NANOEMULSIONS, LIPOSOMES, AND NANOPARTICLES
A variety of different nanomaterials exist, and each material has different properties that confer special features and benefits for their utility in cosmetics. Nanoemulsions, liposomes, and nanoparticles are detailed below. Figure 1 As the field of Delivery Systems has expanded in order to provide selected active ingredients to target layers within the skin and then deliver their payload when it will do the most good as well as delivering at a rate that typically slows down the transfer, nano-based materials have emerged as a viable modality.
Figure 1: Nanomaterials. Adapted from Zhang et al.108
Lipid-based nanoparticle delivery systems, including liposomes, nanoemulsions, and solid lipid nanoparticles, are becoming more widespread in study and use because they are made of well-defined and biocompatible molecules, are simple to characterize, and can be synthesized for different methods of delivery. These modalities are particularly useful for the delivery of oily, hydrophobic actives, making them advantageous for use in cosmetics and pharmaceuticals.7 Oily compounds are often included in topical formulations for the purpose of protecting the skin and improving its inherent barrier function. The delivery vehicle must be a well-tolerated system, and given the biocompatible and nontoxic nature of lipids, they can be easily utilized in nano-approaches.7
Nanoemulsions are similar to traditional emulsions in that both are composed of water and oil droplets that have the tendency to break into their constituent ingredients upon topical application to the hair or skin.3 Of course, high-shear forces typical of those imparted between hands and skin, with almost no gap between them, are significant to their impact on the stability of the interfacial barriers between the hydrophilic and hydrophobic components. Nanoemulsions are typically comprised of a combination of traditional cosmetic ingredients including water, oils, and surfactants in a two-phase or multiple-phase emulsion system. These systems contain small droplets with diameters of 50 to 100 nm dispersed in an external phase.3 The presence of ultra-small droplets confer special properties to nanoemulsions, including transparency and a pleasant texture and sensory experience when applied topically.5 Nanoemulsions are beneficial in cosmetic applications due to their rapid penetration past existing barriers and for having suitable textures as well as biophysical properties such as hydration capability.8 A wide variety of cosmetic products have been synthesized utilizing nanoemulsions: lotions, milks, and clear gels, all with slightly varying, and controllable, physical behavior.8
Liposomes and niosomes are globular vesicles whose diameters range from 25 to 5,000 nm. These nanomaterials are composed of amphiphilic molecules that associate into a double layer or multiple double layers, forming unilamellar and multilamellar vesicles, respectively.3 While liposomes are primarily composed of phospholipids, niosomes are mostly generated from nonionic surfactants, such as alkyl ethers or esters.9 Both can incorporate hydrophilic or lipophilic substances within them. The structure of liposomes is analogous to that of mammalian milk, which contains milk fat globular membrane surrounding nano-sized fat droplets.10,11 When applied topically, liposomes and niosomes tend to break into their individual components, similar to nanoemulsions.3,5 These vesicles play an important role in cosmetic applications because they improve the stability and tolerance of the encapsulated active ingredients. They are also advantageous in that they are well tolerated and can be easily produced on a large scale.12,13
Nanoparticles are defined as single particles with at least one dimension less than 100 nm, although their agglomerates may be larger in size.3 There are several subtypes of nanoparticles, which are described below.
Polymeric Nanoparticles
Depending on their composition and molecular structure, polymeric nanoparticles can be classified as nanocapsules or nanospheres. Nanocapsules are vesicular structures that function as carriers for lipophilic materials that can then effectively penetrate the skin via slow absorption into the stratum corneum.14 In contrast, nanospheres are matricial structures, in which the active ingredient is encapsulated during the synthetic polymerization process. These nanoparticle systems can enhance active component penetration of the skin barrier, which would otherwise demonstrate inadequate penetration in their unencapsulated form.15 Nanoencapsulation can also increase the bioavailability16 and the stability of the topically applied compounds.17,18 Moreover, nanoencapsulation can protect the substances from degradation in storage, as well as photodegradation.14
Solid Lipid Nanoparticles
Solid lipid nanoparticles (SLN) were developed in the 1990s as the first generation of lipid nanoparticles 19 and served as an alternative carrier system to other nanomaterials such as emulsions, liposomes, and polymeric nanoparticles already being utilized.12 SLN are synthesized by replacing the liquid oil in an oil-in-water emulsion with a lipid that is solid at both room and body temperature.12 SLN have several advantages as carriers for active ingredients as compared to emulsions and liposomes. Firstly, encapsulating the active ingredient within the matrix of the SLN shields the ingredient from degradation in solution. In addition to this protective aspect, the solid matrix of the SLN can be modified to control the rate of release of the active compound.20 Active compounds can be incorporated homogenously throughout the SLN matrix, concentrated into a compound-enriched outer shell, or integrated into the core of the SLN.20 Formulation parameters and compound concentrations can be varied to yield different release profiles from SLN.20 Cosmetic ingredients and active pharmaceutical ingredients (APIs) can subsequently be incorporated into these carriers, providing the potential for a wide variety of topical applications.19
SLN exhibit properties that are advantageous for dermal application: they are colloidal carriers that permit controlled release of many active substances, as well as enabling enhanced tissue distribution and targeting.21 In addition, they have low toxicity profiles and are well tolerated. Because SLN possess occlusive properties, they also increase skin hydration when applied cutaneously. These lipid nanoparticle carriers also increase the stability of active compounds that are sensitive to light, oxidation, or hydrolysis. Additionally, SLN stability in body fluids can be amplified by modifying their surface with hydrophilic molecules of varying length such as polyethylene glycol (PEG).21 This hydrophilic coating decreases phagocytic uptake of the nanoparticles while minimizing unintended interactions with other proteins.
After SLN synthesis, component lipids partially crystallize in high-energy modifications. During storage, however, these modifications can transform to the low-energy, more highly ordered crystal lattice form.12,22 The high degree of order in these crystals decreases the number of imperfections in the crystal lattice and results in an increased expulsion of the active ingredient. The potential for SLN formation of a perfect crystal lattice has been compared to the structure of a brick wall comprised of unbroken bricks of one size. Such a structure has no imperfections and little space to accommodate added ingredients. We contrast this tight, uniform structure to the image of a wall made of stones of different type, size, and shape. Such a wall, or crystal, is less ordered and has more space within itself to accommodate active ingredients. In other words, a less-ordered crystalline lattice contains more imperfections and therefore a higher drug or active ingredient loading capacity.12 Thus, despite the advantages of SLN, their tendency to form a highly structured lattice precludes higher maximal incorporation of the active ingredient.
Nanostructured Lipid Carriers
In light of the limitations of SLN, a second generation of nanoparticle carriers, nanostructured lipid carriers (NLC), was developed in an effort to better encapsulate and increase the load capability of active ingredients as well as to prevent release during storage.21 These carriers differ from SLN because rather than using solely a solid lipid component, they contain blends of both solid and liquid lipids at varying ratios.22 By using a combination of solid and liquid lipid components in NLC, the resultant lipid matrix is less highly ordered, has more imperfections, and thus enables greater loading of active nanoparticles.
Three types of NLC have been developed: imperfect type, amorphous type, and multiple type.13 Imperfect type NLC have an imperfectly ordered solid matrix achieved by blending structurally different liquid and solid lipids. This synthetic method leaves large distances within the lipid matrix that can accommodate encapsulation of active ingredients in random clusters.21 Amorphous type NLC are synthesized by combining solid lipids with special lipids (e.g., hydroxyoctacosanylhydroxystearate, isopropyl myristate, or medium-chain triglycerides). This combination yields a noncrystalline lipid matrix.23 Multiple type NLC are synthesized with nano-solids and oil components in a water carrier. These NLC can be utilized when the solubility of an active ingredient is not adequate for achieving the desired concentration.21 Adding a higher fraction of liquid lipid will modify the solid matrix to allow for greater solubility of active ingredient in the lipid regions.24 These liquid regions, known as oily nanocompartments, prevent expulsion of the active ingredient, while maintaining the solid matrix structure of the NLC.24
Both SLN and NLC are appropriate for use on inflamed or damaged skin because they are composed of non-irritant and nontoxic lipids.25,26 SLN and NLC have been investigated using a variety of encapsulated compounds, including Vitamin E,20 tocopherol acetate,27 retinol,15 and ascorbyl palmitate.28 Yet, evidence suggests that NLC are advantageous over SLN in that they have a higher loading capacity for active ingredients and lower expulsion rate of the active ingredient during storage,29 providing increased utility for topical administration. NLC are also superior to polymeric nanoparticles because of their low toxicity, biodegradability, controlled release, and avoidance of organic solvent use during production.19 SLN and NLC can achieve successful encapsulation of active compounds within their structure and offer an economical and simple administration route for patients.
10.1.3 NANOTECHNOLOGY AND PHOTOPROTECTION
Excessive skin exposure to ultraviolet (UV) radiation causes a variety of adverse effects including sunburn, dry skin, photo-aging, wrinkles, photo-immunosuppression, and photo-carcinogenesis.6,30 Protective clothing such as long-sleeved clothing and hats to shield the face are one way to decrease sun exposure. However, sunscreen remains the most widely used form of photoprotection.6 The degree of photoprotection offered by a particular sunscreen depends on its sun protection factor (SPF), which corresponds to the duration of time for which the sunscreen will prevent reddening of the skin.30 Despite their benefits, molecular sunscreens can penetrate the skin and can cause photo-allergies, skin irritation, and phototoxic reactions.31
Titanium dioxide (TiO2) and zinc oxide (ZnO) are two of the most common inorganic UV filters utilized in sunscreens.2,32 These inorganic sunscreens are effective filters of UVA and UVB light2 and have long been considered safe and effective methods of photoprotection because of their photostability and low photo-allergic potential.32,33 Yet historically, their use was limited because of poor aesthetics: the large size of the TiO2 and ZnO particles caused a white film to remain on the skin after application.2,6 Additionally, these inorganic particles did not disperse well in their carriers and left a grainy feeling on the skin when applied.6 This resulted in decreased consumer desire to utilize these products.
Sunscreen safety can be improved by means of employing carriers that possess sunblocking characteristics themselves, such as SLN.31 These decrease the concentration of molecular UV blockers required in the sunscreen formulation, provided that these carriers have a beneficial safety profile. SLN do in fact demonstrate sunblocking activity by scattering UV light. This intrinsic sunblocking activity is thought to correlate with the degree of crystallinity of the lipid matrix. Therefore, sunscreens can be formulated with a decreased quantity of molecular sunblocking agents combined with these crystalline lipid matrices.31 An in vitro study evaluated the efficacy of a highly crystallized nanoparticle carrier for benzophenone-3, a liquid lipophilic molecular sunscreen. When compared to a standard sunscreen emulsion, the crystalline lipid nanoparticles more strongly scattered incoming UV rays; when benzophenone-3 was incorporated into these nanoparticles, there was a synergistic effect on photoprotection.31 More importantly, when incorporated into nanoparticles, the concentration of benzophenone-3 could be reduced by 50% and still maintain the same degree of photoprotection as the reference formulation. This study demonstrated that highly crystallized lipid nanoparticles are effective carriers for molecular sunblocking agents (as opposed to particulate agents) and that they are capable of conferring increased photoprotection.31
TiO2 and ZnO insoluble nanoparticles were incorporated into sunscreens in an effort to reduce the disadvantages traditionally associated with these inorganic compounds and thus increase effectiveness and consumer use. Nano-sized TiO2 and ZnO enabled sunscreens to be transparent upon skin application, resulting in increased consumer acceptance and use.5 Nano-sized TiO2 and ZnO were first patented in the 1980s; the United States Food and Drug Administration (FDA) approved nanoparticle use in sunscreens in 1999.2 Today, one of the largest applications of nanoparticles is in sunscreen products.3,5 The proposed benefits include not only improved aesthetics, but also superior UV protection6,30 when compared to micron-sized particles.34 Their enhanced UV blockage properties are thought to be due to their ability to scatter light efficiently.30
TiO2 and ZnO nanoparticles exist in three states: the primary nanoparticles, aggregates of these nanoparticles, and agglomerates. The first stage in manufacturing requires the production of the TiO2 and ZnO primary nanoparticles, which range in size from 5 to 20 nm. These primary particles cluster to form aggregates ranging from 30 to 150 nm in size. The driving force for the clustering is similar to that seen in macroscopic aggregation of larger particulates. The aggregates are the smallest units that are present in sunscreens. However, as a result of the drying and heating process during sunscreen formulation, aggregates clump into loosely bound agglomerates with a size greater than 1 micron.
The degree of UV absorption by inorganic nanoparticles such as TiO2 and ZnO depends on the size of the aggregates3,6; SPF will vary significantly if different sunscreen formulations are made with varying size of inorganic nanoparticles, even if the concentration of those particles is the same.6 Large agglomerates of TiO2 and ZnO nanoparticles are not effective UV attenuators and therefore must be broken back down into nanoparticle aggregates and maintained in this form in the final sunscreen formulation. To do this, inert coating materials such as aluminum oxide or organosilicone oils (polydimethyl siloxane) and other variations of silicone polymers and copolymers are added to the sunscreen to maintain small aggregate size, prevent agglomerate reformation, and improve nanoparticle dispersion.3,5,6 This process ensures that the targeted SPF will be maintained in the final sunscreen. In contrast, organic UV filters absorb UV light as a function of their concentration; therefore, different concentrations of such filters will confer different SPFs.6 Regulations around the world vary as to the acceptability of organic versus inorganic UV filters.
Despite the cosmetic benefits of nano-sized TiO2 and ZnO in sunscreens, which include dispersal in a less viscous vehicle, increased transparency, and skin blending,2 safety concerns have emerged. While particle size is fundamental to their utilization, size is also the cause for concern regarding their potential toxicity,4 including the potential for reactive oxygen species (ROS) generation upon UV exposure,2,6 percutaneous penetration of the particles,6 their ability to evade the host’s immune defense mechanisms and complex with host proteins, and the potential respiratory tract consequences resulting from inhalation.2
Overall, both TiO2 and ZnO have an excellent safety record and have been utilized for decades in a variety of consumer products, including skim milk, cottage cheese, lotion, toothpaste, baby powders, and shampoo. However, because TiO2 and ZnO are known photocatalysts and emit electrons upon UV exposure, there has been concern about the generation of free radicals and oxidative damage to living cells.2 Several in vitro studies have suggested that TiO2 and ZnO generate ROS that cause subsequent DNA damage as a result of UV exposure. However, there are limitations to these studies. For example, one study did not confirm particle size in the sunscreen; therefore it is unclear whether they truly were nanoparticles in the formulation.35
Another study failed to describe its methodology and therefore the results cannot be appropriately analyzed or reproduced.36 A commonly cited study by nanotechnology proponents refutes the potential dangers of nano-sized TiO2 and ZnO. Investigators applied nano-sized ZnO in the dark, either under simultaneous irradiation with UV light, or to cells pre-irradiated with UV light. Pre-irradiated cells later treated with ZnO and cells given concurrent ZnO and irradiation both demonstrated the same type and incidence of chromosomal aberrations. Researchers therefore concluded that ZnO is not phototoxigenic, and that the DNA damage resulted from UV-mediated enhanced susceptibility to ZnO.37 Regardless, in response to the many in vitro studies demonstrating ROS generation, manufacturers began to coat the surface of these nanoparticles to decrease free radical generation upon UV exposure.
Oxidative damage due to TiO2 and ZnO exposure to UV radiation should only be considered a valid concern if the nanoparticles are proven to penetrate the epidermis.2 In healthy human skin, nanoparticulate TiO2 or ZnO do not penetrate further than the upper layers of the stratum corneum.2,38-44 The stratum corneum, the outermost layer of the epidermis, consists of nonviable keratinocytes and represents the rate-limiting barrier against absorption and percutaneous penetration of materials applied topically.5 Figure 2 The barrier protection provided by the stratum corneum is thought to prevent deeper penetration of nanoparticles and inhibit their migration to living epidermal and dermal cells. (See Chapter 1 for a detailed description of the skin structure.)
Figure 2: Structure of the skin.
Although nanoparticles do deposit within the stratum corneum, the continuous shedding of this epidermal layer prevents deeper nanoparticle penetration into the epidermis. However, nanoparticles have been shown to penetrate the skin via the follicular ostia. TiO2 nanoparticles have been detected within hair follicles after topical application of sunscreen; however, this does not represent nanoparticle penetration into living skin layers.39 Furthermore, sebum flow from the pilosebaceous glands and follicular epithelium turnover effectively eliminate insoluble particles collected in follicular orifices.3 Thus, despite the safety concerns regarding the toxic effects demonstrated by TiO2 and ZnO nanoparticles on living cells, available evidence demonstrates that when applied to healthy, intact skin, TiO2 and ZnO nanoparticles do not have the capacity to penetrate living skin cells; therefore the risk of ROS generation and subsequent DNA damage is minimal.2 However, more research is warranted to examine the effect of nanoparticle application to damaged skin, as no conclusive evidence exists in the literature.2,6
While it may be intuitive to assume that compromised skin has increased susceptibility to penetration by small particles, this may not be the case. In fact, skin inflammation often produces epidermal thickening, which may in turn enhance the barrier function of the skin, rather than reduce it.5,45 For example, psoriasis results in hyperkeratosis, which can decrease penetration of topically applied substances. In contrast, eczema and similar cutaneous pathology involves breaks in the stratum corneum, effectively reducing the barrier function of the epidermis and thereby permitting increased penetration of topical treatments.3 Similarly, sunburned skin slightly enhances TiO2 and ZnO nanoparticle penetration in sunscreen formulations in in vitro and in vivo models, but transdermal absorption was not detected.46
Nanoparticle carriers have also been employed for encapsulating sunscreen agents to improve efficacy and minimize side effects. Examples of organic UV filters include avobenzone and oxybenzone.6 Oxybenzone is an aromatic ketone that provides broad-spectrum UV coverage and was first approved by the FDA in the early 1980s.33 Despite its effectiveness as a sunscreen agent, it is the most common cause of photoallergic contact dermatitis19 and systemic absorption of oxybenzone following its topical application has been reported.47 Sanad et al. developed NLCs of oxybenzone to enhance its photoprotective efficacy as well as safety.48 The synergistic effect of NLCs’ and oxybenzone’s UV blocking power is sixfold more efficient in protecting against UV radiation, while simultaneously minimizing skin irritancy traditionally seen in oxybenzone.48 Today, it is one of the most widely used organic UV filters used in sunscreens produced in the United States.33
10.1.4 NANOTECHNOLOGY AND HAIR CARE
Hair covers much of the skin’s surface area, with the exception of glabrous zones such as the palms, soles, and vermilion border of the lips. Appendageal hairs consist of pilosebaceous apparati coupled to either terminal or vellus hairs. The abundance of human hair lends it to being a focus of many cosmetic enhancements and treatments.
Hair care consumer products are designed for maintenance of hair health, cosmetic enhancement, or alleviation of hair damage or disease. Hair health maintenance entails preservation of hair color, texture, shape, or shine. Cosmetic enhancements include hair coloring, styling, and volumizing, as well as growth of missing hair or removal of unwanted hair. Mitigation of hair damage may be accomplished via strengthening, lengthening, or volumizing hair. Hair and scalp disease may be alleviated with topical agents. This subject has been discussed in detail elsewhere in this book.
Current hair care products have some limitations including chemical content, potential irritancy, or allergenicity49-51 and potential side effects from systemic absorption.51 Hair dyes consist of a range of chemicals whose composition and duration of effect varies among dyes.49 Contact sensitization to hair dyes is a well-known safety issue and is most common from professional exposure.51 There has also been increasing awareness regarding p-phenylenediamine (PDA), a potent contact allergen found in permanent hair dyes.49,50 Delayed-type (and rarely, immediate) hypersensitivity reactions have been reported following exposure to PDA and its related compounds.49,50 When tested in murine models, PDA-containing hair dyes induced local inflammation, Th1 cell proliferation50 and increased levels of inflammatory cytokines such as interferon-gamma and interleukin (IL)-17.52 The field of nanotechnology offers unique solutions to some of these hair care challenges, as significant strides have been made in the development of new and useful hair care tools. For example, PDA-incorporated nanoparticles were less cytotoxic to human skin keratinocytes compared to PDA alone.49
Hair-coloring products generate $12 billion per year with an estimated 50% of adults using hair dyes at some point in their lifetime.49 Incorporation of nanotechnology into hair-care products has improved such products. Nanostructured liposomal emollients penetrate the hair shaft better, protect chemically unstable ingredients against degradation, and permit controlled and sustained release of the dye’s active ingredient.53 An in vitro study on porcine skin compared the degree of penetration of dye-containing nanoparticles to the penetration of the same quantity of dye in the nonparticle form. The dye-containing nanoparticles demonstrated much deeper penetration into hair follicles, especially if massage was applied. However, without mechanical manipulation of the skin surface, there were no differences in penetration between the two groups.54 It is thought that hair shaft movement induced by massage acts as a pumping mechanism to facilitate particle translocation into the hair follicle. Figure 3. Under in vivo conditions, this presumed pump mechanism is thought to occur without massage via continuous body movement.54,55 Additionally, this study found that the nanoparticles remained stored in the hair follicle for a much longer period of time compared to the non-nanoparticle dye.
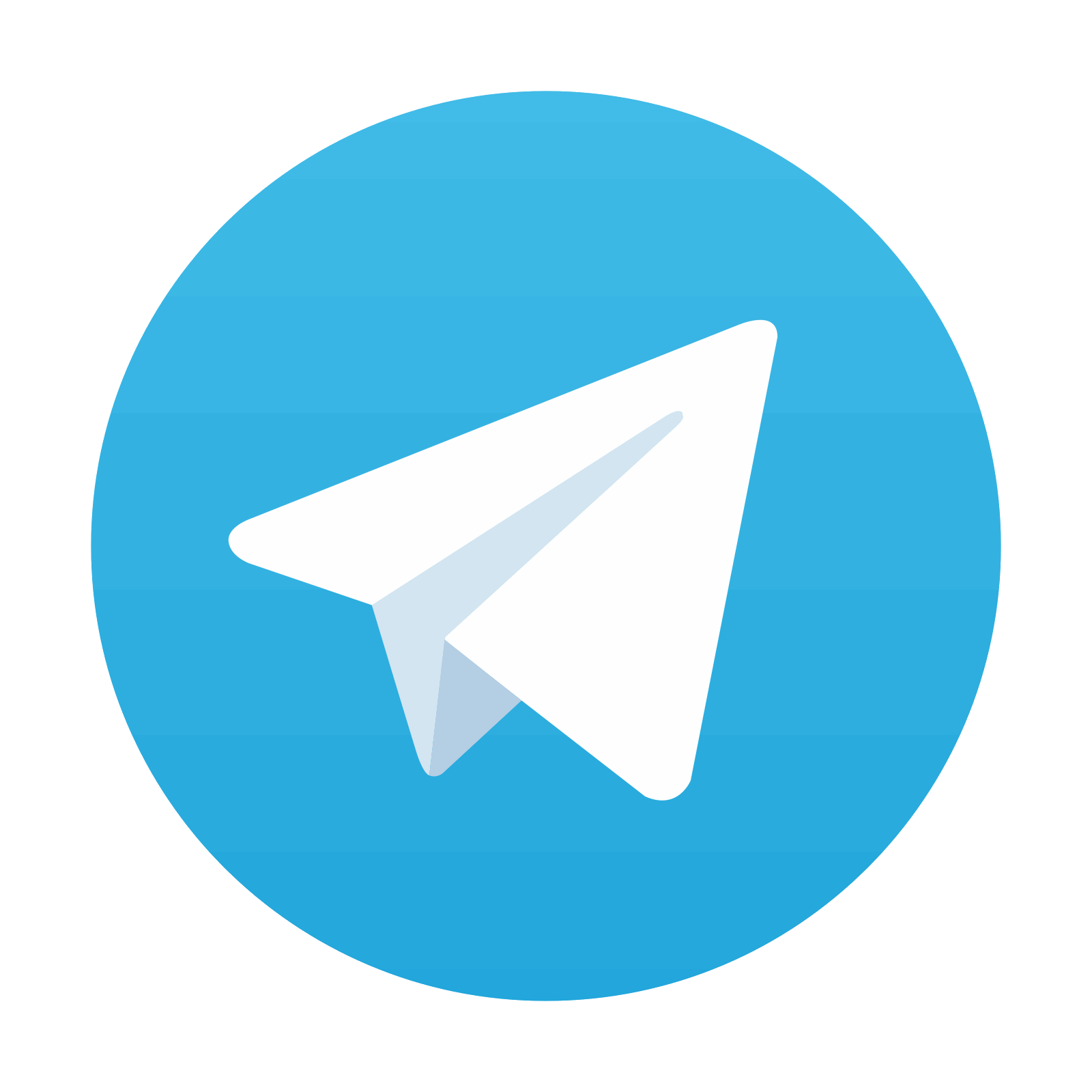
Stay updated, free articles. Join our Telegram channel

Full access? Get Clinical Tree
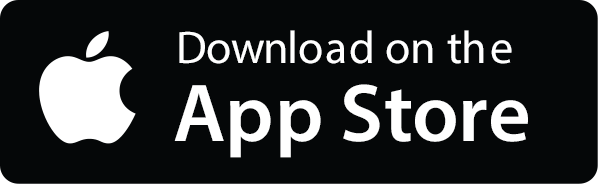
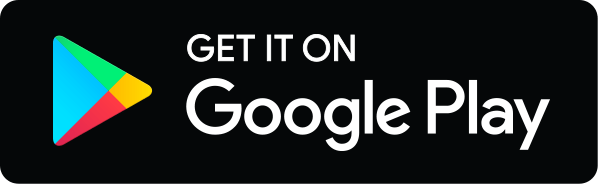