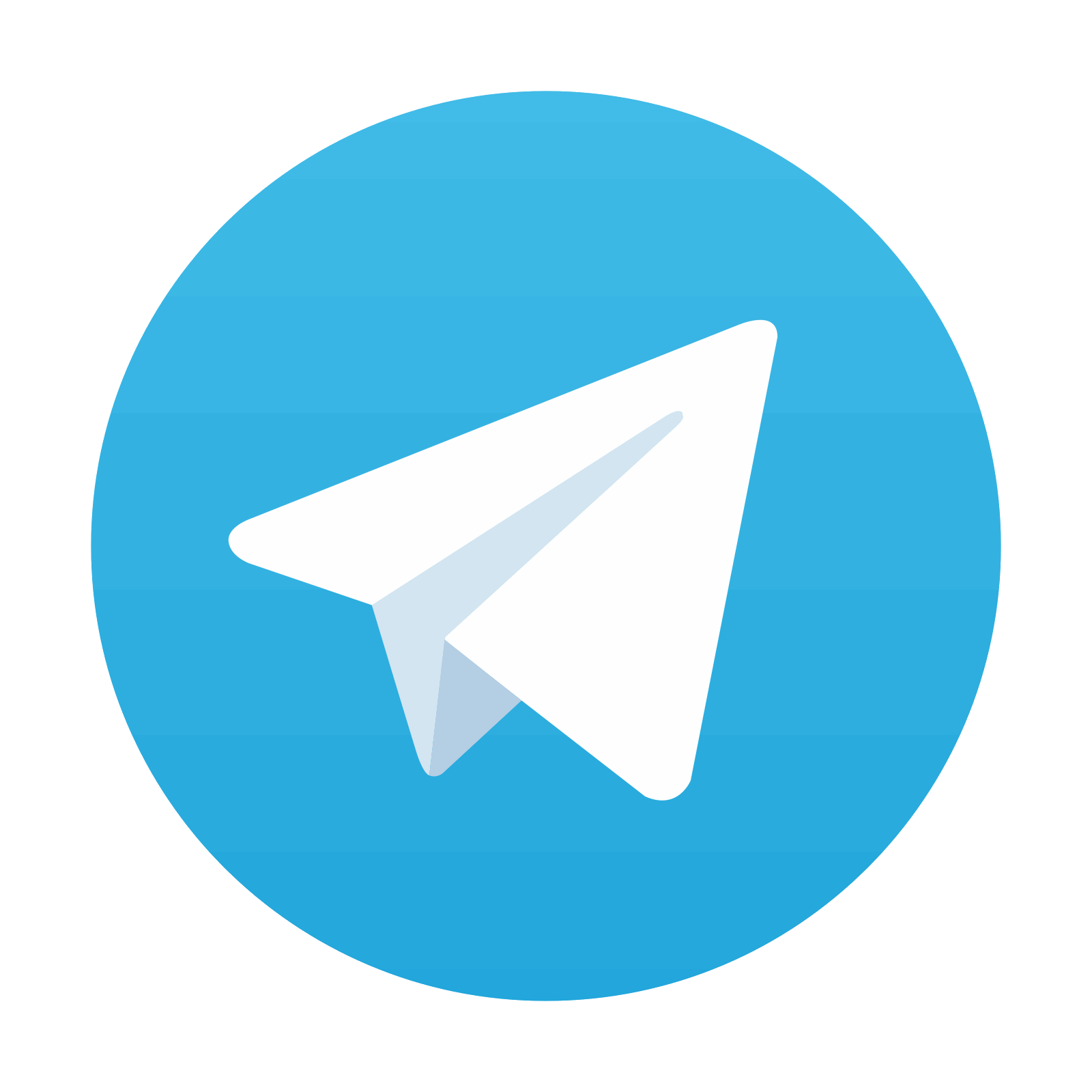
Stay updated, free articles. Join our Telegram channel

Full access? Get Clinical Tree
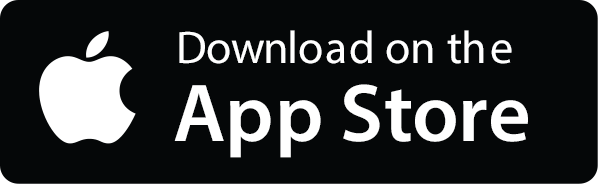
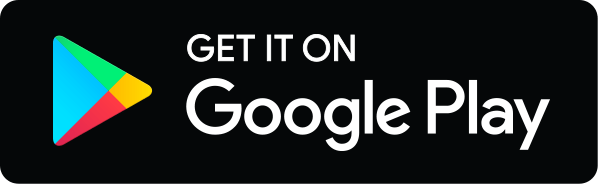