Chapter 46 Intratunnel Tibial Fixation of Soft-Tissue Anterior Cruciate Ligament Grafts
Graft Sleeve and Tapered Screw
Introduction
Tibial fixation of soft-tissue anterior cruciate ligament (ACL) grafts remains challenging.1–5 Tibial fixation is challenging because of the lower bone mineral density of the proximal tibia, the fact that tibial fixation devices must resist shear forces applied parallel to the axis of the tibial bone tunnel, and the longer time required for soft-tissue grafts to heal within the bone tunnels.2,6 Tibial fixation of soft-tissue grafts using screws and ligament washers that anchor to the tibial cortex can address many of these issues; however, these implants are often prominent and often cause local skin irritation and pain, requiring a second operation for removal.5 Intratunnel tibial fixation of soft-tissue grafts using interference screws avoids the problem of prominent hardware; however, laboratory biomechanical studies have shown that the tensile properties of soft-tissue grafts fixed with interference screws are highly dependent on bone mineral density, and this fixation technique often results in low initial fixation strength and slippage under cyclical loading.2,4,5 The Graft Sleeve and Tapered Screw (GTS) System (Smith & Nephew Endoscopy, Andover, MA) intratunnel tibial fixation technique was developed to increase the failure load and stiffness and to decrease slippage of four-strand soft-tissue grafts.
The GTS System is an intratunnel tibial fixation technique that positions a poly-L-lactic acid (PLLA) tapered, fine-pitch screw concentrically within a four-strand soft-tissue graft. The tapered screw features shorter thread distance, which enhances compression of the soft-tissue graft in cancellous bone. The GTS System consists of the tapered screw and a three-lumen, woven, nonabsorbable polypropylene (PPE) mesh graft sleeve that organizes the four-graft strands in the tibial tunnel. The graft sleeve prevents graft twisting during screw insertion; maximizes bone–tendon contact, which enhances healing; and provides better compression of each ligament strand against the bone tunnel wall while protecting the graft strands from screw damage (Fig. 46-1).
Basic Science
Biomechanical Testing
Biomechanical testing of the graft sleeve and tapered screw using human doubled gracilis and semitendinosus tendon (DGST) grafts has been performed in the proximal tibia of calf bone (2 years or younger) with bone mineral density similar to that of the proximal tibia in young humans.1,7 The tibia–DGST–graft sleeve complex was subjected to a 50N preload followed by cyclical loading between 50N and 250N at 1 Hz for 1000 cycles with the direction of tensile loading applied parallel to the axis of the tibial bone tunnel. Graft slippage was measured using a noncontact, three-camera, motion analysis system that allowed the position of retroreflective bone and graft markers to be recorded in three dimensions during cyclic loading. Mean graft slippage, in which graft slippage was defined as the change in position of the tendon marker relative to the bone marker under the 50N preload and after cyclical loading, was 1.14 ± 0.83 mm. The mean linear stiffness was 158 ± 31 N/mm, and the mean failure load was 736 ± 162 N, in which failure load was defined as the load when the load displacement curve substantially deviated from linear. The predominant mode of failure (60%) was pullout of the DGST tendons, graft sleeve, and tapered screw from the tibia. Comparison of the GTS System and the IntraFix Tibial Fastener (DePuy Mitek, Norwood, MA) using the just-mentioned testing protocol demonstrated no statistically significant differences in the cyclical and failure properties between the two devices.
Biocompatibility and Histology of Fixation Site Healing
Biocompatibility of the PLLA screw and PPE graft sleeve has been studied in a sheep model.8 Gross histological examination demonstrated that implantation of the PLLA screw and polypropylene graft sleeve had no adverse effect on the articular cartilage. Microscopic analysis of the synovial fluid using polarized light failed to detect the presence of any polymeric debris. Signal intensity at the bone–tendon interface evaluated by computed tomography (CT) was used as an indicator of bone–tendon healing. CT slices in the axial plane demonstrated a progressive increase in healing at the bone–tendon interface over time. By 12 weeks a neocortex surrounded the tendon grafts. Based on the appearance of the signal intensity at the bone–tendon interface, the presence of the graft sleeve and tapered screw did not interfere with healing of the tendon to bone. No adverse reactions were noted related to the PLLA screw and PPE graft sleeve. Histological examination performed using a hard tissue technique in polymethylmethacrylate (PMMA) demonstrated that the PLLA screw compressed the tendons directly against the bone tunnel wall. These sections showed correct positioning of the PLLA screw in the PPE sleeve, as well as organization of the tendons in the PPE sleeve and bone tunnel to give a maximum bone–tendon interface (Fig. 46-2).
Healing at the bone–tendon and tendon–screw interface was evaluated on paraffin sections stained with hematoxylin and eosin (H&E) and trichrome stains. Bone–tendon healing progressed over time and was observed in all specimens. The tendon grafts were compressed against the adjacent bone as a result of the screw being placed in the central lumen of the graft sleeve. The bone–tendon interface was composed of loose connective tissue, fibroblastic cells and local areas of bone–tendon integration. There was no evidence of macrophage or foreign body reaction at the bone–tendon interface (Fig. 46-3). Connective tissue was noted to infiltrate throughout the PPE sleeve, and no significant difference was seen between areas where the sleeve and screw were present and areas in the proximal part of the bone tunnel where only the tendon grafts were present. The interface between the tendon graft and screw was composed of a thin layer of loose connective tissue with cellularity. There was no evidence of macrophage or foreign body reaction at the tendon–screw interface. In conclusion, histological analysis demonstrated the following:
Surgical Technique
Advantages of the Graft Sleeve and Tapered Screw
Compared with other intratunnel soft-tissue tibial fixation techniques, the advantages of the graft sleeve and tapered screw include consistent concentric insertion of the tapered screw, high screw insertion torque, uniform compression of the four-strand soft-tissue graft into the bone tunnel walls, and minimal rotation of the graft strands during screw insertion. Laboratory biomechanical testing has shown that these properties enhance initial graft fixation strength, stiffness, and resistance to slippage under cyclic loading.7,9 Finally, optimal surgical technique for implantation of the graft sleeve and tapered screw incorporates use of a mechanical tensioning device that equally tensions all four strands of the soft-tissue graft. Hamner et al10 have demonstrated in a laboratory biomechanical study that equal tensioning of all four strands of a four-strand hamstring tendon graft is necessary to maximize initial tensile strength and stiffness of the DGST graft.
Tibial Tunnel
A tibial tunnel length of 40 to 50 mm is optimal because this length range will allow the 30-mm-long tapered screw to be inserted flush with the tibial cortex, with there being no possibility that the screw will protrude into the intraarticular aspect of the knee joint. Setting the adjustable tibial aimer between 50 and 55 degrees will usually allow these tunnel lengths to be achieved. If the transtibial tunnel technique is used to drill the femoral tunnel, the starting position of the tibial guide pin must be located adjacent to the anterior fibers of the medial collateral ligament (MCL). This is necessary to achieve the correct tunnel angulation so that the femoral tunnel can be oriented at the 10-o’clock position. Laboratory biomechanical studies have demonstrated that single-tunnel ACL grafts placed at the 10-o’clock position provide better rotational control compared with ACL grafts placed at the 11-o’clock position.11 The starting location of the tibial guide pin is not critical if the femoral tunnel is drilled using the anteromedial portal technique.
A tight fit of the soft-tissue ACL graft in the bone tunnels is desirable to optimize tendon–bone healing.12
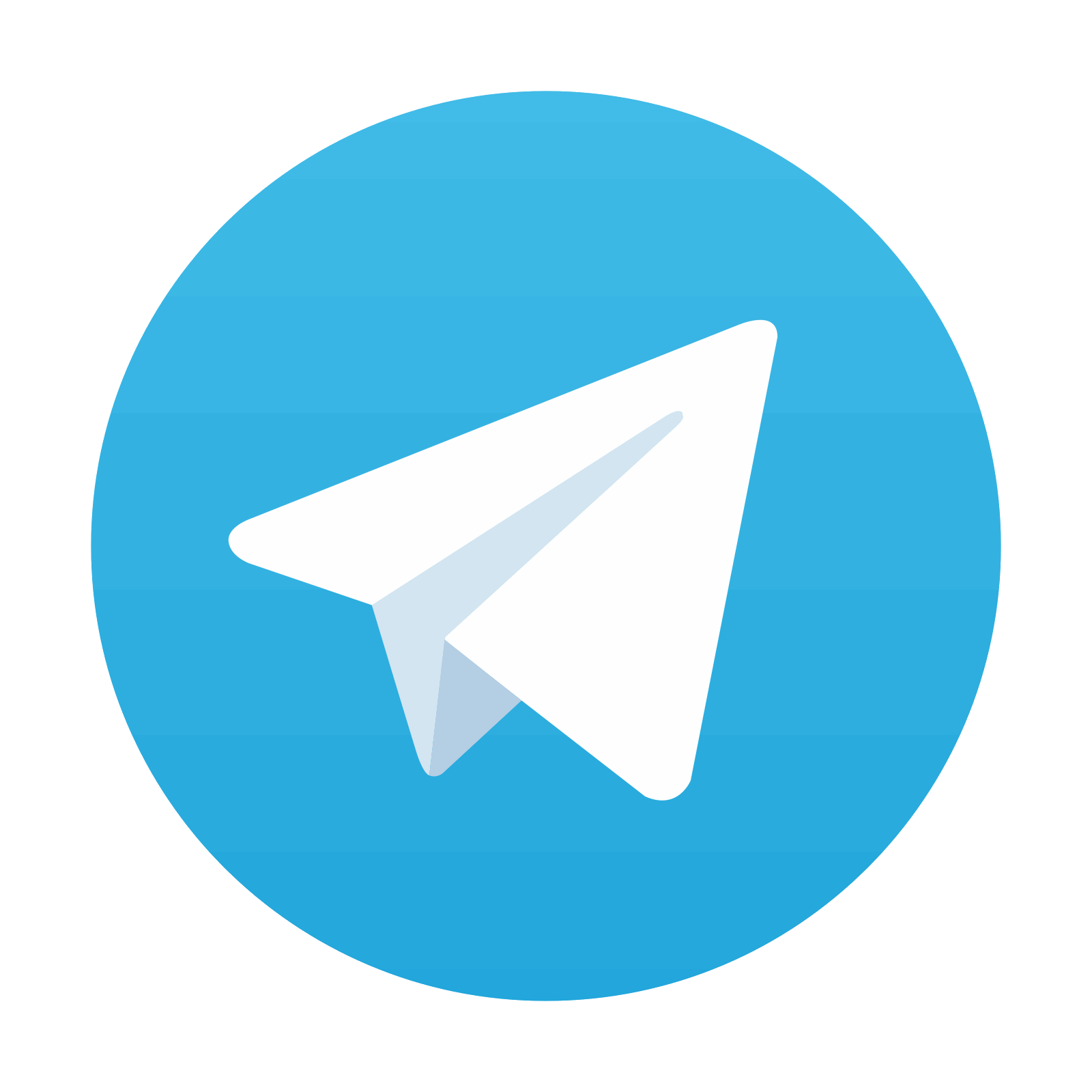
Stay updated, free articles. Join our Telegram channel

Full access? Get Clinical Tree
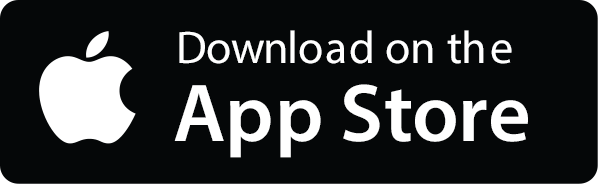
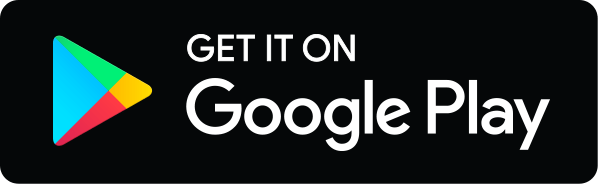