Lasers and Flashlamps in Dermatology: Introduction
|
Principles and Safety
Laser emits radiation whose properties are fundamentally different compared to radiation of other sources. Laser radiation is monochromatic in a range from 100 nm to 3 mm, appears as strictly parallel beam, can be either continuous or pulsed in a range from seconds to femtoseconds (10−15 s), and can show intensities of more than 1010 W/cm2.
A few years after development of lasers in 1960, physicians such as Leon Goldman started to apply this new and exciting radiation in dermatology. Since 1963, numerous physicians have investigated the use of various types of lasers to treat different skin disorders. When laser radiation is absorbed in tissue, radiation energy is predominantly converted to heat. Because of its exceptional nature, laser radiation can be applied to cut, vaporize, coagulate, or ablate skin. It was identified as able to achieve selective destruction of targets inside skin. Laser pulses precisely destroy even very small targets in skin such as small vessels or melanosomes. By manipulating the appropriate laser parameters such as wavelength, pulse duration, and radiant exposure, the destruction of a target occurs without damage to the adjacent tissue, which substantially minimizes the risk of side effects like scarring. The selection of the appropriate laser parameters for an effective and precise destruction of targets in skin were summarized as the rules of selective photothermolysis.1
A newer light source appeared in dermatological practice some years ago: the intense pulsed light source (IPL).2,3 It mainly consists of a flashlamp that emits white light ranging from about 250–1,400 nm. The spectrum is narrowed (∼500–1,000 nm) by applying optical edge-filters (cut-off filters) and water film in front of the flashlamp. In contrast to lasers, IPL emission shows a broad spectral range of up to 500 nm and the pulse duration is limited to milliseconds. IPL radiation can be successfully applied for some dermatological indications by achieving partially selective photothermolysis.
Electromagnetic radiation can be described as a plane wave that is propagating in space with a constant velocity c of 299,790 km/s. The major properties of radiation are wavelength λ and frequency ν, which are correlated:
Radiation also can be considered as particles, which are called photons, with a specific energy
where h is Planck’s constant (6.6 10−34 Js). The energy of radiation increases with decreasing wavelength. The spectrum of electromagnetic radiation exhibits a broad range, with wavelengths ranging from a few nanometers to thousands of meters. Only a small part of the spectral range is visible for human eyes (400–700 nm); this, and only this, is light.
The generation of laser radiation is a complex process. The acronym LASER is derived from the most important elements: Light Amplification by Stimulated Emission of Radiation.
The process of spontaneous emission of photons describes the emission of radiation from a usual light source such as light bulb. After excitation, atoms or molecules can emit photons at different times, in any direction and usually with different wavelengths. Therefore, such a radiation is incoherent, divergent and spectrally broadband.
In contrast, a laser medium consists of identical atoms or molecules (photon emitter, Table 239-1), which are excited by different means such as electrical discharge (gas lasers) or optical radiation (flashlamp pumped lasers). These photon emitters are placed in a substrate, which is either gas, liquid, or solid state. Together the photon emitters at a certain concentration and the substrate represent the laser medium. After excitation, which is called pumping, the atoms or molecules return from the excited to the ground state and thereby emit photons with a specific wavelength λL that is determined by the energy difference ΔE of the excited and the ground state with
Name | Wavelength (nm) | Photon Emitting Medium | Substrate | Type | Typical Mode Pulse Duration |
---|---|---|---|---|---|
Excimer laser | 308 | Excited dimer molecules (Xe-Cl) | Different gases | Gas | Pulsed μs–ms |
“KTP” laser (Nd:YAG laser) frequency doubled) with KTP crystal) | 532 | Nd3+ | Crystal: Yttrium-aluminum Garnet (Y3Al5O12) | Solid state | Pulsed ms–ns |
Dye laser | 585–600 | Dyes, e.g., Rhodamines | Organic solvents | Liquid | Pulsed ms |
Ruby laser | 694 | Cr3+ | Al2O3 | Solid state | Pulsed ms–ns |
Alexandrite laser | 755 | Cr3+ | Chrysoberyl (BeAl2O4) | Solid state | Pulsed ms–ns |
Diode laser | Different wavelengths (e.g. 810, 940) | InGaAs AlGaAs | Semiconductor material | Solid state | Pulsed ms |
Nd:YAG laser | 1,064 | Nd3+ | Crystal: Yttrium-aluminum Garnet (Y3Al5O12) | Solid state | cw, pulsed ms–ns |
Er:YAG laser | 2,940 | Er3+ | Crystal: Yttrium-Aluminum Garnet (Y3Al5O12) | Solid state | Pulsed ms |
CO2 laser | 10,600 | CO2 | Different gases | Gas | cw, pulsed ms |
If one considers the same transition in all atoms or molecules in a specific laser medium, the emitted photons have the same wavelength λL leading to the monochromatic nature of laser radiation. If the ground state and the excited state are spectrally broadened, then laser transition is possible in a certain wavelength range and a laser is tunable to different wavelength λL within this spectral range.
The combination of such identical transitions and photons with the same wavelength λL in a confined volume (laser medium) allows another process to come to the fore: the stimulated emission of photons, as described and published by Albert Einstein in 1917.4 Figure 239-1 shows the basic process of stimulated emission of radiation. One photon stimulates the next atom or molecule to emit a photon. The resulting two photons stimulate the next two atoms or molecules that results in four photons and so on. A laser medium consists of up to 1020 photon emitting atoms or molecules, which provides an immense number of stimulated photons. For example, a laser pulse with energy of 1 Joule and a wavelength in the visible spectrum contains about 1019 photons. Thus, the light amplification is actuated by the stimulated emission of radiation, as described by the acronym LASER. The process of light amplification does not change the energy of photons but increases their number exponentially.
To promote the stimulated emission of radiation and hence to optimize the process of light amplification, the laser medium is placed in an optical resonator that consists of two or more mirrors (Fig. 239-2). Due to the reflection of laser photons inside the resonator, the laser starts to oscillate and the number of photons continues to increase exponentially. Laser action can be achieved when the gain of photons in the laser medium is higher than the loss caused by spontaneous emission or absorption of photons. The temporal behavior of the laser emission depends on the temporal behavior of the excitation of the atoms or molecules. That is, when the energy supply to the laser medium is continuous, the emission of the laser is continuous wave (cw). When the energy supply is limited to a certain time interval by gas discharge or flashlamp, we speak of pulsed excitation, and the laser emits pulses accordingly. The laser pulse duration can vary from microseconds to milliseconds.
Figure 239-2
The number of photons increases rapidly due to stimulated emission (top). As the laser medium is placed between two mirrors, the stimulation of emission of radiation is continued by reflection of photons back and forth (bottom). The reflectivity (R) of one mirror is less than 100% and a small fraction of radiation can traverse this mirror (therapeutic radiation). This laser radiation is focused by a lens and fed into an optical fiber, which delivers it to the patient.
To achieve very short pulse durations in the nanosecond range, the resonator is additionally equipped with an optical polarizer and a nonlinear crystal. The polarizer sets the linear polarization of the laser beam that passes through the crystal. However, if an appropriate electrical voltage is applied to the crystal, the polarization of the photons is changed inside the crystal and subsequently blocked by the optical polarizer. This prevents photons from running back and forth inside the resonator (oscillation), which hampers light amplification and which is equivalent to a low circuit quality of the resonator. In such a case, the stimulated emission is minimized and the pumping of the laser medium produces a maximum number of excited atoms or molecules in the laser medium. When this maximum number is achieved, the electrical voltage of the crystal is switched off within nanoseconds and the photons can easily pass the crystal, which is equivalent to a high circuit quality of the resonator. Now, the laser oscillation immediately starts and the light amplification yields a very high number of photons per time unit due to the high number of excited atoms or molecules in the laser medium. The outcome of this circuit quality switch (Q-switch) is a very intense laser pulse with pulse duration in the range of nanoseconds.
The major component of an IPL is the flashlamp that emits radiation from the ultraviolet to the far-infrared part of the electromagnetic spectrum (Fig. 239-3). The broad emission spectrum is narrowed to match the absorption spectra of the different chromophores in skin. The infrared part is usually blocked for wavelengths longer than 950 nm using water in front of the glass applicator. The short wavelengths are blocked by an optical edge filter (cut-off filter), which is only transparent for radiation with wavelengths longer than the cut-off wavelength (e.g., 500 nm, 650 nm). The choice of cut-off filter depends on the chromophore and the target in the skin. A 500 nm cut-off is usually employed for vascular lesions, while a 650 nm cut-off is preferred for the melanin chromophores employed in hair removal. This cut-off also reduces the unwanted interaction with oxyhemoglobin in the vessels.
The main parameters of optical radiation are wavelength, optical power, intensity, the exposure time and radiant exposure. In the medical literature the phrase fluence is frequently used instead of radiant exposure. The correlation of these parameters are shown in Table 239-2, where the unit for energy is Joule (J); optical power Watt (W); intensity (W/cm2); radiant exposure (J/cm2); the exposure time second (s); and the area of irradiation on skin surface (cm2). After being transported through a glass fiber or articulated arm, laser radiation is usually applied as circular spot to the skin surface by means of a lens in a holder. The spot size and the energy of the laser radiation determine the radiant exposure (J/cm2). The distal end of a bare glass fiber can be placed inside tissue through a hollow needle for interstitial laser application. The IPL applicator directly contacts the skin through an ultrasonic gel. Thus, the radiant exposure of IPL emission is determined by the size of the applicator and the energy of radiation (J/cm2).
The photon is the smallest unit of radiation. The energy of a single photon at 532 nm (fd Nd:YAG laser, KTP) is about 4 10−19 J, which requires a high number of such photons in the order of 1019 photons to generate a single laser or IPL pulse. Table 239-3 shows examples of typical intensities that are emitted by IPL or lasers in medical applications. These intensities are substantially higher as compared to typical radiation sources in our daily life, such as light bulbs or solar radiation.
Radiation | Intensity | Exposure Time ms | Radiant Exposure |
---|---|---|---|
IPL | 1,500 | 10 | 15, broadband |
fd Nd:YAG laser (e.g., telangiectases) | 500 | 50 | 25 |
Pulsed dye laser (e.g., port wine stains) | 12,000 | 0.5 | 6 |
Q-switched ruby laser (e.g., tattoos) | 25 × 108 | 0.00002 | 5 |
To achieve various therapeutic objectives, physicians use different exposure times of radiation, which range from a few nanoseconds to seconds. In case of cw lasers, the exposure time is in the range of seconds, which correlates simply to the time span of pressing the laser foot switch. In most medical treatments, the exposure time is predefined by the pulse duration of a single laser or IPL pulse. In case of pulsed radiation, the exposure time is equivalent either to a single pulse or to a train of single pulses. The broad range of pulse durations implies a broad range of intensities that is important for safety issues. Therefore, lasers are classified by cw (>0.2 s), pulsed (0.2 s– >1 μs), or giant pulsed lasers (Q-switched lasers) (1 μs–1 ns) in safety regulations.
The most frequently modified parameter in laser treatments is the radiant exposure or fluence. It is the product of light intensity and exposure time (Table 239-2). When taking the total range of medical treatments into account, the radiant exposure varies from about 1–400 J/cm2 for pulsed radiation. This range can be exceeded for cw applications. Table 239-3 shows a few examples for different intensities and exposure times that are employed in the treatment of different skin lesions. It is striking that the values of radiant exposures are within a rather narrow range, whereas the respective intensities and pulse durations vary in an inversely proportional manner by orders of magnitude.
Skin Optics
Propagation of optical radiation through normal media such as air or glass can be described in a straightforward fashion, in particular, for monochromatic and collimated laser light. Reflection, refraction and absorption dominate the propagation. However, when optical radiation penetrates turbid media like skin, we also must consider scattering of radiation inside tissue that hampers light propagation in a complex manner.
When optical radiation strikes skin, part of the photons is reflected back due to the different refractive index of air and skin. This effect necessitates the wearing of safety goggles during laser therapy. Photons inside the skin do not propagate straight on but frequently and abruptly change their direction due to collision with skin constituents, which is called scattering (Fig. 239-4). The mode and the extent of scattering depend on the size of the scattering objects and the wavelength of the radiation. The size of the scattering objects range from a few nanometers (small cell organelles, cell membranes) to a few microns (large cell organelles, cells, collagen) and to a hundreds of microns (hair follicle, sweat glands). The small objects follow the principle of isotropic Rayleigh scattering, the large objects the non-isotropic scattering. In both processes, the extent of scattering decreases with increasing wavelength. With increasing wavelength, photons are less deflected on their path into skin. Thus, the longer the wavelength, the higher is the penetration depth of radiation in skin. Ultraviolet B radiation (around 300 nm) penetrates skin up to a few tenths of millimeters only, whereas infrared radiation (e.g. Nd:YAG, 1,064 nm) achieves a penetration depth of up to a few millimeters. However, the increase in penetration depth with increasing wavelength reverses for wavelengths longer than about 1,100 nm because radiation is increasingly absorbed by water in skin.
Fortunately, the scattering in skin is mainly forward and many photons penetrate the skin. Unfortunately, the scattering changes the beam geometry in the skin and thereby substantially affects the dosimetry. It is complex to determine the number of photons that reach a target (for example, a vessel) in the skin. The higher the number of photons reaching the target, the more heat can be produced inside the target.
The propagation of broadband radiation such as IPL emission is more complex than that of lasers. Photons with different wavelengths in the broadband emission (∼500–950 nm) penetrate skin to different depths. While propagating through the skin, photons can be absorbed by the different chromophores at any time.
Interaction of Radiation with Skin
For monochromatic lasers, photons with single wavelength are absorbed according to the absorption coefficient of the respective chromophores at this single wavelength (Fig. 239-5). The absorption of broadband radiation of IPL is complex (Fig. 239-6). The photons with wavelengths in the range of 500–950 are absorbed to a different extent. This clearly complicates dosimetry of IPL in clinical use. In addition, the different IPL in the market emit radiation with considerable different spectra, even though the same cut-off filters are used. This precludes a comparison of radiation dosimetry for different IPL, which impedes clinical studies.
When monochromatic laser radiation or broadband IPL emission is absorbed by the different skin chromophores oxyhemoglobin, melanin, or water, the radiation energy is converted to either heat or chemical reactions. Nd:YAG laser (frequency doubled at 532 nm) and pulsed dye lasers (585–600 nm) preferentially interact with oxyhemoglobin. Radiation of ruby laser (694 nm), alexandrite (755 nm), and diode laser (around 810 nm) is less absorbed by oxyhemoglobin and more suitable for pigmented lesions including hair removal. Radiation of Nd:YAG laser (1,064 nm) is minimally absorbed by all chromophores. Nevertheless, at high radiant exposures (J/cm2), this laser can be used for unspecific coagulation of tissue (cw mode) or vascular lesions (pulsed mode). The latter is also possible for diode and alexandrite lasers. Infrared lasers such as Er:YAG and CO2 lasers interact solely with water heating up tissue for vaporization or ablation.
In contrast to lasers, IPL emission is broadband and may be absorbed by all chromophores simultaneously. Using a 500 cut-off filter, the radiant exposure (J/cm2) is assembled by photons of different wavelengths (∼500–950 nm), which are diversely absorbed by a skin chromophore like oxyhemoglobin. In addition, these photons reach different depths in skin as explained in skin optics. Thus, the conversion of IPL radiation to heat energy in a target at a certain depth is quite different as compared to laser radiation.
While photochemical reactions require only a small intensity, photothermal reactions necessitates a high intensity. After being absorbed in a chromophore of a specific target (e.g., oxyhemoglobin in a blood vessel), the energy of these photons causes an increase of temperature inside the target. At the same time, the elevated temperature provokes a flow of heat energy to outside the target. This counteracts the heat generation and thereby limits the temperature increase to a maximum value.
For low intensities and long exposure times (pulse duration), the application of laser or IPL radiation causes moderate temperature increase. The temperature ranges from body temperature to 100°C (coagulation), which is achieved with exposure times from milliseconds to continuous wave (cw). When tissue is heated toward the boiling point, radiation causes vaporization of the skin. The use of short CO2 laser pulses heat up tissue very rapidly leading to a rapid vaporization. This effect precisely causes small holes with minimal thermal damage of the adjacent tissue.
The use of sufficient high intensity and pulse duration of microseconds is suitable to perform skin ablation at temperatures higher than 100°C. When using very high intensities (107–109 W/cm2) and very short pulse durations (nanoseconds), the temperature of the target can reach hundreds of degrees celsius. This very short and intense heating may cause explosion of the target. Since the total energy of the laser pulse is limited, ablation and explosion in laser medicine can be restricted to a very small volume. Such high intensities at short pulse durations are accomplished only with lasers and not with IPL.
When pulsed radiation is delivered to skin, the radiant exposure (J/cm2) stays in a narrow range of about two orders of magnitude. In contrast to that, the intensity and the pulse duration may vary with 5 orders of magnitude at least (Table 239-3). As a rule of thumb, we can say that the higher the intensity (W/cm2) and the shorter the pulse duration, the higher the maximum temperature inside the targeted volume. By changing the parameters intensity and pulse duration, the temperature can be adapted to achieve coagulation, vaporization or explosion of a specific target in skin.
It is a major goal to destroy precisely a specific target (such as vessel, pigment) in skin without permanent damage to the adjacent skin structures. This goal can be achieved by applying pulsed radiation and the principle of selective thermolysis.1 To destroy selectively a target by heating, the following issues must be considered. The wavelength of the applied radiation should be preferentially absorbed in the chromophore of the target (Fig. 239-6), which is difficult to accomplish for broadband IPL emission (Fig. 239-7). The energy of the radiation pulse should be high enough to achieve thermal destruction of the entire target.
The pulse duration should be comparable to the thermal relaxation time (tR), which mainly depends on the diameter of the target (D)
where , c, k are thermal parameters of the target).5 The thermal relaxation time is the time it takes to decrease the temperature in the target to 50% of the maximum value. The thermal relaxation time is roughly proportional to the square of the mean diameter of the target. Many of the calculated times match the pulse durations that are used in clinical practice (Table 239-4).
Target | Mean Diameter | Thermal Relaxation Time |
---|---|---|
Pigment (melanosome) | ∼0,1 μm | 5 ns |
Small vessel (e.g., port-wine stain) | ∼50 μm | 1.1 ms |
Hair follicle | ∼0,2 mm | 18 ms |
Large vessel (e.g., leg veins) | ∼1,5 mm | 1,023 ms |
Pigmented lesions containing small melanin or tattoo pigment particles are treated with nanosecond pulses of Q-switched lasers. Besides the short pulse duration, the treatment requires high light intensities to achieve explosion of the pigment particles in the skin.
The use of millisecond pulse duration of either IPL or laser is appropriate to coagulate small blood vessels with diameter of 50–150 μm.6–8 Figure 239-7 illustrated coagulation of a blood vessel in the dermis. Pulsed radiation penetrates the skin and the radiant exposure (J/cm2) is reduced from the known value (J/cm2 at skin surface) to a smaller value due to scattering. The reduced value represents the radiant exposure to be absorbed in the blood vessel. Initially, only the temperature in the vessel increases, coagulating the vessel. Due to the temperature increase inside the vessel, however, heat energy starts to flow to the adjacent dermis. At this point, the radiation pulse should be terminated to achieve selective vessel destruction. Pulse duration longer than the thermal relaxation time results in excessive heat flow to the dermis, which may cause unnecessary adverse reactions.
The thermal destruction of hair follicles is achieved by using radiation pulses in the range of 10–50 ms.9–11 The same range of pulse durations is applied for the treatment of large vessels such as leg veins.12 However, the calculated value (Table 239-4) does not match this range. In case of voluminous targets, the selective photothermolysis must be aligned to a new parameter, which is the cooling down of heated target. The larger the target, the slower is the cooling down and hence the higher the probability of effective coagulation.12
Laser radiation is usually applied on the skin surface as a circular spot showing spot sizes in the range of millimeters. The use of fractional photothermolysis leads to spatially distributed zones of microthermal injury within the treatment area, called microscopic treatment zones (MTZs). These are generated in the skin by focused laser irradiation. MTZs consist of sharply confined tissue denaturation with a diameter of about 100 μm that is surrounded by viable tissue, at intervals of about 200 μm.13 MTZs are produced either in the ablative or in the nonablative mode. Fractional photothermolysis is a new concept for skin restoration. The MTZs are placed not manually but using computer-assisted scanner device to produce a periodic grid of thermal injuries.
Laser therapy of skin disorders require high radiant exposure to be delivered to the skin surface. Most of the targets are located at the epidermal-dermal junction or within the dermis. To reach these targets, the photons have to traverse the epidermis, which can partly absorb the incoming radiation. In addition, excess heat, caused by the absorption of radiation in the dermis, may provoke damage of dermal and epidermal structures. To avoid unwanted thermal damage especially to the epidermis, the skin surface is frequently cooled before, during, and after laser impact by a variety of measures.
The simplest and cheapest cooling is accomplished by applying ice cubes on the skin surface prior to or even during laser impact.14 An application time of 10 seconds can result in skin surface temperature of about 10°C. About the same results are achieved by applying actively cooled metal or glass to the skin surface.15 Instead of contact cooling, some laser systems are equipped with spray cooling. Shortly before laser impact, a burst of cold gas (usually tetrafluoroethane) is applied to skin surface reducing the temperature of the epidermis to about 5°C.10 Cooling is also achieved by exposing the treatment area to cold airflow that is provided by a special air conditioner.
Safety
It was recognized early that radiation of any wavelength can pose a risk to humans, in particular, to unshielded eyes and skin.16,17 This risk is caused by the absorption of photons leading to photochemical or photothermal alterations of tissue.18,19 These alterations may affect tissue integrity for a short period of time or damage tissue permanently. When lasers or intense pulsed light sources are used to treat skin lesions, a variety of safety measures are required.
The use of lasers in medical care is governed by rules and standards that are established in the Safety of Laser Products of the International Electrotechnical Commission (IEC) (http://www.iec.ch/) or American National Standards Institute (ANSI) (http://www.ansi.org/). The safety rules are based on IEC 60825-1 or ANSI Z136.1 for all laser systems, including medical lasers. Lasers have been classified in four groups based on the accessible emission limits (AEL). The rules are applicable to safety of laser products emitting laser radiation in the wavelength range 180 nm to 1 mm. These regulations unfortunately do not cover the use of IPL; there is no existing FDA performance standard for IPL products. We provide some advice regarding IPL safety, based on comparisons with laser systems. IPL systems emit high radiant exposures (J/cm2) that are comparable to lasers and have therefore the potential to cause substantial damage to the eyes and skin.
When using lasers for tissue vaporization, laser plume must be evacuated by using an appropriate device to avoid contact with infectious material.
The high radiant exposures, high intensities and short pulse durations in laser therapy not only can harm the skin but also can severely damage the eyes even causing complete loss of eyesight. The side effects of radiation to the eyes are comparable to those effects at the treatment site. It is inevitable that radiation partly reaches the eyes due to reflection and scattering at the treatment site. Other reasons are accidental and careless handling of lasers and IPL during treatment.
In the spectral range of about 370–1,000 nm, radiation readily penetrates the cornea and the lens reaching the choroid and retina. The radiation of this spectral range is well absorbed by the hemoglobin in the choroid vessels that may lead to thermal damage of the retina. Refraction by the lens and cornea increases the intensity of the incoming radiation by several orders of magnitude.
For wavelengths shorter than 370 nm and longer than 1,000 nm, radiation is predominantly absorbed in water (Fig. 239-1) and do not completely traverse lens and cornea. The short wavelengths can cause injury of the cornea (cornea ablation) or cataract formation in the lens. With increasing wavelengths above 1,000 nm, the penetration of radiation decreases leading again to cataract formation (Fig. 239-3). For wavelengths around 3 μm, the water absorption is maximal for causing cornea ablation. At even longer wavelengths, vaporization of the cornea may occur.
The eyes try to protect themselves from damage that could be induced by excess radiation energy. The eyelid is closed automatically in case high radiation energy is detected. However, the corresponding time span is about 250 ms, which is longer than most of the pulse durations used for lasers and IPL. Radiation that is invisible for the eyes (UV, IR) will not trigger an eye blink.
Users of lasers or IPL should always keep in mind that even small intensities reaching the open pupil can cause severe damage of the retina, which, in most cases, is irreversible and may entail a complete loss of vision. Radiation of lasers or IPL can also damage skin outside the treatment area.
For nearly all medical laser systems, even scattered radiation can be dangerous to eyes. To shield the eyes, it is mandatory to wear safety goggles during treatment with lasers or IPL according to EN 207 in Europe or to ANSI Z136 in the United States. To achieve maximal safety for the eyes, it is important to adjust the optical filters of the safety goggles to the radiation source used (laser or IPL). The important parameters of the radiation sources are wavelength, pulse duration, intensity, and radiant exposure. These parameters of a laser or IPL system determine the characteristics of the safety goggles. Each laser or IPL requires special safety goggles that are labeled for their use, which are wavelength range of protection, laser mode, and scale number of protection. The manufacturer of lasers or IPL must provide sufficient information about the appropriate safety goggles, which can be found in the handbook of each laser or IPL device.
All laser systems, including the medical lasers, sold in Europe must be certified to EN 60825-1. In the United States, several organizations concern themselves with laser safety including ANSI and the Center for Devices and Radiological Health (CDRH). There are some basic categories of controls useful in laser environments. These are engineering controls, personal protective equipment, administrative and procedural controls, special controls, and correct labeling of the laser products. It is required and mandatory to appoint a laser safety officer (LSO). This person has the authority to monitor and enforce the control of laser hazards and effect the knowledgeable evaluation and control of laser hazards. The LSO administers the overall laser safety program where the duties include items such as confirming the classification of lasers and ensuring that the proper control measures are in place, approving substitute controls and conducting medical surveillance. The LSO should receive detailed training including laser fundamentals, laser bioeffects, exposure limits, classifications, control measures (including area controls, eye wear, barriers, etc.), and medical surveillance requirements. Nearly all medical lasers are assigned to class III or class IV. The class of a laser can be found on the label present on the laser device and in its handbook. Class III means moderate power lasers (cw: 5–500 mW, pulsed: 10 J/cm2 or the diffuse reflection limit, whichever is lower). In general, Class IIIB lasers will not be a fire hazard, nor are they generally capable of producing a hazardous diffuse reflection. Specific controls are recommended. Class IV means high power lasers (cw: >500 mW, pulsed: 10 J/cm2 or the diffuse reflection limit) are hazardous to view under any condition (directly or diffusely scattered) and are a potential fire hazard and a skin hazard. Significant controls are required of Class IV laser facilities (http://www.osha.gov/dts/osta/otm/otm_iii/otm_iii_6.html). When using medical lasers, it is unavoidable that part of the beam path from a Class IIIB or Class IV laser is not sufficiently enclosed and/or baffled to ensure that radiation exposures will not exceed the MPE. Then a “laser-controlled area” is required. Although recommendations for the safe use of IPL in medical practice are currently not available, many of the items mentioned for lasers can be applied as well for IPL, in particular regarding eye protection.
Therapeutical Application
Since the introduction of lasers in dermatology by L. Goldman20 and the development of selective photothermolysis in the 1980s,1 laser therapy has become an indispensable therapeutic modality and more than 80 indications are documented.
PWS are asymmetric usually congenital capillary vascular lesions present in up to 2% of newborns. In childhood, they are pale red macules and patches. In adults they tend to become darker (port wine) and develop an irregular surface sometimes with blue-red papules and nodules. PWS are probably secondary to abnormal vascular innervation leading to a reduced vascular tone.21–26
In hypertrophic PWS with nodular surface, besides the vascular abnormalities extensive epithelial, neural, and mesenchymal harmartomatous changes have been observed suggesting a genetically determined multilineage development field defect.27
More recent studies indicate that RASA1 mutations cause capillary and arteriovenous malformations and hereditary capillary malformations and limb enlargement without arteriovenous malformation.28–30
Vascular endothelial growth factor (VEGF) and VEGF-receptor 2 expression is significantly increased in PWS compared to controls, indicating that VEGF and VEGF-R2 may contribute to vessel proliferation and vasodilatation.31
PWS are often associated with other malformations (Sturge-Weber-Krabbe syndrome, Cobb syndrome, Klippel-Trenaunay syndrome, Parkes-Weber syndrome).
Acquired PWS are rare but may be caused by trauma, hormonal imbalances or medications.
The introduction of the pulsed dye laser (PDL) in the 1980s (λ = 585 nm, t = 0,45 ms, Ø = 5 mm) greatly improved the treatment options for PWS. In the past two decades, important advances include the use of wavelengths of 585–600 nm, longer exposure times and increasing the diameter of the beam. In addition cryogen spray cooling is used to protect the epidermis, allowing a higher fluence to be delivered to the dermis.
Standard parameters for treating a PWS are a wavelength between 585 and 600 nm, a fluence of 4–20 J/cm2, a pulse duration of 1.5–10 ms, a beam diameter between 7 and 10 mm and cryogen cooling of the skin surface, also known as dynamic cooling device (DCD). Larger beam diameters require a lower fluence. In some studies, longer wavelengths produced better results than 585 nm.32–34
The results depend on the age of the patient, the location, the color of the PWS and the number of treatment sessions.35,36 The success rate for children and adolescents is around 65% with the best results obtained when treatment is started in the first year of life (Fig. 239-8). Reyes and Geronemus37 treated 73 children less than 14 years old; 45% got over 75% lightening and 42% obtained 50% improvement. A complete disappearance can be expected in only 15% of patients. In a prospective study by van der Horst et al38 the benefit of early treatment could not be confirmed, although these authors did not employ a laser system with dynamic cooling.
The location of a PWS is also a significant factor. PWS on the face and back respond much better than those at other sites. Those on the legs respond worst. Even on the face, there is considerable variation. PWS in the midface and distribution of the second trigeminal branch lighten less than those in the periorbital region, forehead, neck and nape.39–41 The size also affects the response. Small lesions respond better than large ones, and, often in the latter case, uniform lightening is impossible to achieve.42,43 Repeated treatments lead to an improved response, and sometimes more than 10 sessions may be required.
The risk of scarring with PDL is quite low, around 1%. It is higher in patients on isotretinoin therapy. The main complications are hypo- or hyperpigmentation and rarely the development of a pyogenic granuloma.44–47 After the treatment is concluded, up to 15% of the patients may develop partial recurrences of their PWS, but not to the same extent and size as prior to treatment.48–50
When PWS are resistant to PDL, there are other laser options. Deeper vessels can be treated with the alexandrite laser (755 nm) and the pulsed Nd:YAG laser (1,064 nm).51–54 McGill et al were able to produce additional improvement in 10 of 18 patients treated with PDL with an alexandrite laser.55
In a study comparing pNd:YAG laser and PDL, both systems produced 50%–75 % improvement. Patients preferred the Nd:YAG laser because it caused less purpura. On the other hand, the risk of scarring was clearly higher with the pNd:YAG laser, which should be used for PWS only by experienced laser therapists.
KTP lasers can also produce improvement in PSW but the results are worse than with PDL and the risk of scarring is greater.56
There is also good experience using IPL to treat PWS.3,57,58 In a comparison study in 20 patients, both PDL and IPL produced improvement but the extent of lightening was significantly better with the PDL.59 In contrast, in our own study, IPL was slightly superior to PDL.60
In individual patients with a nodular component to their PWS, vaporization of the individual lesions with the cwCO2 laser has proven helpful.
Hemangiomas are benign proliferative tumors, which occur in about 5% of children. They are more common in premature infants and following amniocentesis. In general, they are not present at birth but appear in the first weeks of live as pale or telangiectatic macules, which then rapidly develop into nodular tumors. After a growth period of 9–10 months, there is a stable period, followed by a regression phase that can last until 10 years of age. About 50% of patients have residual lesions such as scars, fibrofatty nodules, or telangiectases. Complications of hemangiomas include ulceration, bleeding, and distortion of orifices (nose, eyes, mouth, and anogenital region). We distinguish between superficial, cutaneous-subcutaneous, and deep subcutaneous hemangiomas. They may be circumscribed nodules, plaques in a segmental distribution or multiple. The tumors cells express FCR II (CD32), merosin, and GLUT1, a pattern similar to placental cells.
Congenital hemangiomas are much less common; they are divided into noninvoluting congenital hemangiomas (NICH) and rapidly involuting congenital hemangiomas (RICH).
The treatment of hemangiomas is controversial, as most of them regress spontaneously.36 Nonetheless, numerous studies have shown that small and initial hemangiomas respond very well to PDL (Fig. 239-9). Growth is arrested in over 60% of treated tumors with just a few PDL sessions: only 15% disappear completely.61–64 Ulcerated hemangiomas also respond well to laser therapy; they become lighter and re-epithelize quicker.65 Deep subcutaneous components of the hemangiomas do not respond.66
In a prospective randomized and controlled study, 121 children with uncomplicated hemangiomas were treated with PDL (585 nm, pulse duration 0.45 ms, spot size 3–5 mm, fluence 6-7.5 J/cm2, no cooling). After a year 30% of the lesions in the treated group had completely disappeared; in the control group, it was only 5%. Residual changes were found in 42% of the treated children and 44% of the control group. Complications were much higher in the treated group with 45% versus 15% in the controls. In the treated group, the hemangiomas had increased in size by 61%; in the control group, 160%.67
In another study, a PDL with a longer pulse duration and surface cooling (595 nm, 7-mm spot size, pulse duration up to 20 ms, 9–15 J/cm2 fluence) was compared to a standard PDL without cooling (585 nm, 7 mm, 0.45 ms, up to 7 J/cm2); treatment with the longer pulse time led to better results with fewer side effects.68
Because of the introduction of propranolol to treat infantile hemangiomas, it is necessary to reexamine the role of lasers in this problem. Cutaneous-subcutaneous hemangiomas and rapidly proliferating hemangiomas in complicated locations respond well to systemic propranolol. They are no longer an indication for cwNd:YAG laser or intralesional laser therapy with bare fibers. Propranolol can be combined with PDL.69,70
Residual telangiectases after the regression of hemangiomas can be improved with either PDL or IPL.
Telangiectases are permanently dilated small venules, which may be solitary or matted together to form larger flat lesions. Primary or essential telangiectasia is often familial, but no underlying cause has been found. Special forms are progressive, essential disseminated telangiectasia, which increase in numbers on lesions on the extremities, trunk and face, as well as unilateral nevoid telangiectasia syndrome (UNTS).
Telangiectases are a good indication for laser and IPL therapy. PDL with large beam size and midrange fluences are employed; the pulses are set to lightly overlap. Many patients consider the resulting purpura after PDL as inacceptable. Pulse stacking has been tried, using a series of impulses all beneath the purpura threshold to reduce problems. The number of sequential impulses is based on the visible vasoconstriction. Using these techniques, good clinical effects can be obtained without increasing the complication rate.71 In a comparative study, long-pulsed PDL proved more effective than two IPL devices.72
KTP lasers also produce good results for this indication.73,74 Infrared laser such as diode lasers or the pulsed Nd:YAG laser can also be used. In a comparison study, a 940-nm diode laser was more effective than a KTP laser.75
The pulsed Nd:YAG laser is best suited for vessels with a diameter of more than 1 mm; the treatment is relatively painful and the risk of scarring greater than with other lasers.77,78
When telangiectases are grouped together and more diffusely red, IPL can be very effective (Fig. 239-10). A major advantage is that the large rectangular impulses can be very precisely placed next to each other.
Poikiloderma of Civatte is also a good indication for PDL and IPL; we prefer the latter.79
A phlebology evaluation is required prior to treating these venous problems. Vessels with a diameter of up to 1 mm are a good indication for PDL with a variety of wavelengths (585, 590, 595, and 600 nm) and a longer pulse duration. For this purpose, oval handpieces with a spot size of 2 × 7 mm are available. Fluences between 12 and 20 J/cm2 are used, and skin cooling is mandatory; despite these measures, sometimes hyperpigmentation cannot be avoided.80,81
The alexandrite laser is well established for treating starburst veins. Using relatively high fluences (60–80 J/cm2) and pulse durations of 3 ms with surface cooling, 65% of patients experienced an improvement of at least 75% when the vessel diameters were between 0.3 and 2 mm. Hyperpigmentation developed in 35%.82 In a study comparing alexandrite, diode, and pulsed Nd:YAG lasers for starburst veins with a caliber of 0.3 and 3 mm, the Nd:YAG laser was most effective.83
Treatments with a 940-nm diode laser produce greater than 75% improvement in 46% of patients.84,85 For vessels greater than 1 mm in diameter, the pulsed Nd:YAG laser with surface cooling is very effective.77,83,86–88 In direct comparison studies, the pulsed Nd:YAG laser was just as effective as sclerotherapy.89–91
A new use for lasers in phlebology is sealing the great saphenous and small saphenous veins with endoluminal laser therapy. Diode lasers, Nd:YAG lasers, and radio frequency ablation devices have all been employed. In a prospective randomized study, radiofrequency ablation produced just as good results as operative vein stripping.92–95
The superficial component of congenital or acquired lymphangioma circumscriptum can be vaporized with the CO2 laser. Although improvement is produced, sometimes for many years, the lesions eventually recur.96–99
Venous lakes respond well to treatment with PDL KTP, diode or Nd:YAG lasers.100
Low-flow venous malformations can be treated with cw Nd:YAG or long-pulsed Nd:YAG lasers or with the intralesional bare fiber technique.101–104
Small angiofibromas in tuberous sclerosis can be treated with the KTP laser. When multiple lesions are being treated, then ablative approaches such as a flash scanned CO2 laser or Er:YAG laser are preferred.105–110
Cherry angiomas respond well to KTP laser and PDL.111 The pulsed Nd:YAG laser can also be used.
The ectatic vessels of hereditary hemorrhagic telangiectasia (Osler-Rendu-Weber syndrome) are best treated with the KTP, diode, or long-pulsed Nd:YAG lasers. The pNd:YAG laser can also be used for larger ectatic vessels but the treatment is painful.112
The matted telangiectases in angioma serpiginosum respond well to PDL and IPL therapy, but recurrences are common.113
Xanthelasmas and Syringomas can be coagulated with a KTP laser114 or destroyed with the cw-CO2 laser; there is a certain risk of scarring. Better choices, because they cause less thermal damage, are pulsed or flash scanner-CO2 laser systems. Another reliable approach is the very exact ablation with the Er:YAG laser.115,116
Trichoepitheliomas are usually skin-colored, so that coagulation with a semiselective coagulating laser is not very helpful. Ablation with the CO2 or Er:YAG laser makes it possible to obtain good cosmetic results even when treated multiple aggregated lesions.117–119
Neurofibromas can also be removed with the CO2 laser if they are not too large. Using relatively high power, the dome-shaped neurofibroma is circumferentially incised around its base; then the neural tissue generally herniates up with a little lateral pressure, so it can be vaporized or destroyed at its base. Although large deep defects may result, they heal amazingly well.120
Eruptive vellus hair cysts can be vaporized with the CO2 laser. In patients with steatocystoma multiplex, one can open the cysts with a focused CO2 laser beam, express the contents, and then coagulate the cyst wall with a defocused beam. Cosmetically pleasing results are not always possible.121
Similarly, a digital mucous cyst
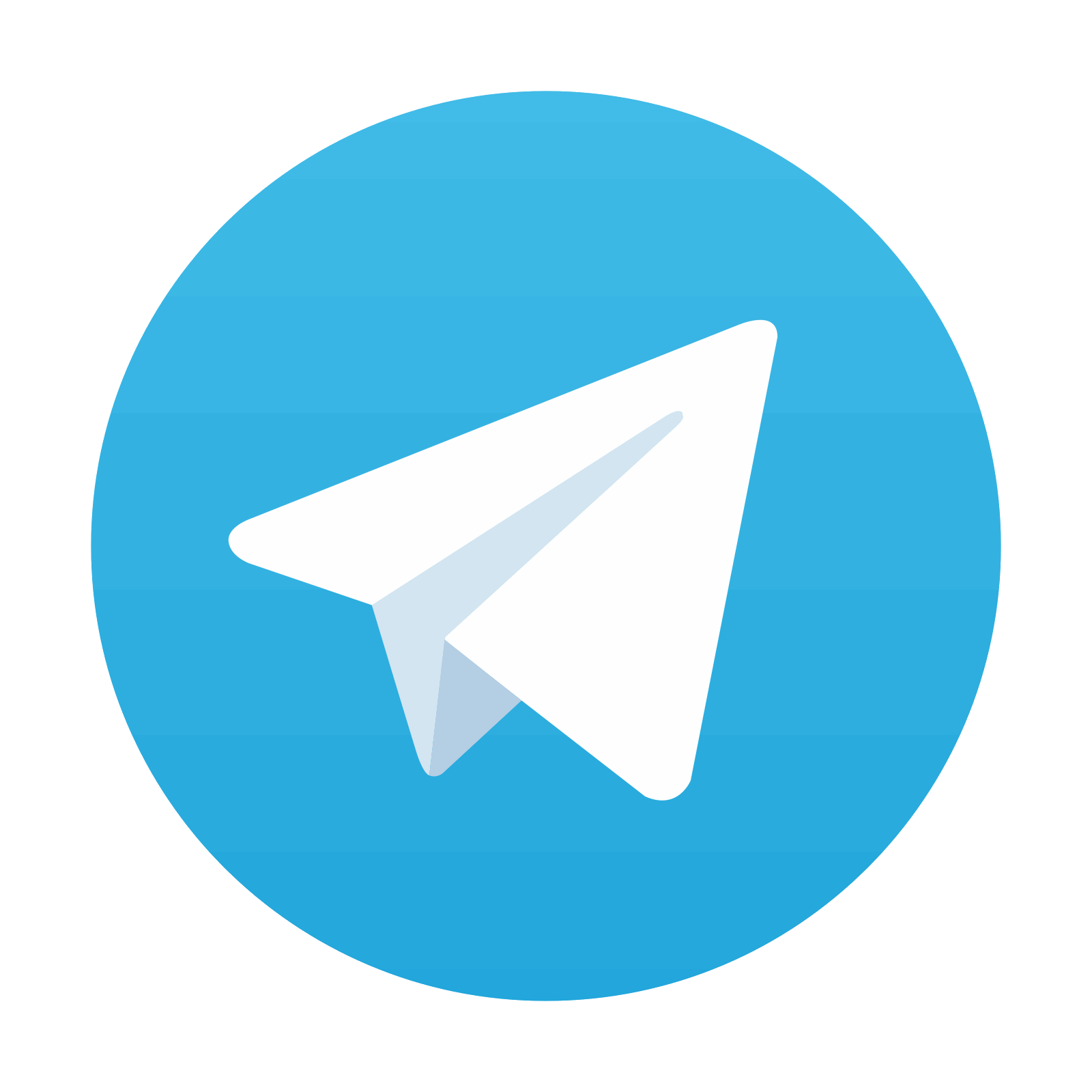
Stay updated, free articles. Join our Telegram channel

Full access? Get Clinical Tree
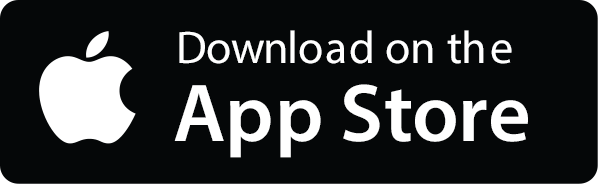
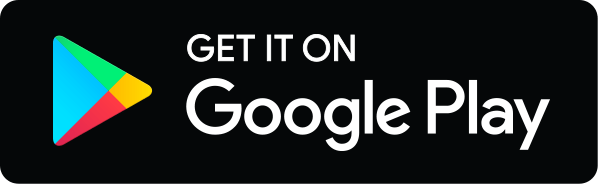
