Laser-Assisted Liposuction
Keywords
• Laser lipolysis • Laser-assisted liposuction • Nd YAG laser
Over the past 30 years, liposuction has become an increasingly popular procedure. According to the American Society for Aesthetic Plastic Surgery, liposuction has been the most commonly performed cosmetic surgical procedure every year of this decade.1 From its modern reinvention over 30 years ago, in which large uterine curettes and gynecologic aspirators were used to remove fat,2,3 to the myriad technological advances that exist today, liposuction has undergone many changes. These changes can be divided into three main categories: changes in anesthesia management, changes in equipment and cannula size, and changes in the actual methods of treating and removing fat.
In the 1980s, liposuction usually was performed as a hospital inpatient procedure under general anesthesia, and it often required transfusion of autologous blood to replace that which was lost during the procedure. In 1988, Klein4 published his landmark article on the tumescent technique; this method of administering large quantities of very dilute buffered lidocaine and epinephrine significantly reduced intraoperative blood loss and allowed the procedure to be moved from an inpatient setting to an office or other outpatient setting. Although slow to be adopted by many plastic surgeons, when properly used, Klein’s tumescent technique dramatically improved the safety of liposuction and became incorporated into the procedure.5
The dramatic reduction in intraoperative blood loss and postoperative ecchymoses improved the recovery for patients undergoing liposuction. Surgeons continued to refine the procedure with the addition of internal ultrasound-assisted liposuction,6–8 external ultrasound-assisted lipoplasty,9 and power-assisted lipoplasty.10 Parallel to these developments, Apfelberg11–13 was beginning to study laser-assisted liposuction; this preliminary investigation utilized a YAG optical fiber contained within a liposuction cannula. The investigators concluded that no clear benefit was demonstrated with the laser; the US Food and Drug Administration (FDA) did not approve the technique, and the sponsoring laser company did not pursue the study. In the late 1990s, Neira and colleagues began studying the effects of low-level laser on adipose tissue.14–17 At the same time, Blugerman18 and Blugerman, Schavelzon, and Goldman19–21 were using 1064 nm neodymium:yttrium-aluminum-garnet (Nd:YAG) laser energy, conducted by means of an optical fiber, within a 1 mm introducer cannula, in direct contact with adipose tissue. They found that the energy from the laser resulted in adipocyte lysis and other salutary side effects of the procedure and patient recovery. Badin and colleagues22,23 arrived at very similar findings: less intraoperative blood loss, less postoperative ecchymoses, and improved skin tightening and skin redraping during the recovery process.
Science
The laser–tissue interaction in adipose tissue has been described by many investigators.11–28 Whether the laser acts directly upon the fat18–25 or whether it is administered transcutaneously,14–17 the final common pathway appears to be similar. Neira,14–17 Goldman,24 Ichikawa,25 and their respective colleagues have elucidated this.
Although these findings could not be confirmed independently by Brown and colleagues,26 Ichikawa and colleagues25 subsequently showed the histologic findings in fat subjected to direct treatment with the 1064 nm Nd:YAG laser. In both photomicroscopic and scanning electron microscopic studies, Ichikawa demonstrated greater destruction of human adipocytes in laser-irradiated fat compared with nonlaser-irradiated controls. There seemed to be a dose-dependent degeneration of adipocyte cell membranes, vaporization and liquefaction of fat cells, and collagen fiber coagulation in response to the 1064 nm Nd:YAG laser irradiation.
With an Nd:YAG laser in direct contact with adipose tissue, Goldman20,24 and Badin22,23 demonstrated adipocyte cell membrane rupture, coagulation of small vessels within the adipose tissue, coagulation of adipose and dermal collagen, and a reorganization of the reticular dermis (Figs. 1 and 2). The latter is associated with a process of neocollagenesis in the deep dermis and the dermal fat junction. These histologic findings seem to correlate with the clinical findings of a reduction in intraoperative blood loss, reduction in postoperative ecchymoses, a more comfortable postoperative recovery, a rapid return to activities of daily living (ADL), and enhanced skin tightening and skin redraping as a result of the neocollagenesis.
Kim and Geronemus27 also confirmed similar findings; the lipolysis produced by the Nd:YAG laser acting in adipose tissue is an elegant and minimally invasive option associated with demonstrable reduction in fat volume, irrespective of subject weight change. There was excellent patient tolerance and the benefit of dermal tightening. The postoperative recovery was quick, and patients had a rapid return to ADL. MRI studies performed before and 3 months following surgery showed an average 17% reduction in adipose tissue volume in the areas treated; site-specific analysis revealed a 25% reduction in the submental area and an overall 14% reduction in the trunk and extremities.
Mordon and colleagues,28 also demonstrated similar clinical and histologic findings using a continuous-wave 980 nm diode laser (Pharaon [Osyris; Hellemmes, France] and Lipotherme [MedSurge Advances, Dallas, TX, USA]). At similar power settings as the pulsed Nd:YAG, the histologic findings were similar. This laser has the capability of higher wattage than the Nd:YAG laser; however, at these higher powers, there was carbonization noted in the adipose tissue and collagen fibers. This is significant, because carbonization and charring of tissues is much more likely to lead to unfavorable and unacceptable scarring internally and possibly externally. Perhaps the different pulse profile may be responsible for this; the continuous-wave diode and the pulsed Nd:YAG interact with adipose tissue in a different manner. The continuous waveform is more likely to char the tissues than a high peak power, but short duration pulsed waveform. The latter is more in harmony with the thermal relaxation time of the tissues.
The mechanism of action on a cellular level is caused by a specific laser–tissue interaction that is defined by the process of selective photothermolysis29; some features of this interaction are wavelength-dependent, and some are independent of wavelength used. There are three wavelengths currently on the market in the United States for laser lipolysis: pulsed 1064 nm, pulsed 1320 nm, and CW 980 nm diode. All three wavelengths generate infrared light energy that is absorbed by adipocytes and converted to heat. This absorption of energy causes deformation of the adipocyte, volume expansion and, subsequently, cell rupture. The adipocytes exposed to the highest energies undergo a photoacoustic or photomechanical disruption; those exposed to lower energies (further from the tip of the optical fiber) undergo a photothermal change. The latter results in cellular deformation and expansion, but may not lead to immediate rupture; because of the changes in membrane permeability, however, many of these cells eventually die. The cellular debris is metabolized, and the metabolic by-products are excreted by means of the liver or kidneys. An additional effect of the laser-induced heating of the tissues appears to be a photostimulatory effect; this phenomenon is a lower energy process that occurs even further from the fiber tip and has broad overall activity on dermal and adipose collagen.
The 1064 nm wavelength also is known to be absorbed by oxyhemoglobin, and has even better absorption by methemoglobin (Fig. 2). Because of these absorption characteristics, this wavelength is effective in coagulating small blood vessels within the fat and seems to be responsible for the histologic and clinical findings noted by several investigators.18–25
As of June 2008, there were four devices approved by the FDA for laser lipolysis in the United States (Table 1
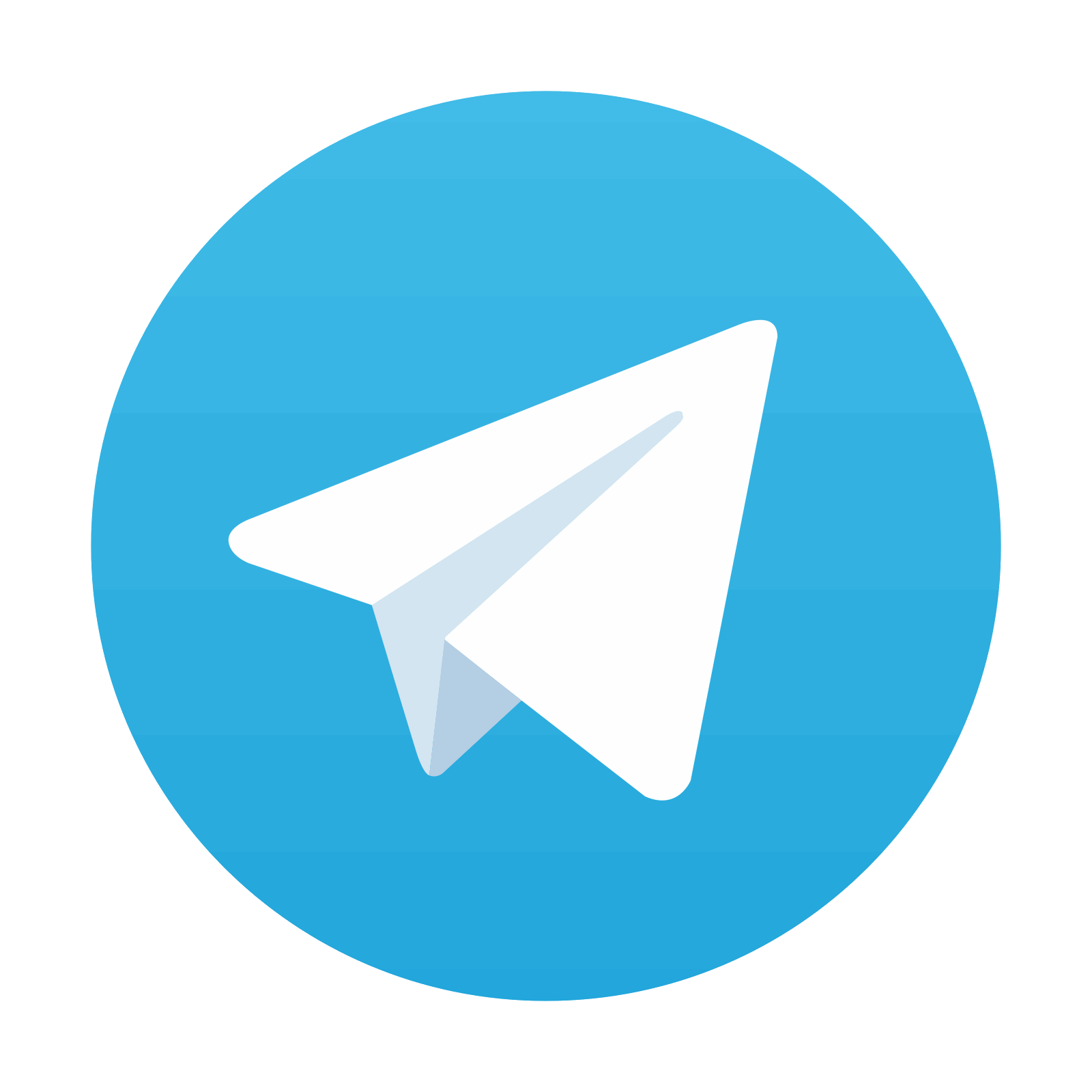
Stay updated, free articles. Join our Telegram channel

Full access? Get Clinical Tree
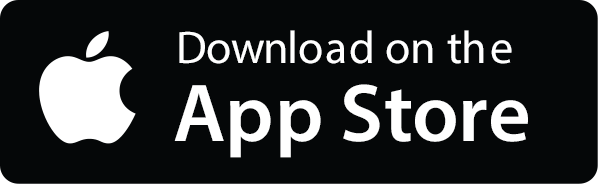
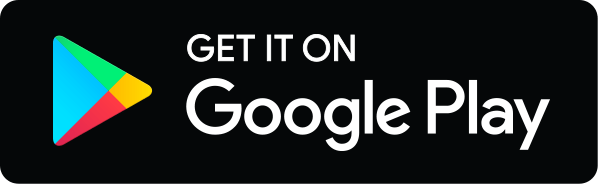