Microneedling has evolved over recent decades and has multiple uses in dermatology.
Complete understanding of microneedling’s mechanism of action is unknown. There are two leading hypotheses: (1) fractionated mechanical microinjury with classical wound healing and (2) demarcation current during which shifts in transepithelial electrical potential leads to cellular migration, proliferation, and remodeling. Stimulation of growth factors and DNA synthesis pathways plays a crucial role in the downstream effects.
There are three main device types: stamps, rollers, and pens. Each has advantages and disadvantages. Needles vary as well.
Providers should understand which systems to use in different clinician scenarios and how to tailor treatments to anatomic location and cutaneous condition.
5.1 Introduction
An increasingly popular, minimally invasive procedure, microneedling combines the ancient principles of acupuncture and mesotherapy with more modern technologies to treat a range of cutaneous conditions. In the 1990s, Orentreich and Orentreich first reported “subcision” involving the use of a needle inserted parallel to the skin’s surface to manually disrupt scars, a procedure best suited for small treatment areas given the risk of bruising. 1 Camirand and Doucet then treated scars using a tattoo gun to break dermally tethered collagen bands, but this technique was labor intensive and slow with little control over the depth of penetration. 2 Fernandes subsequently built upon these principles in his seminal paper from 2005 and coined the term “percutaneous collagen induction” (PCI) therapy, referring to rejuvenation and skin remodeling with tiny needles mounted on a cylindrical roller (also known as dermaroller). 3 Since this time, the technique has undergone several iterations with its avatars including collagen induction therapy, dermal needling, dry tattooing, intra-dermabrasion, and microneedling.
5.2 Mechanism(s) of Action
Microneedling involves the production of transient epidermal and dermal pores ranging from 25 to 3,000 µm in depth with the fundamental goal of upregulating normal skin synthesis mechanisms and not inducing prolonged inflammation or trauma that may lead to fibrosis. There are two major theories explaining how needles induce tissue remodeling and proliferation.
5.2.1 Fractionated Mechanical Microinjury
The first explanation involves what the authors like to refer to as fractionated mechanical microinjury ( ▶ Fig. 5.1). Needling forms tightly packed, minute perforations. The resultant near-confluent dermal and superficial capillary disruption provides a strong stimulus for growth factor release and fibroblast infiltration. 4, 5, 6, 7
Fig. 5.1 Fractional mechanical microinjury hypothesis for the mechanism of action in microneedling. Tiny, superficial wounds form a strong stimulus for growth factor release and fibroblast infiltration following the classical phases of healing.
Microchannels heal following the three classic phases of wound healing: inflammatory, proliferation, and remodeling. In reality, these phases overlap and a full discussion of the process is beyond the scope of this chapter. However, briefly, the inflammatory phase begins when disruption of the stratum corneum, endothelial lining, and subendothelial matrix recruits platelets and neutrophils to the site of injury. Needling expose thrombin and collagen fragments, which attract and activate platelets locally within minutes. The platelets, in turn, form a plug, initiate clotting, and release a myriad of growth factors and cytokines ( ▶ Table 5.1). These signaling molecules control clot formation, increase vascular permeability, and attract leukocytes and fibroblasts to the site of injury. In the first 48 hours, neutrophils predominate; however, it is the subsequent influx of macrophages that is critical not only for phagocytosis but also to perpetuate signaling cascades that induces cell migration and division—without which healing fails to occur. 3, 7
Molecule | Abbreviation | Functions |
Fibroblast growth factor | FGF |
|
Platelet-derived growth factor | PDGF |
|
Transforming growth factor alpha | TGF-α |
|
Transforming growth factor beta | TGF-β |
TGF-β1 and TGF-β2 are profibrotic while TGF-β3 is antifibrotic |
Epidermal growth factor | EGF |
|
Insulin-like growth factor 1 | IGF-1 |
|
Vascular endothelial growth factor | VEGF |
|
Connective tissue growth factor | CTGF |
|
Keratinocyte growth factor | KGF |
|
Connective tissue activating peptide III |
| |
Neutrophil activating peptide-2 |
| |
Interleukin 1 | IL-1 |
|
Interleukin 10 | IL-10 |
|
Abbreviation: MMP, matrix metalloproteinases. Note: This table is intended as an overview and is not exhaustive. 3, 6, 8, 9, 10, 11 |
This also marks a transition to the proliferative phase of wound healing, during which growth factors derived from macrophages (i.e., PDGF [platelet-derived growth factor], TGF-α [transforming growth factor] and TGF-β, interleukin-1, tumor necrosis factor, FGF [fibroblast growth factor]) recruit fibroblasts and alter extracellular matrix synthesis. Fibroblasts migrating along a fibronectin scaffold allow for fibroplasia and granulation of the wound. Typically in healing of larger wounds, keratinocytes change their phenotype and migrate to repair tears in the basement membrane. Desmosomes dissolve and actin forms to knit keratinocytes together. The small injuries created by microneedles disrupt the basement membrane and expose keratinocytes to dermal collagen, thereby stimulating multiplication. However, these defects are miniscule and close rapidly—within hours—so the proliferating keratinocytes serve more to thicken the epidermis rather than close the needling channels. 3, 7, 12
At the same time, relative tissue hypoxia is another cue that triggers cytokine release, in response to which fibroblasts produce PDGF, FGF, and EGF. There is an upregulation of procollagen mRNA, which later converts to collagen III in the presence of oxygen supplied by new vessels. 3, 6 VEGF and other growth factors are key in the angiogenesis process. 6
The remodeling begins in days with the formation of the fibronectin matrix but takes months to years for full execution. Hence, much of the improvement in skin quality seen after microneedling appears after three to four weeks and continues for months. 13 Collagen type I replaces III, which replaces the early granulation tissue laid down in the upper dermis. Interestingly, this collagen integrates a regular basket-weave lattice rather than in the parallel bundles seen in scars. 13, 14, 15, 16 Assays from needled skin in rats suggest that disproportionately high levels of TGF-β3, an antifibrotic cytokine, may account for the scarless healing seen with microneedling. 8 Vitamin A or retinoic acid favors the production of TGF-β3 over its profibrotic family members TGF-β1 and TGF-β2 and explains why some providers combine topical retinyl esters in their protocol. 8 Some investigators assert that matrix metalloproteinases may also play a crucial role in decreasing the fibrosis and perhaps minimizing hyperpigmentation, 13 yet these mechanisms have not been elucidated fully.
5.2.2 Demarcation Current
Leibl proposed an alternative explanation for the cutaneous effects status post microneedling—shifts in transepithelial potentials called “demarcation current” ( ▶ Fig. 5.2).’ 13 In the resting state, the intracellular potential of epidermal cells is negative (approximately –70 mV), while the extracellular potential is positive. Injury releases intracellular potassium and proteins into the extracellular compartment, further decreasing the negative potential of the intracellular compartment to as low as –120 mV, thereby increasing the potential difference between the interior and exterior of the cell. When this process occurs repeatedly in close proximity, the generated bioelectrical current triggers growth factor cascades that elicit the migration of fibroblasts, which proliferate and produce collagen. 13, 17 While deep skin cuts heal via the classic wound healing mechanisms briefly outlined above, superficial injuries produce only minor inflammation and are more likely to interfere with the intracellular electric fields to alter DNA expression and enhance cell motility. Ultimately, the hemostatic, inflammatory, proliferative, and remodeling phases of wound healing are circumvented or curtailed, so repair occurs sans scar formation. 13 This hypothesis may explain why some studies find better results with repeat superficial treatments (1 mm depth) compared to deeper, aggressive needling (3 mm), although that has been explored fully. 8
Fig. 5.2 Demarcation current hypothesis for the mechanism of action in microneedling. En masse changes in cell membrane potential create bioelectrical current that changes DNA synthesis and growth factor production.
(Adapted from Liebl H and Kloth LC. Skin cell proliferation stimulated by microneedles. J Am Coll Clin Wound Spec 2012;4:2-6.)
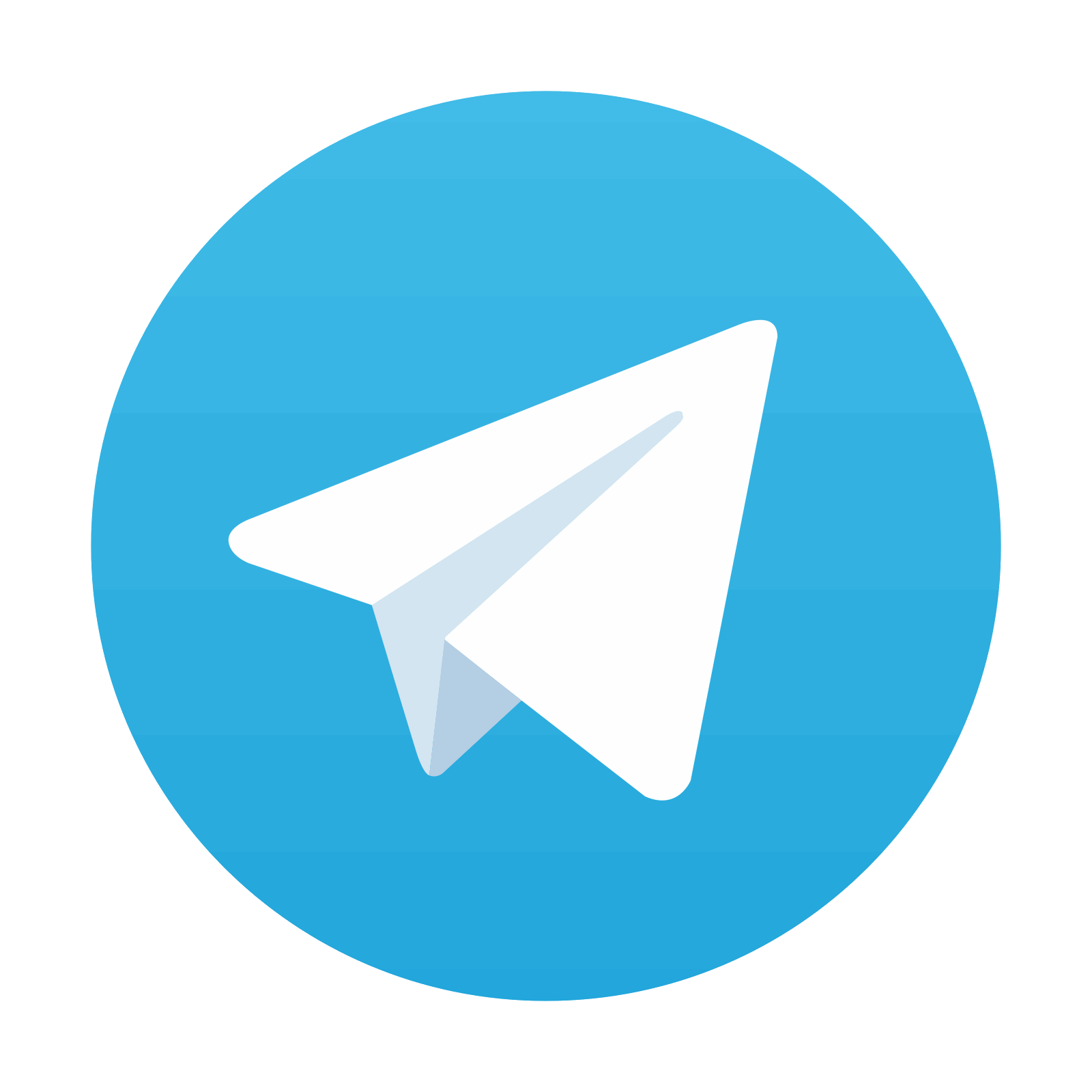
Stay updated, free articles. Join our Telegram channel

Full access? Get Clinical Tree
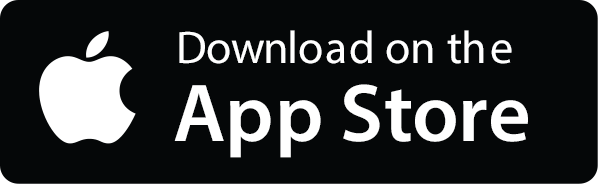
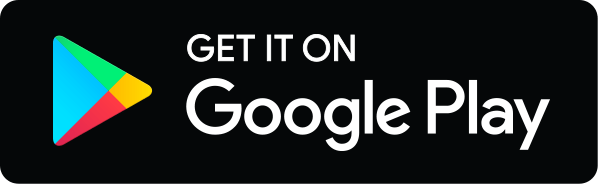