Lasers and High-Intensity Pulsed Light
INTRODUCTION
Since the first application of lasers in medicine and surgery, the safe use of light energy to painlessly eliminate spider veins of the leg has been the holy grail of laser technology. However, lasers typically do not achieve equivalent results; sclerotherapy remains the gold standard of treatment for leg veins. As the successful use of lasers and light sources for facial vessels is widely known, patients seeking cosmetic treatment for their legs often request laser as they believe it is more effective, less painful, and less invasive than sclerotherapy. Although sclerotherapy causes an inflammatory reaction sometimes accompanied by side effects such as postsclerosis pigmentation and/or telangiectatic matting, lasers cause tissue destruction by conversion of light energy to heat absorption by the target tissue.1 This heat denatures protein, primarily vein wall collagen, although other blood proteins and muscular layers can denature as well. Heating is a process that should theoretically lead to less of an inflammatory reaction than that seen with sclerosing solutions, but this is not always the case.
One of the responsibilities of the treating physician is to explain to the patient the differences between thermal and chemical destruction in terms of efficacy, risks, and side effects. Some recent advances have permitted lasers and intense pulsed light (IPL) devices to become methods for treating telangiectatic vessels of the leg with reduced risks of adverse effects and greater efficacy compared to previous devices. Consensus over a perfect device has not been reached. Consideration of the principles and physics involved will allow the physician to choose a laser or light source suitable to the size, location, and oxygenation state of a targeted vessel as well as the skin color of the patient.
Laser Versus Sclerotherapy
A complicating factor for laser treatment of leg veins is that most telangiectases are associated with high reverse pressure from associated reticular veins. Most lasers or IPL (except possibly 1064-nm lasers) will not treat the associated high-pressure reticular veins. The success rate is therefore greatly diminished for any treatment of telangiectasias when proximal hydrostatic pressure remains. Additional problems with treating leg veins as opposed to facial veins are the deeper and varied locations of leg veins, their thicker and larger walls, the overall larger vessel diameter through which the laser must penetrate, and the reduced oxygenation state leading to a violaceous rather than red color. Red telangiectasia have been found to have an oxygen saturation of 76 percent compared with blue telangiectasia that have an oxygen concentration of 69 percent.2 Thus, each type of telangiectasia may have a slightly different optimal wavelength absorption based on its color in addition to its relative size and depth. With all of these hurdles, it is inherently more difficult to get photons safely and in sufficient number through melanin into the target chromophore in leg veins as compared to facial vessels. One must employ specific measures to decrease the risk of burning the epidermis when treating leg veins.
It must be understood that for leg telangiectasias, sclerotherapy remains the gold standard of treatment. Lasers are adjunctive and not replacement therapy. The newest technological improvements of lasers, however, have improved the rate of success and are slowly beginning to challenge these perceptions. These modifications include:
• Longer wavelengths that penetrate more deeply and interact less with melanin;
• Use of epidermal cooling devices including dynamic (spray cooling) versus contact cooling to protect the epidermis;
• Longer pulse durations that allow more thorough heating of larger vessels; and
• Larger spot sizes that allow more photons to reach the target.
One must explain to patients that injection directly into the target vessel with subsequent spread of solution into connecting telangiectasias is a more efficient method that will treat larger areas more quickly than laser. Particularly when patients have large reticular networks, they are informed that failure to eliminate hydrostatic pressure sources will render the laser treatment less effective, with shorter term results, and require many more repeated treatments. Laser treatment is considered when patients are irrationally fearful of needles, have concerns of allergy to sclerosant, or severe hypercoagulability.
For patients who insist on laser treatment, consider performing tests of both methods (laser and sclerotherapy) on the initial visit, thus allowing the patient and physician to compare results and to choose the modality at the subsequent treatment session. Often the combination of treatments will allow efficient elimination of associated reticular veins with treatment of the smaller, more difficult to cannulate telangiectasias with the laser or light source. A very simplified comparison of sclerotherapy versus laser can help guide patient decision-making (Table 20-1).
TABLE 20-1
Simplified Comparison of Laser and Sclerotherapy
Indications for Primary Laser Treatment of Leg Veins
Although sclerotherapy is the treatment of choice for most small telangiectasias, there are several clinical states in which lasers or IPL is primarily indicated (Table 20-2). When no apparent connection exists between deep collecting and reticular vessels and the superficial telangiectasia, this telangiectasia may arise from a terminal arteriole or arteriovenous anastomosis.3 These telangiectasias may be treated without consideration of underlying forces of hydrostatic pressure. They occur far more commonly near the ankle and appear as bright red telangiectasias with a central point of origin. Use of sclerotherapy incurs the risk of injection into the arteriolar component with subsequent risk of delayed skin necrosis.
TABLE 20-2
Primary Indications for Lasers/IPL for Leg Veins
A-V malformations—bright red, central source
Ankle telangiectasias (for inexperienced injectors)
Resistance to sclerotherapy
Fine matting postsclerotherapy
Hemangiomas (ectasias)—cherry, venous lake
Needle phobia
Patients prone to pigmentation from sclerotherapy (choose wavelength wisely)
In patients prone to hyperpigmentation from sclerotherapy, the destruction of endothelial cells through thermal damage caused by lasers and IPL is theoretically believed to produce less inflammation and subsequent hyperpigmentation compared to the damage of the vessel wall caused by sclerosing solutions. This assumes that the patient’s natural pigmentation is taken into account when choosing and utilizing the laser or IPL.
When patients have been repeatedly treated by sclerotherapy without good results, and all the measures outlined in Chapter 13 have been followed, then lasers should be considered as an alternative treatment. The needle phobic patient who becomes vaso-vagal at the sight or thought of a needle penetrating the skin is also an excellent candidate. Laser best treats some superficial vascular ectasias such as cherry angiomas and deeply purple venous lakes. Sclerotherapy has no advantage in these cases, incurs greater risks of necrosis, and is technically very difficult. Lasers may also treat resistant matting following sclerotherapy; in particular, devices that treat bright red superficial blood vessels, such as the yellow light lasers and IPL, have the best results.
Principles of Lasers for Vascular Lesions
In 1981, Anderson and Parrish introduced the theory of selective photothermolysis, which is the basis of most of the lasers and light sources in use of vascular indications.4 This principle dictates that selective destruction of the target takes place with minimal damage to surrounding structures. Selective light-induced thermal damage occurs by selecting a wavelength that is maximally absorbed by the targeted structure combined with a pulse duration that is less than the thermal relaxation time of the target, or the time required for the target to dissipate 50 percent of the heat acquired following laser irradiation. The most widely understood example of selective photothermolysis is that of pulsed dye lasers (PDLs) in the treatment of port wine stains.5
To understand the best wavelength to treat superficial red vessels, one must know that oxyhemoglobin’s primary absorption peaks are in the blue-green-yellow portion of the visible range (418, 542, and 577 nm), but that the lowest wavelength peak of 418 nm is strongly absorbed by melanin and cannot be utilized for vascular lesions (Figures 20-1 and 20-2A). Even light with wavelengths at the peak of 542 nm, unless cooling protects the epidermis, will have too much absorption by melanin and therefore fail to be photoselective, with probable epidermal damage. There is a fourth broadband hemoglobin absorption peak from 700 to 1100 nm, which becomes more significant for more deeply situated vessels, as such wavelengths may penetrate over 3 mm into the dermis to actually reach these deeper vessels. In general, longer wavelengths penetrate more deeply into the skin with less scattering of the photons and greater penetration to a deeper target. So not only do they penetrate more deeply, but they also heat the target more uniformly.
FIGURE 20-1 Absorption spectrum of hemoglobin. (Courtesy of ESC/Sharplan, Norwood, MA.)
FIGURE 20-2 A. Color spectrum guide for lasers with melanin absorption shown in brown. Melanin absorbs very little at 1064 nm. B. Vessel heating as a function of size and depth. (Courtesy of Lumenis, Santa Clara, CA.)
We now understand that telangiectasias and venulectases of the leg are at certain points deeper vessels (over 1 mm), thus requiring a longer wavelength to allow penetration. However, even at a penetrating wavelength, pulse duration must be matched to vessel size. The pulse duration is determined by the thermal relaxation time that can be up to 160 ms for a 0.4-mm vessel. As depth and size of the vessel change so do the absorption characteristics (Figure 20-2B).6 Large diameter vessels require a longer pulse duration to allow sufficient time for diffusion of heat evenly throughout the cylindrical vessel lumen.7
Various lasers have been utilized in an effort to enhance clinical efficacy and minimize the adverse sequelae of telangiectasia treatment. The optimal light source would have a wavelength specific for the vessel treated and be able to penetrate to the depth and diameter of the vessel through its entire course. Unfortunately, for the treating physician with a laser, leg telangiectasias course along at different depths in the skin and subcutaneous tissue,8 unlike facial vessels that are more uniform in distance from the epidermis. For telangiectasias, wavelengths at between 600 and 900 nm have been predicted to be most useful for vessel depth.9 Ideally, a light source should have a pulse duration that would allow the light energy to build up in the target vessel so that its entire diameter is thermocoagulated.
During the process of delivering a sufficient quantity of energy to thermocoagulate the target vessel, the overlying epidermis and perivascular tissue should be unharmed. This requires minimal interaction with melanin and some mode of protective epidermal cooling. A number of different laser and IPL systems have been developed with this goal, with cooling incorporated into the treatment handpiece. Cooling may be applied by direct contact, cold air blowing, or spray cryogen. Cooling may also be applied before, after on continuously during the laser pulse. Fluence needs to be adjusted on the basis of the baseline vessel temperature. As a general rule, the colder the vessel from prechilling, the higher the fluence required. We believe, as others, that cooling is essential for safe treatment of leg veins by lasers.10
The principles of laser treatment of leg veins are outlined in Table 20-3. Lasers should be analyzed in terms of the color of light they produce, starting with green, then yellow, red, and infrared. A color spectrum diagram to assist in understanding the laser wavelengths relative to melanin absorption is shown in Figure 20-2A. Treatment endpoints are discussed later. Endpoints are visual clues that effective heating has occurred and include immediate vessel contraction, visible intravascular coagulum (photodarkening), or rupture of the vessel with surrounding immediate or delayed purpura.
TABLE 20-3
Principles of Laser Treatment for Leg Veins
LASER SOURCES FOR PHOTOTHERAPY OF TELANGIECTASIA
Argon (Blue-Green)
The argon laser (488 and 511 nm) produces wavelengths absorbed by hemoglobin and to a lesser, although significant, extent by melanin. Its relatively short wavelength, combined with a spot size of 1 mm or less, prevents its penetration much beyond 0.5 mm. When the patient is pigmented or tanned, epidermal melanin will selectively absorb the laser energy preventing penetration below the epidermis. This absorption of heat by the epidermis typically results in thermal injury. Thus, the argon laser is not recommended for leg veins due to its relative non-specificity and associated unacceptably high risk of adverse sequelae in treating leg telangiectasia. Hypopigmented scarring is a frequent occurrence, as is treatment failure (Figure 20-3). For these reasons, the use of this laser for the legs has been abandoned.11–13
FIGURE 20-3 Hypopigmentation after argon laser on the legs. Similar hypopigmentation can occur after 532-nm treatment.
KTP Crystal Frequency Doubled Nd:YAG (532 nm; green)
Potassium titanyl phosphate (KTP) laser generally refers to a laser beam generated by a neodymium:YAG (Nd:YAG) laser, which is directed through a KTP crystal to produce coherent light in the visible green portion of the spectrum. The 532-nm wavelength is one of several hemoglobin absorption peaks. Although this wavelength does not penetrate deeply into the dermis (about 0.75 mm), damage can occur in the vascular target by selecting a more vessel-specific pulse duration (10–50 ms, compared to argon laser’s 100 ms), although absorption by melanin is still significant. KTP lasers that utilize a 1- or 2-mm diameter beam focus too much heat in one spot in the target vessel with less than optimal results (Figure 20-4A).14
FIGURE 20-4 Postlaser hyperpigmentation. A. Hyperpigmentation after KTP. B. Pigmentation following PDL treatment of telangiectasias lasting as long as eight months. (Courtesy of Cyrus Chess, MD, Norwalk, CT.)
A redesigned long-pulse 532-nm laser (frequency-doubled Nd:YAG; Versapulse®, Coherent, Inc., Palo Alto, CA) was more effective in treating leg veins due to its larger spot sizes and the use of epidermal cooling. It is no longer manufactured and has been replaced by the Gemini laser (Gemini®, Iridex, Mountain View, CA). Utilizing fluences between 12 and 20 J/cm2 delivered with a 3–5-mm diameter spot size, a train of pulses is delivered over the vessel until spasm or thrombosis occurs. For leg vessels less than 1 mm in diameter which are not directly connected to a feeding reticular vein, and with the use of a 4°C chilled tip to protect the epidermis, this method can be effective. There is considerable variation in results reported by individual physicians, with more than one treatment typically necessary for maximal vessel improvement.15 This laser is best used for vessels recalcitrant to other lasers, IPL and sclerotherapy treatment, or post-sclerotherapy matting. Enhancements with a longer pulse duration of 20-50 ms and an increase in spot size to 5 mm for the highest fluence of 20 J/cm2 have improved the performance of the 532-nm frequency-doubled Nd:YAG. However, response rates of 33 percent are typically reported with 10-ms pulse durations for leg veins.16 It has shown increased efficacy with reduction in nonspecific pigmentary changes seen with the shorter pulse duration (Figure 20-5).
FIGURE 20-5 Frequency-doubled Nd: YAG with 10-ms pulse duration and epidermal cooling. A. Immediate (10 min) linear urticarial reaction is seen along the treated vessels, a desired visual endpoint. B. Hypopigmented streaks are seen along with vessel clearance at one month. Most of the hypopigmentation cleared after six months.
Pulsed Dye Laser (Yellow 585-595 nm)
Although PDL is no longer typically divided into short PDLs (SPDLs) and long PDLs (LPDLs), it is important to understand historically that these terms were used. The PDL was initially demonstrated to be highly efficacious in treating cutaneous vascular lesions consisting of very small superficial vessels, including portwine stains (PWS), hemangiomas, and facial telangiectasia at short pulsed durations of 450 ms (0.45 ms).17 The depth of vascular damage for a short pulse is estimated to be 1.5 mm at 585 nm. The pulse duration of the first generation of PDL was 450 μs, optimal for the 50–100 μ diameters of PWS vessels. This pulse duration was believed to be effective for treating leg telangiectasia less than 1 mm in diameter at one time,18–20 but was ultimately found to be ineffective for consistent response for treating larger telangiectasias on the legs. Vessels that should optimally respond to PDL treatment are predicted to be red telangiectasia less than 0.2 mm in diameter, particularly those vessels arising as a function of telangiectatic matting postsclerotherapy. The primary problem for treatment of cosmetic telangiectasias on the leg is the purpura that can lead to long-standing hyperpigmentation. Patients thoroughly detested the long-lasting pigmentation that commonly replaces the fine telangiectasias for months before fading with the standard short-pulse (450 μs) PDL (Figure 20-4B).
The PDL produces vascular injury in a histologic pattern that is different from that produced by sclerotherapy.19
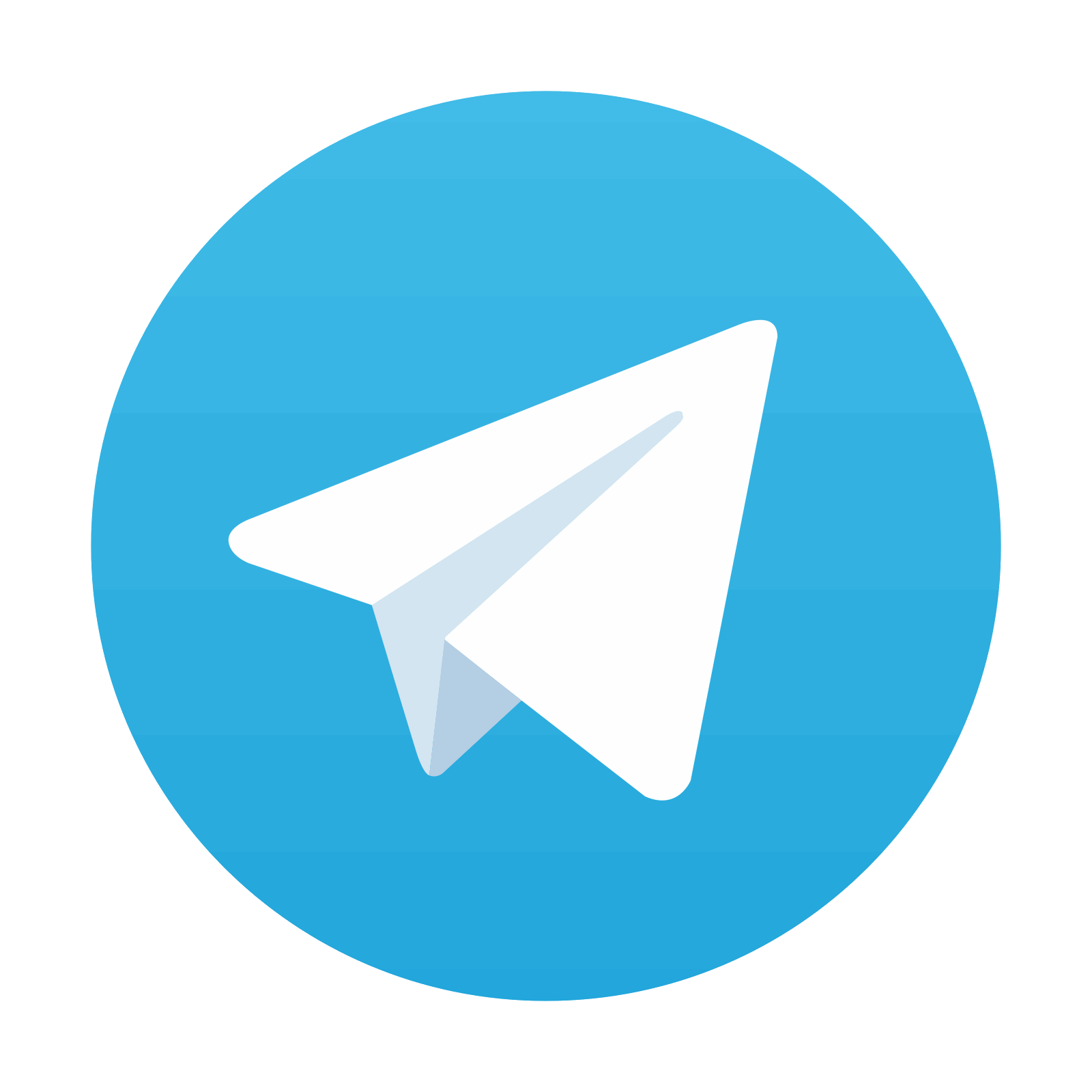
Stay updated, free articles. Join our Telegram channel

Full access? Get Clinical Tree
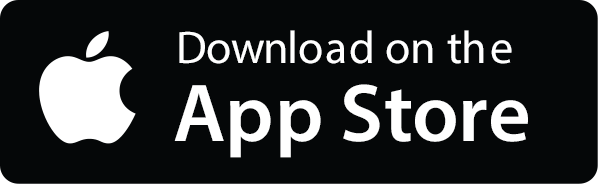
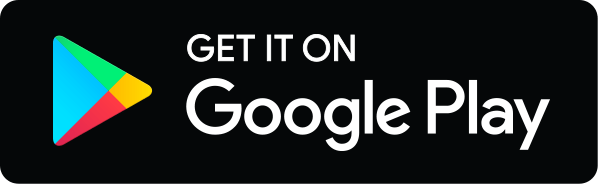