© Springer-Verlag Berlin Heidelberg 2014
Melvin A. Shiffman, Alberto Di Giuseppe and Franco Bassetto (eds.)Stem Cells in Aesthetic Procedures10.1007/978-3-642-45207-9_5555. Interactions Between Adipose Stem Cells and Cancer
(1)
Department of Plastic Surgery, Adipose Stem Cell Center, University of Pittsburgh, 3550 Terrace St., 497 Scaife Hall, Pittsburgh, PA 15213, USA
(2)
Department of Plastic Surgery, University of Pittsburgh, 3550 Terrace St., 497 Scaife Hall, Pittsburgh, PA 15213, USA
55.1 Introduction
Aesthetic deformity is an unavoidable consequence following mastectomy or wide local excision in breast cancer patients for which tissue flap- and implant-based procedures have become the standard of care. An alternative approach that is in keeping with the plastic surgeon’s philosophy of replacing “like with like” is autologous fat grafting which holds the potential to allow for a more natural-appearing reconstruction. The concept is not new and was reported as early as 1895 by Czerny [1] for the correction of a postmastectomy defect. However, concerns regarding complication rates (fat necrosis [2], fibrosis, calcification) and interference with breast cancer surveillance led to recommendations against the procedure in 1987 by the American Society of Plastic and Reconstructive Surgeons (ASPRS) Ad-Hoc Committee on New Procedures [3]. Consequently, for many years fat grafting to the breast remained controversial. More recently, improvement in surgical and radiographic techniques has been accompanied by a renewed interest in breast fat grafting, with studies now demonstrating the ability to adequately screen for breast cancer after treatment [4–9].
In the past decade, adipose biology has been highlighted due to the discovery of a multipotent mesenchymal stem cell (MSC) population, deemed “adipose-derived stem cells” (ASCs), which captured the imagination of the scientific community and public alike. Successful fat grafting has in part been attributed to their contribution to adipogenesis and angiogenesis through either direct differentiation or paracrine-mediated effects [10–14]. In fact, several clinical studies in which lipoaspirate was enriched for these ASCs prior to injection have reported enhanced volume retention using this approach [4, 15, 16]. Furthermore, ASCs appear to be responsible for the dermatologic improvement that has at times been noted in fat-grafted breasts that had previously suffered extensive scarring or radiation damage [17, 18]. Certainly, the regenerative properties of ASCs make them a valuable resource which could be used to further improve the clinical outcomes of fat grafting procedures.
The beneficial properties of stem cells also create concerns over safety for fat grafting in breast cancer survivors. The implications of introducing ASCs into previous sites of cancer on local or distant recurrence are unclear, but foreseeable risks include ASC transformation into cancer stem cells and ASC-cancer interactions leading to enhanced tumor growth or metastasis. This chapter will review some of the findings from major in vitro, in vivo, and clinical studies related to this topic in an attempt to summarize the current understanding of ASCs/whole fat and its role in the setting of breast cancer.
55.2 The Risk of ASC Maldifferentiation
Concerns regarding ASC maldifferentiation into breast cancer stem cells are twofold. Firstly, if a therapeutic regimen requires extensive tissue culture expansion of ASCs in order to obtain adequate cell numbers for successful treatment, there may be selection for rapidly dividing cells, which in turn generates the risk of accumulating the genetic and epigenetic alterations necessary for tumorigenesis. Indeed, there have been reports from several laboratories of mesenchymal stem cells derived from adipose tissue or bone marrow undergoing immortalization after multiple in vitro expansions [19–21]. However, the issue may be inconsequential in that clinically relevant passage numbers are far lower than that which was needed for transformation to occur, and in the case of ASCs, their relative abundance makes it possible to obtain therapeutic yields without the need for in vitro expansion [22]. Furthermore, some of these reports were retracted due to DNA fingerprinting studies, which revealed cross-contamination with cancer cell lines. In addition, a much greater body of evidence suggests that such a phenomenon does not occur in human MSCs. Therefore, ASC maldifferentiation in vitro does not appear to be a major concern [23–30].
Alternatively, therapies that introduce additional stem cells could potentially increase cancer risk by providing additional targets for cancer stem cell transformation in vivo. Such a risk would be relevant to therapies involving in vitro expanded ASCs or those involving non-autologous sources of ASCs. It has been suggested that the association between increased breast cancer risk and high levels of growth hormone signaling during key periods of mammary development may be related to IGF-1-mediated upregulation of mammary stem cell proliferation. Despite the plausibility of this proposal, as of yet there is no direct evidence to show that increased mammary stem cell number confers an increased lifetime risk of developing breast cancer [31, 32]. Additionally, given the multiple genetic hits that must amass over an individual’s lifetime in order for carcinogenesis to occur and the epidemiological data demonstrating the majority of new breast cancer cases (~78 %) occurring in women aged 50 years or older, the risk of increasing overall ASC count in the former breast cancer patient may be negligible.
55.3 Interactions Between ASCs and Breast Cancer
The more pertinent concerns of ASC therapy in the setting of breast cancer are related to the tumor stroma, which insulates the tumor from normal host tissue. Over the years, this tumor microenvironment has gained increased recognition as a critical component in the development and progression of cancer. MSCs comprise one of the major cell populations situated in this milieu and appear to have an active role in this process. However, their origin or exact role in the breast cancer niche is not well defined [33–37]. Tumor tropism studies involving both BM-MSCs and ASCs strongly suggest an interaction between MSCs and cancer cells [38–43]. Although investigations attempting to delineate these interactions are ongoing, whether this cross talk incurs a risk of cancer recurrence in the former breast cancer patient is uncertain at this time.
55.4 In Vitro and In Vivo Studies
Before translating findings from basic science to patients, it is important to verify that the experimental model accurately depicts the clinical question at hand, which is the safety of ASC-based breast reconstruction in the postmastectomy or post-lumpectomy patient. Given the heterogeneity of various types of cancer, it would be imprudent to draw conclusions from studies using cancers other than those of the breast. This has been highlighted by a number of studies that in combination suggest whether ASCs in the setting of cancer will lead to tumor promotion, inhibition, or no perceivable effect may in part be related to the particular cancer type [44–48]. Additionally, while bone marrow mesenchymal stem cells (BM-MSCs) have been more extensively studied in the setting of cancer, extension of findings from BM-MSC-based studies for ASCs must be done with caution. Despite the phenotypic and functional similarities that have been observed between these MSCs, specific differences at the transcriptional and proteomic levels can be found [49, 50]. Finally, the route of ASC administration must be taken into consideration as well given the reports suggesting that this may also be a factor affecting tumor response [39, 51]. The following studies were selected because in vitro findings were tested in an animal model in which MSCs were delivered subcutaneously into an orthotopic site and, with one exception, all specifically involved breast cancer cells and ASCs.
55.5 Murine ASC Breast Cancer Model
One of the seminal works in this area was that performed by Muehlberg et al. [40] in which enhanced tumor growth and metastasis in the presence of ASCs was demonstrated in a 3-week immunocompetent mouse model using the murine 4T1 breast cancer cell line and ASCs isolated from the perirenal and pelvine fat tissue of Balb/C nude mice. Co-injection of 5 × 103 4T1 mammospheres, a subpopulation of 4T1 cells with enhanced clonogenicity, together with 5 × 104 ASCs into the cleared mammary fat pad of Balb/C mice led to faster tumor growth rates, increased tumor vascularity, and greater incidence of lung metastasis when compared to mice injected with 4T1 mammospheres alone (n = 10 per group). The group then showed that the tumor-promoting effects were in part mediated by ASC secretion of stromal cell-derived factor-1 (SDF-1), a chemokine that has been implicated in cancer metastasis via direct effects on tumor cell migration, which was enhanced under the influence of the tumor microenvironment. Additionally, increased expression of α-smooth muscle actin and von Willebrand factor in ASCs was seen after co-injection with 4T1 mammospheres suggesting differentiation of ASCs into myofibroblasts and vascular endothelial cells, respectively.
55.6 Human ASC Breast Cancer Xenograft Model
Chandler et al. [52] found similar results while using a human breast cancer cell line, MDA-MB-231, and human ASCs in an immunodeficient SCID/NCr xenograft mouse model. Co-injection of MDA-MB-231 cancer cells and ASCs, 1 × 106 of each in 20 μl of media (DMEM/Ham’s F-12, 10 % FBS, 1 % antibiotic), into the cleared mammary fat pad similarly led to larger, more invasive tumors with greater blood vessel density and myofibroblast content at 6 weeks postimplantation (n = 14–16 per group). In vitro experiments exposing ASCs to human breast cancer-conditioned media led to increased proliferation, decreased activity of adipogenic factors (glycerol-3-phosphate dehydrogenase and peroxisome proliferator-activated receptor gamma), decreased lipid droplet accumulation, and enhanced secretion of vascular endothelial growth factor, thereby suggesting alteration of ASC function via soluble tumor-derived factors. Additionally, through a series of experiments employing a variety of gel constructs, a role of ASCs in the physical alteration of the tumor microenvironment towards increasing rigidity was found. Following exposure to tumor-conditioned medium, ASC-derived myofibroblasts exhibited stress fiber development, increased expression of procollagen I/collagen I, and enhanced myosin-mediated contractility, which increased overall matrix stiffness. A feed-forward effect was revealed since greater matrix stiffness in itself led to increased ASC seeding of matrices along with recapitulation of effects that were seen with exposure to tumor-conditioned media. These findings were confirmed in vivo. Tumors recovered in the ASC co-injection group compared to those from the group receiving cancer cells alone had a smaller range of elastic deformation and elevated collagen content.
In direct conflict with these findings were those of Sun et al. who also used the MDA-MB-231 breast cancer cell line, as well as human ASCs, in a similarly immunodeficient NOD/SCID mouse model (n = 10 per group). Unlike the case for Chandler et al. [52], co-injection of MDA-MB-231 cells and ASCs, 1 × 106 of each in 100 μl of PBS, into the mouse mammary fat pads demonstrated inhibition of tumor formation and progression when compared to injection of cancer cells alone at 6 weeks. GFP-labeling of cancer cells in combination with fluorescence microscopy revealed a reduction in the number of lung metastatic sites for mice that had received ASCs [51].
The observed decrease in metastasis was inconsistent with the work of Karnoub et al. [38] in which co-injection of 1.5 × 106 human bone marrow mesenchymal stem cells (BM-MSCs) and 5 × 105 GFP-labeled cancer cells of either the MDA-MB-231, MDA-MB-435, MCF-7/Ras, or HMLER breast cancer cell line in 200 μl of PBS into gamma-irradiated (400 rads, sublethal dose to reduce NK cell activity) athymic female nude mice (n = 5–8 per group) demonstrated increased number of micro- and macroscopic lung metastasis for all breast cancer cell lines (sevenfold, threefold, fourfold, and twofold when normalized, respectively) at 8–12 weeks using a similar quantification technique. They demonstrated in vitro and in vivo an essential role of CCL5 expression by BM-MSCs for the apparent BM-MSC-induced metastasis of MDA-MB-231 cells. Pinilla et al. [53] provided in vitro work suggesting this to hold true for human ASCs as well.
55.7 Primary Human ASC Breast Cancer Xenograft Model
Given the limitations of modeling ASC breast cancer interactions in patients using breast cancer cells of murine origin or established human breast cancer cell lines, Zimmerlin et al. [54] provided a more clinically accurate depiction of these interactions by incorporating primary breast cancer cells isolated from malignant pleural effusions (MPE, n = 10) in conjunction with human ASCs (n = 4). In vitro coculture experiments of MPE cells and ASCs in a 1:1 ratio revealed a greater than fivefold increase in MPE cell proliferation. Previous work by this group demonstrated that CD90 positivity was a marker of cells with stem/progenitor-like qualities in a variety of epithelial tumors including breast cancer and could be used to isolate a subset of cells from MPE that was enriched for tumor-initiating capacity. Additionally, it was found that selecting among the CD90+ population cells having low light scatter properties could further increase tumorigenicity [55]. Based on these earlier findings, following the elimination of hematopoietic, endothelial, and mesothelial cell populations, MPEs were sorted into three groups: high light scatter/CD90+, low light scatter/CD90+, and CD90−. These sorted MPE groups were then injected (100 cells/injection in 50 μl of 50 % Matrigel in DMEM) into the mammary fat pad of immunodeficient NOD/SCID and NOD SCID gamma mice either alone, with 10,000 irradiated unsorted MPE feeder cells, or with 10,000 ASCs. None of the injections involving CD90− cells resulted in tumor formation. Although tumors were detected in the low light scatter/CD90+ injection groups, co-injection of ASCs did not affect the rate of tumor occurrence (6/40 in irradiated feeder group and 4/40 in ASC group). Interestingly, while high light scatter/CD90+ cells alone or with irradiated feeder cells were not tumorigenic, significant tumor formation was noted with the co-injection of ASCs (17/40, 42.5 %) [54].
It would appear from most in vitro and in vivo work involving ASCs and breast cancer that the risk of ASC-mediated cancer progression is a strong possibility. However, as mentioned earlier, MSCs may also be capable of inhibiting tumorigenesis depending on the experimental conditions. Sun et al. have demonstrated this in the case of ASCs and breast cancer [47, 48, 51, 56–58]. Taken in combination with the findings of Zimmerlin et al. [54], it would appear that even in breast cancers that are promoted by ASC-derived factors, the process is not homogenous but rather dependent on the specific subpopulation of the cancer cells that is responsive to this signaling. Defining the specific conditions that determine the type of effect ASCs will have on tumor pathogenesis will be important in resolving the conflicting evidence.
55.8 Limitations of In Vitro and In Vivo Studies
55.8.1 Limitations of Methods
Several methodological considerations that limit the ability to translate basic science findings should be mentioned. Tumor size is often compared in murine studies assessing for tumor-promoting or tumor-inhibiting effects of ASCs. Muehlberg et al. used microCT imaging to measure tumors in all three dimensions and employed the ellipsoid volume equation (4/3πr x r y r z). Sun et al. report volumes using equation volume = (length × width2)/2 in conjunction with caliper-based measurements. Karnoub et al. [38] used spherical volume equation 4/3πr 3 in which the radius was estimated using precision calipers. Approximating the tumor volume with equations imparts an error because the tumor does not represent a perfect sphere or ellipsoid. Furthermore, the user-to-user variability of caliper measurements compounds the error if this method is used to determine the radii for the equation. Approximation errors may be acceptable in the context of large volume differences; however, the range of this error must be determined to know what constitutes a sufficient volume difference. A more precise measure of volume such as gas pycnometry is preferable, but simply providing tumor weights rather than volumes like Chandler et al. [52] seems reasonable. Cases in which tumor mass or volume appears to be enhanced with the addition of ASCs require confirmation that this is not due to the mass or volume of the ASCs themselves.
Limitations intrinsic to the animal model design must be noted as well. While it may be preferable to conduct studies using human breast cancer cells and human ASCs as opposed to those of murine origin, the former generally necessitates immunocompromised mice for xenograft acceptance. Such models inevitably minimize or eliminate the role of the host immune response against cancer initiation and progression. Taken in context of the growing number of reports on the immunosuppressive properties of ASCs and data that suggesting their role in protecting cancer cells form immune recognition, it is clear that immunologic aspects cannot be overlooked in attempts to delineate the risk of introducing ASCs into the breast cancer survivor [59–62]. Moreover, MSCs are but one component of the intricate tumor microenvironment, and despite the use of orthotopic sites for cancer xenograft studies, the absence of other human stromal elements may lead to aberrant behavior that would not occur outside of experimental settings. Therefore, it may be beneficial to humanize murine mammary fat pads such that the stroma may be represented in its entirety [63]. Conversely, including artificial components in the in vivo model such as Matrigel, a reconstituted extracellular matrix derived from mouse sarcoma should be limited since this may give rise to a less physiologic environment [64]. Experiments which employ injection of an admixture of breast cancer cells and ASCs, especially if using relatively high cell numbers, do not seem representative since the clinical risk is that of activating existing dormant or subclinical breast cancer in a patient that has been treated and deemed to be in remission. It is possible that the tumor-promoting effects of ASCs are of consequence only during cancer initiation. ASC-based interventions on preestablished tumors may give more insight into this clinical situation.
55.9 Limitations When Comparing Studies
In addition to remaining cognizant of the deficiencies in current animal models, it is important to be aware of additional variables that may influence outcomes when comparing across studies. With regard to ASCs, donor-related issues and preparation must be considered. Although this remains an area of ongoing research, there have been reports of reduced ASC proliferative capacity or differentiation potential with advancing donor age, gender-specific differences in ASC subpopulation distributions, as well as disparities in plasticity and gene expression profiles based on the anatomic site ASCs were obtained from [65, 66]. Furthermore, changes in functionality and surface marker expression have been noted with variations in harvesting method, culture conditions, and cell passage number [67–70]. As with ASCs, patient-to-patient variation can be expected for clinically isolated breast cancer cells, and whether they were isolated from the primary tumor or MPE will likely be an important consideration as well [71]. Even among established breast cancer cell lines, there will be differences with regard to proliferation rates and propensity for metastasis [54]. ASCs and breast cancer cells together introduce further areas for discrepancy such as cell dose, ASC-to-cancer cell ratio, sequence of injection, or timing of injection [37, 51]. Finally, results may vary due to differences in the time point at which in vivo studies are ended, a concern in particular if a study fails to capture a difference due to premature conclusion.
55.10 Clinical Trials
The oncologic risks are best determined by clinical data, but the overall level of evidence in this area has been fairly low. In a recent publication by Krastev et al. [72], a systematic meta-analysis of all clinical trials published by the end of 2011 was performed to determine the risk of locoregional recurrence in former breast cancer patients following autologous fat grafting to the breast. After an extensive search of PubMed, Embase, and Cochrane Library databases, only 20 trials were recovered in which autologous fat grafting was performed in the context of breast cancer, and only nine of these had reported on oncologic recurrence rates. It was found that most trials suffered from a lack of control groups, small sample sizes, absence of randomization, and their retrospective nature. The highest level of evidence available was determined to be a retrospective cohort by Petit et al. [73], where 321 breast cancer patients that had undergone mastectomy or lumpectomy and subsequently received autologous fat grafting to the breast were matched to twice as many control subjects that did not undergo this procedure. Control subjects were matched such that there would be no significant baseline differences in potential confounders. Characteristics that were matched included age, year of surgery, type of cancer resection surgery, histology, tumor size, estrogen receptor status, and follow-up period. Median follow-up was 56 months from primary surgery and 26 months from lipofilling. In this study, the incidence of local events was comparable between the fat grafting and control groups (hazard ratio lipo vs no lipo = 1.71 [95 % CI 0.67–4.39], gray test P
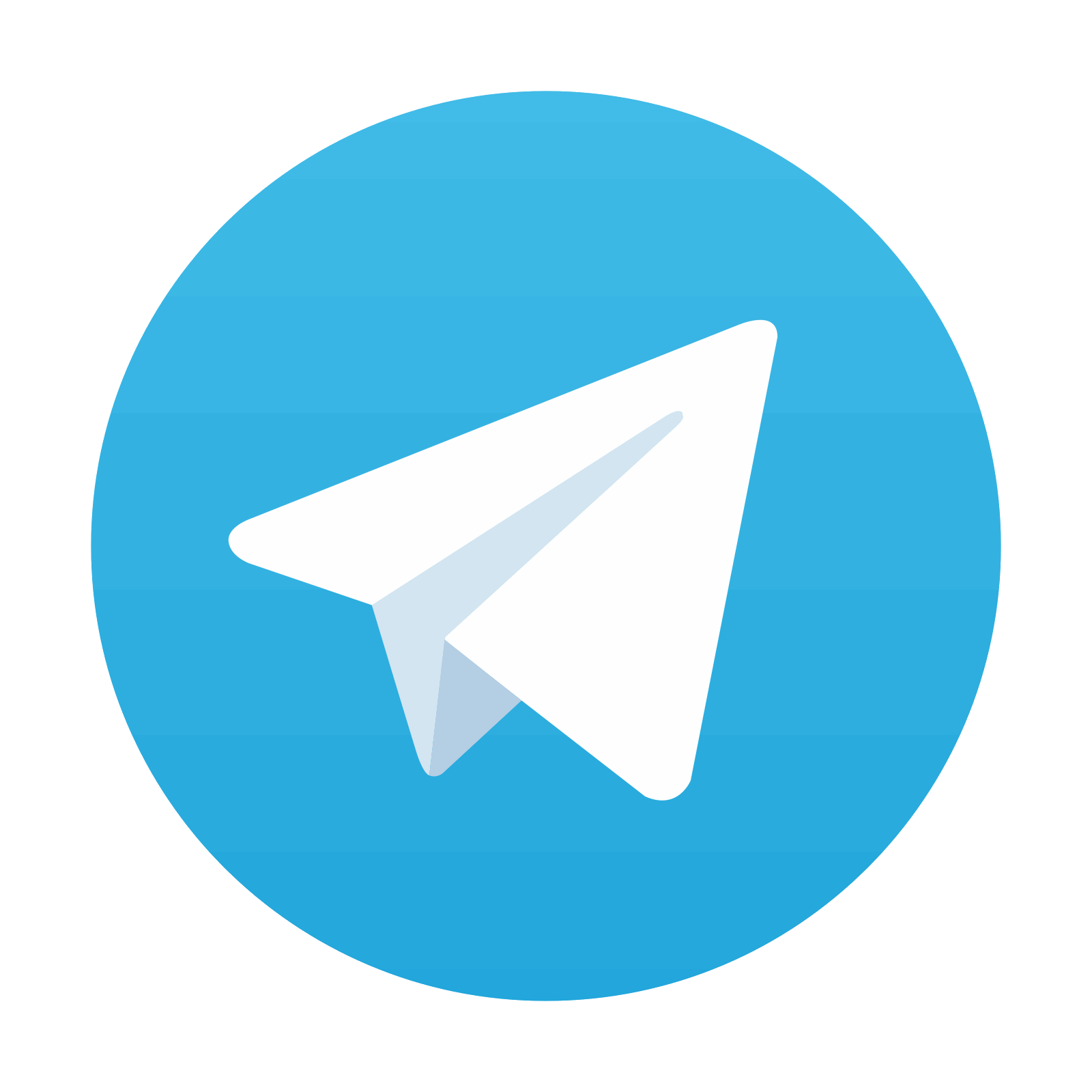
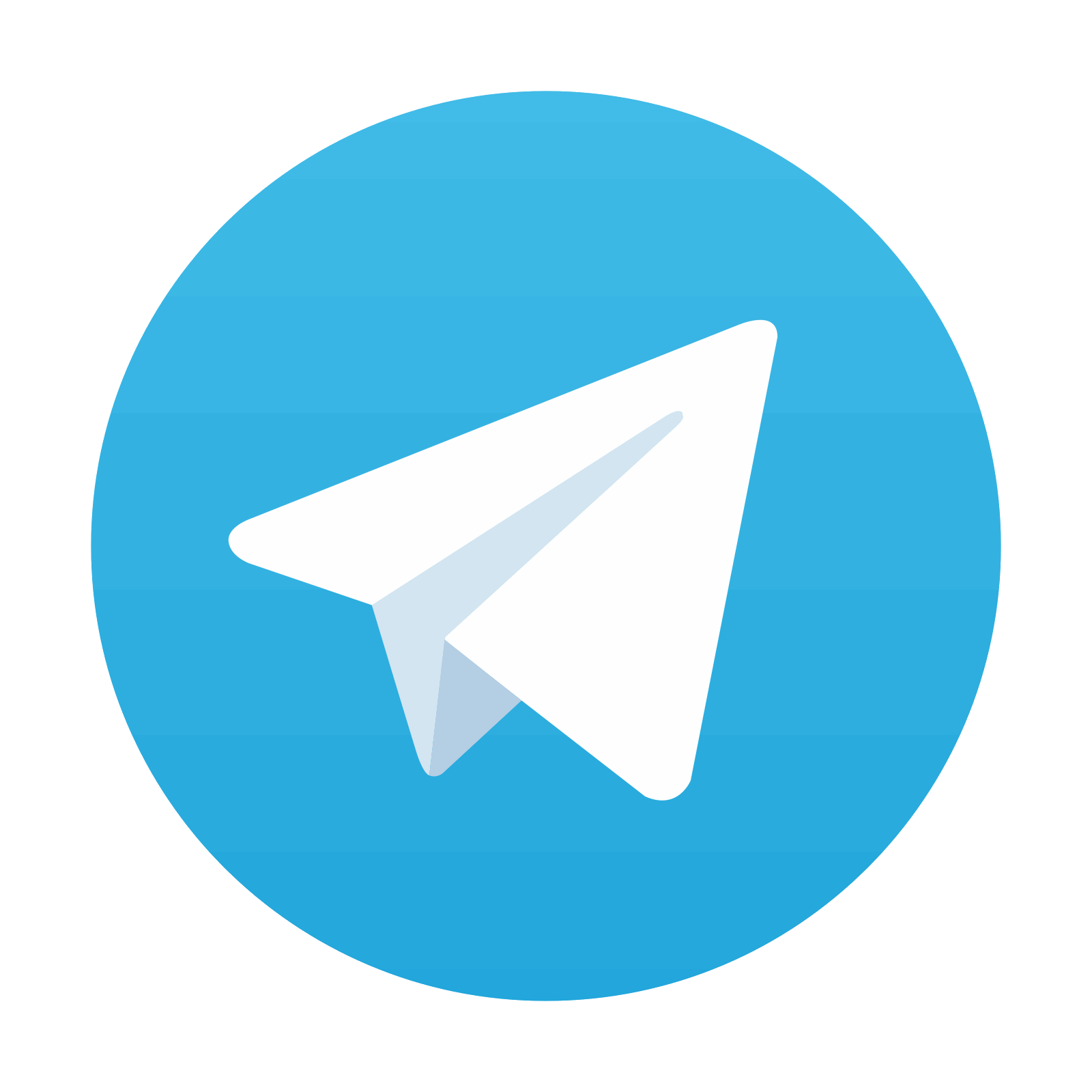
Stay updated, free articles. Join our Telegram channel

Full access? Get Clinical Tree
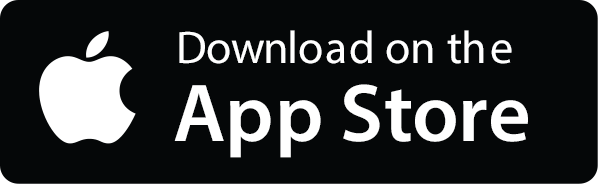
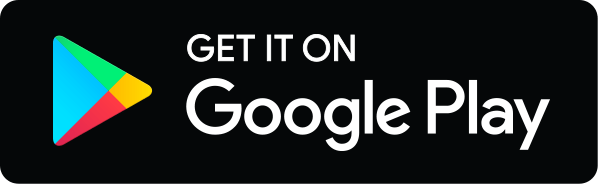