div class=”ChapterContextInformation”>
9. The Mass Decontamination Paradigm: Response Relating to Gas Phase Exposures and Skin Decontamination
Keywords
HAZMATGasVaporIn vitroSulfuryl fluorideHydrogen cyanideThe Problem
The accidental or intentional releases of toxic gases or vapors1 are the most common occurrence in hazardous material (HAZMAT) incidents that result in human injuries [1, 2]. Such atmospheric releases are typically associated with industrial accidents . (e.g., chlorine gas release after a train derailment in South Carolina [29]) and terrorism-related events. (e.g., Tokyo subway nerve agent sarin attack [3]). Historically, deliberate acts using hazardous materials and chemical agents have been sparse. Nonetheless, in the last few years, deliberate releases dispersing chlorine, mustard agent, and sarin in Iraq and Syria have occurred illustrating ongoing national and terrorist groups’ interest.
These releases of hazardous materials have significant consequences on public health and impose significant demands on HAZMAT response resources [4, 5]. During and following HAZMAT incidents, there are four main groups of people affected: (a) exposed and deceased, (b) exposed and symptomatic, (c) exposed and asymptomatic, and (d) unexposed and asymptomatic. Groups (a) and (b), usually acutely affected by inhalation exposure, are the most clearly recognized, with group (b) being able to be triaged and evacuated to hospital or treated at the scene. However, the onsite management of group (c) is problematic as it may be numerically large and potentially resource-intensive. Group (d), also known as the ‘worried well’, may self-present to first responders at the scene, or at nearby hospitals, or medical facilities, placing further demands on valuable resources and adding to the potential delay in identifying and treating those who need more urgent attention.
The established approach globally is, by default, to decontaminate the skin of all exposed persons using field portable shower systems. Jurisdictions have invested hundreds of millions of dollars establishing and extending their field deployable mass decontamination resources. Nonetheless, a significant incident will likely rapidly overwhelm the available field deployable HAZMAT mass decontamination capacity.
Is this approach consistent with the nature of the exposure incurred by the affected persons? Is it always appropriate for the skin interactions between the contaminant and the exposed persons? There is little if any published work investigating these interactions and their implication on the subsequent approaches that should be applied during a HAZMAT emergency. Most published works have focused on the mechanics of decontamination rather than whether decontamination is necessary, and what, if any, type and extent of decontamination should be applied before medical care. Decontamination modeling estimates show that if the number of persons undergoing decontamination can be reduced by 80%, then the available resource demand is reduced by 63% and the times required by 77% [6]. This has a significant impact on the efficiency of a HAZMAT response and saving the lives of those who were affected.
There is a limited evidence base describing skin absorption of toxic gases and vapors in HAZMAT exposure scenarios, which tend to be relatively brief compared with traditional test periods for skin absorption studies. In order to address this gap, there is a need for a testing strategy that encompasses the relevant kinetics and equilibria of skin deposition, absorption and penetration of gases and vapors, and the moderating effects of environmental factors. (e.g., temperature, humidity), clothing, and other factors. This is a complex multicompartment system, and any protocol should also take into account typical short-term exposures of less than 1-h duration and subsequent periods of off-gassing. Investigations within this period (sometimes termed as the “golden hour”) are of particular relevance to HAZMAT incidents [1].
From the first responder perspective, a number of key questions arise: (1) how much gas gets on, in, or through the skin in this first hour following contact, (2) what are the modifying influences of environmental factors and street clothing worn, and (3) what are the off-gassing rates from skin and clothing.
Relevant toxicological testing should aim to generate data and provide advice. These empirical data and advice relate only to the specific chemical exposures and are not intended to support recommendations regarding dissimilar HAZMAT chemicals for which alternative individual data must be gathered.
Nature of Gas Exposure
Compared with liquid or aerosol exposure scenarios, gas concentrations in gas-only scenarios are often low, i.e., typically less than 1% v/v. Following toxic gas exposure, it is often assumed that isolation from the source and removal of clothing is typically all that is needed to prevent further exposure or injury [1, 7, 8]. Levitin et al. [1] and Houston et al. [7] suggest removal and double-bagging of the victim’s clothing can eliminate 80% to 90% of the contaminant and minimize the risk of spreading the toxic agent to others. This percentage may depend on amount/type of clothing worn and the duration of exposure. Bagged clothing should be left at the scene and managed by emergency responders so as to minimize risk of exposure to hospital personnel [7, 8]. However, accident scene management and victim care varies throughout the world and is based primarily on personal judgment and dogma rather than on scientific evidence. Ill-informed decisions about the management of exposed individuals and groups during chemical releases have the potential to cause a significant drain on resources as well as a variety of undesired outcomes among those affected. In particular, decisions about mass decontamination following suspected or known chemical exposure should be risk-based and supported by suitable empirical evidence.
Following a review of toxic gas exposure literature, there appears to be limited specific advice for first responders regarding skin decontamination. As mentioned, the current generally accepted approach to mass-casualty decontamination is that all exposed individuals must “strip and shower”, regardless of the mechanism or likely form of exposure (liquid versus gas/vapor). This advice could be seen as inconsistent with ATSDR studies from 13 US states for 7 years, which determined that in 90% of cases, only six or fewer human casualties required care after chemical exposures [1, 9]. The Chemical Weapons Improved Response Program projects at least a 5:1 ratio of unaffected to affected casualties [10]. These “worried well” noninjured victims comprise the largest group in mass-casualty events (80–90% of survivors in most cases). These victims will wait for care at the scene, transport themselves to the closest medical facility for care, or leave the scene and not seek care. While evacuation from the area of chemical release will decrease their level of primary exposure, secondary exposure can potentially occur from the amount of gas/vapor remaining in their clothing. By the time showers can be set up, any trapped gas/vapor in victims’ clothing may have dispersed, making decontamination unnecessary. In this regard, Markel et al. [11] supports a “scoop and run” evacuation approach rather than onsite decontamination. Little has been done to investigate the degree and duration of trapped gas/vapor released from different types of street clothing. Furthermore, sunscreen and cosmetics may enhance, buffer or reduce dermal exposure or act as a chemical reservoir [12, 13]. In practice, emergency personnel need practical guidance about ongoing exposure potential and decontamination advice [4].
Nature of Gas/Vapor Interactions with Skin
There is a limited evidence base for the extent and kinetics of skin absorption in HAZMAT scenarios involving toxic gases and vapors [5, 14]. Much of the pre-planning has generally been based on knowledge of the basic physicochemical properties of the released chemical such as volatility/vapor pressure, water and oil solubility, molecular mass, density and other routine information. However, this information may do little to inform a dynamic or evolving risk assessment process under the variable environmental conditions that may exist during an incident.
It is well recognized that vapors, aerosols, and liquids deposit onto the skin from the atmosphere. If sufficient vapor is deposited onto the skin, condensation may occur and a liquid-like phase formed. A proportion of these absorbed molecules also evaporate. The molecules will either physically interact (absorption) or chemically interact (adsorption) with the surface. The transport of chemicals through the skin [17, 18] is either active (mediated transport requiring energy) or passive (diffusion requiring no energy). Often, the kinetics of skin absorption of gases/vapors (and their dissociation products) is slow, with competing processes of absorption and desorption more evident than that of liquid exposures.
The literature indicates that a variety of factors can play a significant role in the dermal absorption of chemicals. These include skin thickness and lipid content, occlusion, clothing, temperature and humidity, and skin damage/disease.
Clothing may serve to initially protect and buffer the skin from toxic chemicals, depending on the material, thickness, cover, and yarn twist. A tightly woven fabric using a high twist yarn gives the most protection against penetration and in conjunction with a thick fabric gives a high protection factor. However, significant skin uptake of chemical may occur if contaminated clothing is in contact with, or occludes, the skin. There do not seem to be any systematic studies of the chemical protection afforded by street clothing or personal skin products.
Personal products applied to the skin and hair, such as cosmetics and sunscreen , may enhance, buffer or reduce dermal exposure. They may also act as a chemical reservoir. The ability to enhance or reduce skin uptake depends primarily on whether the product is oil- or water-based [12], although some active ingredients can enhance penetration [13]. Indeed, it is only recently that the community usage of personal products has been studied for risk assessment purposes [15, 16].
Current Experimental Approaches to Gas Interaction Studies
The best models for understanding dermal exposures during CBR incidents would be human volunteer skin exposure studies, either whole body, or segmental, or limb exposures. These exposures may be authentic in that the chemicals may have been applied via surface sprays, droplets, spills, immersion, deposition, or from gas and vapor contact. They may be further modified under varying conditions of temperature and humidity, clothing coverage, occlusion, vehicle, and all other parameters that may be imagined or predicted for ‘real-life’ exposures . However, these methods cannot be applied for highly toxic materials, or for other materials at concentrations that may be toxic. Individuals are highly variable in their absorption, and the small number of trials that may be performed limits statistical interpretation. Also, the measures that indicate the extent of absorption may be limited to blood concentrations , urine concentrations or other such estimates that, being markers of systemic absorption, may not fully inform investigators about localized concentrations and effects. However, tape-stripping applications , in which the stratum corneum may be removed by successive applications and removal of adhesive tape, have been performed to identify the localizations of dermally applied chemicals in the stratum corneum or to demonstrate altered rates of penetration through skin in the absence of stratum corneum. Recently, confocal Raman spectroscopy has been used to examine chemical concentrations in skin strata [17]. Such approaches, however, have not been nor could easily be applied to gas or vapor exposures.
Whole animal (in vivo) models may suffer from poor extrapolation to human circumstances because of significant interspecies variation. These are also relatively expensive, especially in large, statistically justified investigations. Ex-vivo systems , including the isolated perfused pig ear, have also been used to examine the uptake of chemicals through a system whose blood circulation (through arteries, arterioles, capillaries, venules, and veins) remains intact.
In vitro models , using either animal- or human-derived tissue, remain the most common methods used for a wide range of assessments of dermal absorption of chemicals. The Organization of Economic Cooperation and Development (OECD) approach has gained widespread acceptance and adoption for in vitro testing for dermal absorption (OECD 2004) [18]. While these models suffer from the handicap of being remote from real-life situations (with consequent reduction in direct validity to human exposure scenarios), they are reliable, generally reproducible, and allow at least the rank comparison of different chemicals under similar exposure parameters. At best, they can provide quantitative comparisons, enabling some numeral risk assessments to be extrapolated.
Rat dorsum, pig ear, human cadaver skin, or viable human skin (commonly obtained from cosmetic reduction surgery) may be used in chambers with an upper application well and a lower receptor well, containing a receptor fluid to mimic cutaneous blood . This may be a simple static diffusion type (such as the Franz cell) or a dynamic, or flow-through cell. The static-type cell requires any media removed from the lower receptor chamber to be replaced at each sampling time point. The flow-through cell has a reservoir of fresh receptor fluid that is pumped through the receptor chamber and collected downstream. The tissues may be full thickness (stratum corneum, epidermis and sometimes partial dermis), split thickness (stratum corneum and epidermis only), or some defined fraction of the skin layer (sometimes dermatomed to a specified standard thickness). Each of these approaches yields different results that may not be directly comparable between methodologies. Furthermore, there are currently no test cells commercially available with a modified donor chamber to allow for gas/vapor delivery to the skin surface. This methodological challenge may in part account for the limited number of published studies on skin exposure to gases. Gaskin et al. [19]) describe a bespoke static diffusion cell with a modified donor chamber to allow for gas delivery, and appear to be one of the only research group assessing dermal exposure to toxic gases and vapors [5, 14, 19, 20].
Recent work has focused on emerging technologies to produce skin substrates that are more consistent, reliable or in other ways considered advantageous for dermal absorption experiments. These include human cell line models (growing sheets of keratinocytes of uniform or near-uniform characteristics) and partial culture (such as using viable human skin, seeded with human stem cells that form a uniform upper skin surface devoid of accessory structures and flaws).
Fresh human skin, from cosmetic reduction surgery , is the most convenient and reliable substrate available, notwithstanding the variations between donor skin characteristics. It usually provides sufficient skin for several diffusion chambers to be set up, allowing manipulation of key parameters in a controlled experiment that is internally consistent and comparable. Skin is usually from breast or abdomen, reducing the extent of site-specific variations that have been identified with skin sourced from varying anatomical sites. Skin can be used in a variety of modes (full thickness, split thickness) to satisfy the needs of the experimental approach.
Dermatomed split-thickness skin is considered by some to be superior to skin split by other methods (heat or chemical treatment), but this has been seen predominantly in studies in which maintenance of metabolic capacity of skin was desirable. For simple diffusion studies, heat-treated, split-thickness skin has been found be effective and reproducible. Integrity of skin is checked by visual inspection under a dissecting microscope prior to mounting in the diffusion cell, and by transdermal resistance (with electrical probes placed on the skin surface and in the lower receptor chamber), following mounting. This is usually sufficient to establish whether the skin sample has defects or perforations that may affect results.
Tape stripping prior to mounting or following chemical exposure can allow examination of the role of the stratum corneum as a barrier or a chemical reservoir, but is methodologically challenging. Skin washing is a common contaminant removal technique (post-exposure), and is appropriate for chemicals that have low rates of dermal absorption.
Keratinocyte culture is currently unproven and may generate a model that is more similar to the stratum corneum than to the integrated structure of the skin. Similarly, the partial culture/stem cell methods have been developed predominantly for medical applications (for treatment of skin injuries such as burns) and have not been used for dermal absorption models.
Although the use of human skin represents the most robust approach, a multispecies study has suggested that pigskin can be a very useful model for in vitro percutaneous absorption studies, provided that the skin integrity is checked prior to skin exposure, and in-house electrical resistance cut-off values are adhered to.
Systematic In Vitro Approach
The approach by Gaskin et al. represents a modification of the OECD experimental protocol for skin absorption of chemicals by specifically aligning with the context of HAZMAT incidents in which exposures occur by vapor or gas for relatively brief periods [19]. It also allows examination of the influence of fabrics and other potential barriers or reservoirs on the secondary exposure and need for decontamination. Supplementary fabric off-gassing experiments may also be conducted under variable conditions. The use of the human in vitro model allows repeatable studies to gauge variation in contributions of the various environmental conditions. Observations may then be summarized and translated into a format that guides emergency personnel in public health management at HAZMAT incidents.

Block diagram of apparatus
Test Cell
Jacketed 9 mm Static Franz diffusion cells housed in a stirrer unit (PermeGear, Hellertown, PA) may be used. Cells have a diffusion-available surface area of 0.64cm2 and utilize appropriate receptor fluid. (e.g., physiological saline, pure water or 50% ethanol). A modified donor compartment of the cell has been described for flow-through gas delivery to the surface of the skin [5]. Of note, the central bar is positioned to ensure turbulence is created in the neck of the donor chamber and allows even distribution of gas flow over the surface of the skin/substrate. The side arm may be enlarged to accommodate real time monitoring of the receptor fluid, e.g., pH/ISE probe. Prepared skin epidermis may be mounted between the donor compartment and the receptor compartment, in this way acting as a barrier for the applied test chemical to permeate into the receptor fluid. Receptor fluid is kept in contact with the skin under-surface and continuously stirred with a Teflon-coated magnetic stirring bar. After the set exposure period, the Franz cell can be dismantled and the skin and complete volume of receptor fluid used for measurements of contaminant concentration in and through skin.
Substrate Preparation
Various skin substrates may be used. However, the use of human skin samples is usually preferred for in vitro studies of dermal absorption [21] as it provides data more appropriate to human in vivo conditions. Freshly excised human abdominal skin obtained from cosmetic reduction surgery is used. In this approach, epidermis is harvested from full thickness skin within 1 h of excision from the donor. Subcutaneous tissue is removed, and the epidermal layer harvested from full thickness skin by heat treatment [17, 22, 23]. Pre-exposure skin electrical impedance (EI) testing is performed to provide a rapid assessment of barrier integrity in vitro [23–25]. For this purpose, a Tinsley LCR Databridge 6401 [26] is used set in Resistance (R), Parallel Equivalent (PAR) and 100 Hz modes. Skin EI may also be assessed post-exposure to toxic gas in order to assess changes in barrier integrity resulting from exposure.
Gas Challenge
Toxic gases and vapors may be delivered directly from the source, for example, a certified gas mixture cylinder. More commonly, it is diluted to test concentrations as required using a bespoke dynamic atmosphere generator and a diluent gas stream, typically purified air [27]. For HAZMAT scenarios, challenge gas concentrations should be physiologically relevant and appropriate for first responder guidance, for example, the lowest lethal concentration (LCLo) , the concentration immediately dangerous to life and health (IDLH), or other relevant concentrations. (e.g., eye irritation). Short-term exposure times of 5, 10, 20, and 30 min are used, simulating HAZMAT incident exposures [5, 14]. A minimum of four replicates for each variable at each exposure time should be gained. For some experiments, a post-exposure open-to-atmosphere ventilation period is used to read at 60 min (i.e., 5 min exposure with 55 min ventilation, 30 min exposure with 30 min ventilation etc.). In all experiments, a method of destructive sampling is applied to make determination of contaminant concentration IN, ON and THROUGH skin possible.
Experimental variables such as temperature and relative humidity may be accommodated using auxiliary components [27]. For elevated temperatures, electrical heating tape wrapped around Teflon tubing can be used in order to maintain an injected gas stream temperature of 30 °C to 60 °C. The system minimizes thermal losses by the use of foam insulation around the Teflon tubing. The heating tape temperature is controlled by a simmerstat or voltage regulator. For lower temperatures of 10–20 °C, a refrigeration unit circulates chilled water around jacketed glass fittings (for the gas stream and also the cell). Humidification of the diluent gas is achieved by using a split stream saturator [27]. Temperature, humidity and pressure are monitored in the final gas stream, which is typically reduced from 10 L/min to 500 mL/min over the exposed substrate. The whole assembly is mounted in a fume cupboard.
Post-exposure Analysis of Absorption and Penetration
Quantification of skin absorption and penetration requires a suitable analytical method. Chemical interactions with skin could be studied, and suitable detection techniques were identified and utilized for a range of toxic gases, using standard calibration curves and relevant blanks. Receptor fluid could be analyzed to determine gas penetration, and following exposure, the amount of gas absorbed was assessed by rapid dismantling of the cell and extraction of the skin substrate in a vial.
Chemicals of Interest
In Australia, using a combination of intelligence assessments , hazardous materials properties and use a list of materials was created, often referred to as the COAG (Council of Australian Governments) list of hazardous materials [28]. Similar lists have been complied by other nations [29, 30]. These lists are applied as a basis to assist communities in focusing their efforts to prevent the misuse of such materials and prepare to manage the consequence of attacks using hazardous materials. The materials are a mixture of solids, liquids and gases and range from toxic chemicals like parathion (organophosphate) to corrosive toxic gases like hydrogen chloride. They are generally toxic and/or corrosive materials.
The toxicity of the chemical is a crucial factor. Toxicity and classification criteria are described in the United Nations recommendations on the transport of dangerous goods. The toxic effect of a material is dependent on several factors, including the route of entry. Materials most toxic by inhalation find favor for deliberate use. The most effective materials to be dispersed are selected on a combination of their toxicity and physicochemical properties , such as volatility, state, and reactivity.
The prioritization of chemicals to be included in the skin decontamination studies should be based on the national lists combined with their intrinsic properties. Toxic and/or corrosive chemicals naturally found in the gaseous state are highest priority. In order to contaminate mass numbers of people, the threat material would likely be a gas or vapor.
For an initial study by the authors, ten chemicals of high priority were addressed. Based upon the way in which the chemicals interact with skin, they were divided into categories of reactive gases, biochemical degradation gases, and fumigant gases.
Reactive Gases
Chlorine
Inhalation is the most significant route of exposure, with approximately 1000 ppm being rapidly fatal, but exposures at half this concentration will be fatal after some delay (over 30 min). This is a deep lung irritant resulting in delayed chemical pneumonitis at lower concentrations. Skin exposure to the gas results in significant irritation, and at higher concentration, blisters and chemical burns, although there are no reports of serious systemic effects following skin exposure only, suggesting poor dermal uptake. Occupational standards do not suggest a skin notation.
Ammonia
A potent upper respiratory tract irritant, ammonia has excellent warning properties for inhalational exposure, resulting in severe lacrimation and irritation on exposures to relatively low concentrations. The physiological response to ammonia is a reduced depth of tidal breathing, limiting the extent of penetration of ammonia into the deeper lung. Short durations of inhalational exposure may be tolerated in this way, although this breathing pattern is not sustainable for extended duration exposures. Skin is a target for toxic effects, with erythema, irritation and chemical burns seen after exposure to ammonia gas, although this has most often been associated with direct exposure to releases from pressurized containers. Skin damage occurs from anhydrous ammonia gas at over 10,000 ppm (a concentration rapidly fatal by inhalation), although this may relate to the lower concentration of ammonium ion. Ammonia (ammonium) in aerosol form may exhibit a different (possibly higher) dermal toxicity.
Hydrogen Chloride
Deleterious effects of gaseous hydrogen chloride exposure are predominantly by the inhalation route, but lower concentrations result in severe irritation and corrosion of skin. Toxicity appears to be limited to the site of application, with physiologic pH buffering systems providing protection from severe systemic exposures, although there are few human studies that reliably examine the systemic characteristics of dermal exposures. Ocular damage is likely at exposures likely to be significant for skin.
Sulfur Dioxide
Sulfur dioxide may enter the body via the respiratory tract or, following dilution in saliva, it may be swallowed and enter gastrointestinal tract in form of sulfurous acid. Due to its high solubility, SO2 is rapidly distributed throughout the body. In the blood, sulfuric acid is metabolized to sulfates which are excreted in the urine [30]. Most people can smell sulfur dioxide at levels of 0.3 to 1 ppm [31].SO2 is very soluble in, and reactive with, water. In the moist pulmonary environment, SO2 produces sulfurous acid (H2SO3), a severe irritant, in addition to H+, bisulfate (HSO3-), sulfite (SO3=), which in turn affect the smooth muscles and nerves involved in bronchoconstriction [31–33]. There are no available studies addressing dermal absorption of sulfur dioxide.
Biochemical Degradation Gases
Hydrogen Cyanide
Rapidly fatal by inhalation but lower airborne concentrations are associated with moderate dermal absorption, shown in a few anecdotal reports and in in vitro skin absorption studies [34–36]. Hydrogen cyanide is a relatively unreactive, small molecule with skin permeation outcomes likely to be related to solubility and ionization. In animal investigations and in vitro cases, exposures are continuous, whereas in the event of an environmental release, the duration of exposure may be likely to be short, resulting in a lower total absorption of CN.
Hydrogen Sulfide
Pungent with strong olfactory warning properties initially (prior to olfactory fatigue), and with significant respiratory toxic effects, hydrogen sulfide is a deep lung irritant capable of causing delayed chemical pneumonitis. Absorption through skin is possible although the toxic sequelae have not been reported (other than death within hours in animals with dermal exposure). For example, historic animal in vivo dermal exposure studies indicate some potential for H2S gas to be absorbed through skin but only at very high concentrations and long experimental time frames [35, 37]. No skin notation is recorded for occupational standards. The corneal surface appears sensitive to hydrogen sulfide, with eye symptoms reported with extended duration exposures.
Nitrogen Dioxide
A deep lung irritant, NO2 has been associated with significant respiratory symptoms with acute exposures and long term respiratory damage with repeated exposures at lower levels. While skin effects have been reported (linked to dissolution of nitrogen dioxide in sweat to generate nitric acid) there is little evidence to suggest a significant systemic toxicity associated with dermal exposure [38].
Fumigant Gases
Phosphine
Phosphine is a deep lung irritant with effects by the respiratory route dominating its toxicity. Phosphine does not have a skin notation for occupational standards. No dermal exposure studies for phosphine gas in humans or animals have previously been reported in the literature.
Methyl Bromide
Direct dermal contact with methyl bromide can lead to severe injury to the skin [39] Symptoms usually do not appear immediately, but develop a few hours after exposure. Early signs typically include a burning or itching sensation, with erythema, edema, and large blisters that resemble second-degree burns developing somewhat later. In the presence of moisture, methyl bromide breaks down to methanol and bromide ion. This may be pH- and temperature-dependent (alkaline conditions increases bromide ions). The published human dermal exposure data for methyl bromide are principally associated with occupational accidental exposures [40, 41]. Methyl bromide has been assigned a “skin notation” in the context of workplace exposure standards, suggesting the potential for skin absorption [42].
Sulfuryl Fluoride
Sulfuryl fluoride is noncorrosive and not very reactive; it does not hydrolyze in water but is hydrolyzed by aqueous sodium hydroxide solution [42]. Based on its low boiling point and high vapor pressure, sulfuryl fluoride is expected to remain a gas over a wide range of ambient temperatures, thus dermal and oral exposure to surface residues are expected to be insignificant. Although no available studies address dermal absorption of sulfuryl fluoride, mucous membrane and dermal permeability are expected to be very low based upon sulfuryl fluoride’s relative insolubility in water and low octanol/water partition coefficient [43].
Detection Methodologies
Detection techniques used to assess chemical interactions with skin
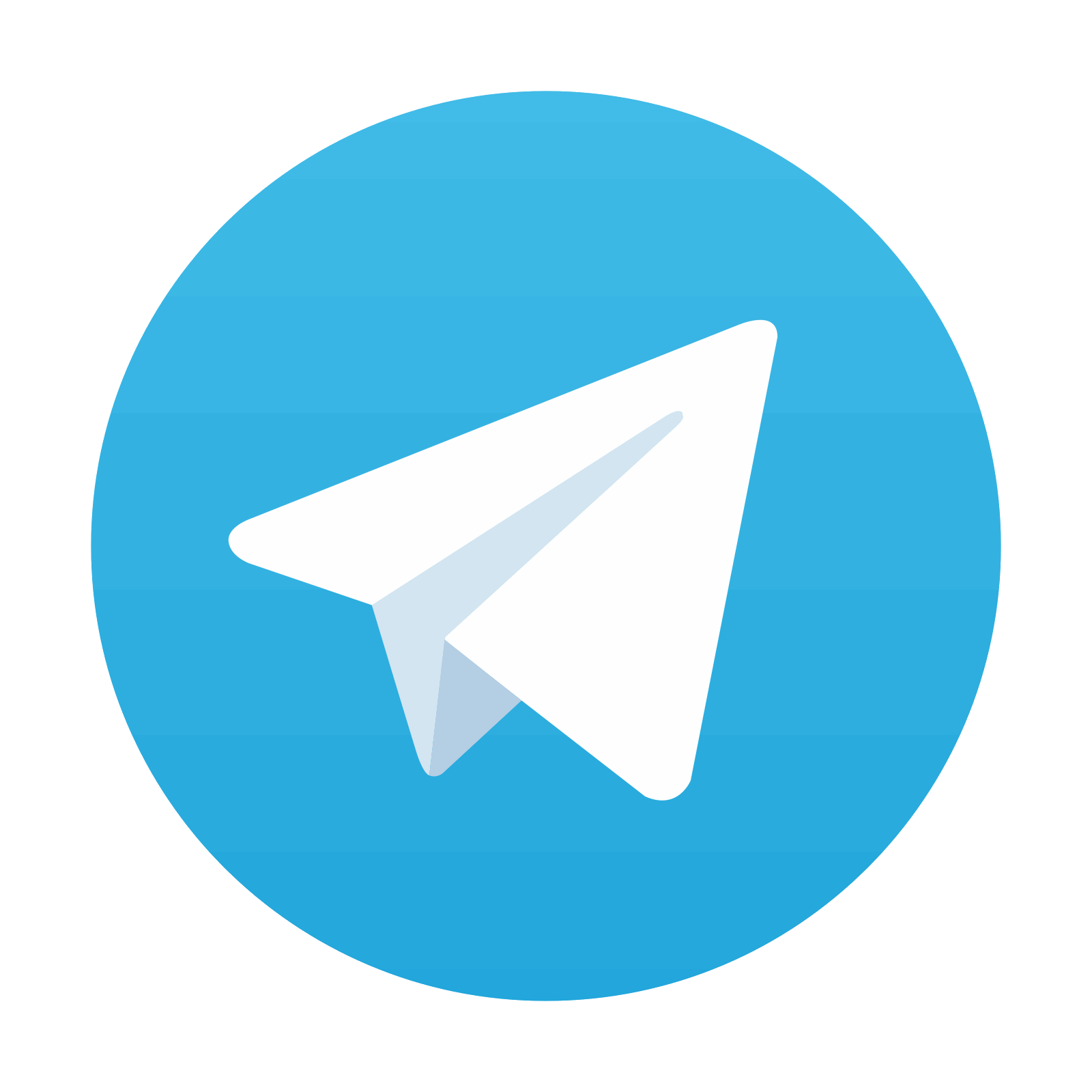
Stay updated, free articles. Join our Telegram channel

Full access? Get Clinical Tree
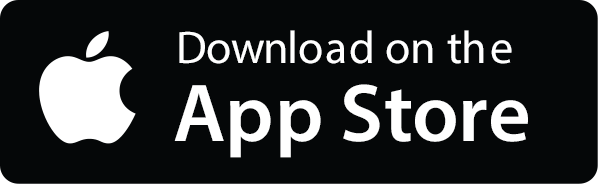
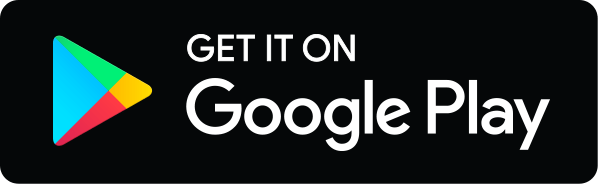
