President of KAL’IDEES S.A.S.
ABSTRACT
Theories of why we age are numerous and no general consensus has resulted from the many years of research. A review article of the various theories of aging (Huber and Hess 2010) lists ROS (free radicals), mitochondrial dysfunction, telomere shortening, and glycation as currently held opinions on why we age.
This chapter briefly describes the two latter mechanisms, focusing on aspects of skin physiology, and then reports some of the latest developments of cosmetic ingredients designed to alleviate and, wherever possible, reverse the aging symptoms that are connected to these causes. As one reads through the literature from various databases (PubMed, Google Scholar, Kosmet), it becomes clear that not one of the various “concepts” often singled out by cosmetic research communication stands truly alone as a target for anti-age activity. Stem cells, sirtuins and hormesis, proteasome and mitochondrial activity, ROS, telomere length, glycation, proteolysis, inflammation, and melanogenesis, all hang together in complex interplays, making any classification of research articles (or ingredient-data brochures) to one or the other topic rather frustrating. It is even worse for finished products, which, except for very specific cases, mix these ideas and properties in often quite arbitrary fashion, depending on the current market and competitive situation. We cannot even really distinguish the two main categories of anti-age cosmetics of “prevention” and “treatment,” as many ingredients possess activities that promise and deliver both aspects, as a review of the relevant literature in peer-reviewed and in technical trade journals shows. A typical example:
Blood circulation becomes poor due to aging or a disturbed daily rhythm, which leads to skin problems such as dark eye circles and lip disorders. In this study, we focused on developing Piper Longum Fruit as a cosmetic ingredient for improving poor blood circulation. . . . Piper Longum Fruit extract promoted blood circulation by the topical application on human skin. In addition, Piper Longum Fruit extract showed various activities such as promotion of keratinocyte proliferation, increase of filaggrin synthesis, inhibition of melanin synthesis and anti-glycation. These results suggested that Piper Longum Fruit extract might be a useful ingredient for cosmetics. (Kiso 2011)
b. Prevention and/or Reversal of Glycation/Glycoxidation
b. Cosmetic approach to proteasome activity
c. The study of the LC3-II protein
d. Cosmetic ideas on telomere maintenance
As so many other topics, glycation (and anti-glycation) are recurring themes in cosmetic research. As our understanding progresses, new ideas of how to fight glycation and/or how to reverse the process once initiated are investigated and tested. Scientists at Estée Lauder some time ago called anti-glycation the tool to fight aging (Maes et al. 2007). They then soon also became interested in the proteasome, the sirtuins, then the stem cell concepts, etc. As we shall see, though, all these ideas are somewhere connected.
Glycation is a non-enzymatic process whereby various sugar molecules react nonspecifically with proteins (and other macromolecules). This phenomenon increases with age, in all types of tissue, particularly in people with diabetes, and leads to degradation of enzymatic vital functions. These include brittleness in collagen and elastin fibers, leading to wrinkles, decreased elasticity, and reduced efficacy of cellular mechanisms. Ingredients with antiglycation activity attempt to prevent this sugar-attachment to proteins; some progress has even been made with partial reversal of the glycation reaction, not only in vitro but also in some clinical studies. For example, skin aging has long been associated particularly with exposure to sunlight, but glycotoxins from the diet or even from cigarette smoke (Cerami et al. 1997) are at least as important a factor in skin aging. The term glycotoxins comprises a heterogeneous group of molecules, many derived from sugars, which bind to the different components of the cell and change their qualities or properties.
Our body needs sugar, glucose, to produce its energy. Glucose, however, is not entirely used by the body and some binds directly to protein amine groups, nucleic acids, or lipids and creates Advanced Gyration Endproducts (AGEs). These compounds were described for the first time by Maillard in 1912.A brief review of the field is given by Draelos and Pugliese (2011).
AGE formation involves several successive stages (see Figure 1). In the early stages, the aldehyde group of a reducing sugar such as glucose forms an unstable bond with the protein amine, known as a Schiff’s base, which rapidly rearranges to produce an Amadori compound. These two formations are reversible. The next, later, stage leads to the formation of almost irreversible advanced glycation endproducts, which accumulate over time in tissues.
At the Amadori compound stage, the sugar can deprotonate and create several highly reactive compounds that themselves become glycotoxins: glyoxal (GO) or its methylglyoxal derivative (MGO), 3-deoxyglucosone (3-DG), and fructosamines (Thornalley et al. 1999).
Figure 1: Simplified mechanism of formation of AGEs
Glyoxals and 3-DG can in turn bind to free protein amines and produce different AGEs such as carboxymethyl-lysine (CML), carboxymethyl-arginine (CMA), carboxymethyl cysteine (CMC) , pentosidine, and pyrraline. These reactive chemical groups can also be formed by glucose auto-oxidation. Other sugars such as ribose or xylose, although present in smaller amounts, are far more reactive than glucose and fructose and also produce AGEs, which have only been shown to be toxic in vitro in the absence of reliable in vivo demonstration methods. Experimentally, these products are formed very quickly (Wei et al. 2009).This leads to the formation of many altered proteins (particularly those with a long half-life), whose functional, enzymatic, and structural properties are also changed with serious consequences on the cell or body. SOD and catalase are among the enzymes most often cited.
Collagen, for instance, has a half-life of 15 years and therefore contains three times more carboxymethyl-lysine (CML), carboxyethyl-lysine (CEL), and pentosidine at age 80 than at age 20 (Page on et al. 2010).
Mizutari (1997) studied the influence of UV exposure and showed that UV-induced oxidation accelerates CML formation in actinic elastosis of photo-aged skin, demonstrating once more that the various influences on aging complement and reinforce each other (oxidation, UV irradiation, glycation . . .).
Glycated collagen also promotes apoptosis, both after injection and in contact with cultured fibroblasts. This may be due at least partly to overproduction of H2O2 by methylglyoxal (MGO) and glyoxal (Sejersen & Rattan 2009), which would then explain the acceleration in the senescent phenotype of these cells. The AGEs also activate inflammatory mechanisms that themselves are pro-oxidizing, further fueling the aging process.
Although the process of glycation occurs throughout the body, and affects all proteins, a study by Kueper et al. (2007) shows that the cytoskeletal protein vimentin is a specific and primary target of glycation in skin, leading to reduced contractile function in fibroblasts. Fibroblasts in the skin’s dermis create collagen and the elastic fibres necessary for young looking skin. An interesting analysis of glycation specifically occurring in the stratum corneum taking into account environmental factors is presented by Gomi et al. (2010).
Many more interesting scientific studies on glycation and the skin can be found in the literature. Their detailed presentation here would go beyond the scope of this chapter and book.
Knowing how detrimental the formation of AGEs is for the body, it had been an important challenge to measure and quantify the amount of glycation occurring in an organism. For many years, diabetologists used skin biopsies and histological AGE labeling to estimate the severity of a patient’s state. This very invasive method had then been replaced by measurement of blood-glycated haemoglobin, which is less invasive and can, for example, be repeated if the patient is in a hospital. More recently, an instrument called AGE-Reader™, which measures AGEs present in the skin completely painlessly by autofluorescence, has become available. The AGE-Reader™ (DiagnOptics Technologies) is the first medical device allowing noninvasive measurement of AGE accumulation. The volunteer’s skin is exposed to light at a wavelength of 370 ± 50 nm, which excites functional fluorescent groups, particularly those in the AGEs. The reflected light is transmitted selectively by a 50 μm diameter fiber and measured by spectrophotometry (Lutgers et al. 2006). In practice, the volunteer places his/her forearm on the instrument and measurements are taken through a 1 cm2 window.
From such studies, a remarkable fact has come to light: there is a strong linear correlation between AGE-Reader™ readings and the age of the person measured; to a point where a significant increase in (arbitrary) units on the instrument readings of 0.023 per year of subject age can be read on a graph; even somewhat different regression lines are obtained for smokers or diabetics (Koetsier et al. 2010).
Maes and his team went further in calculating apparent age by using both the autofluorescence of skin combined with facial skin parameters to predict the efficacy of cosmetic products and to assess the predisposition to accelerated aging (Dicanio et al. 2009, Corstjens et al. 2008).
b. Prevention and/or Reversal of Glycation/Glycoxidation
Is there a way to prevent glycation, in general, and on the skin in particular? And even better: Is there a way to reverse it, to eliminate glycated structures or to “deglycate” them? Surprisingly—in view of earlier documents that call glycation an “irreversible” deterioration of macromolecules—there have been at least partially successful attempts to fight this particular aspect of aging.
Of course, reducing sugar intake in our diet and consuming food rich in antioxidants are first measures of prevention. Not using monosaccharides (honey . . .) in cosmetic formulas would be another logical consequence.
More to the point of the present chapter: AGEs are not formed immediately, glycation proceeds in two phases, the first stage is rapid, the subsequent more slowly. The early forms of AGEs are generated either directly or indirectly, via glyoxal or methylglyoxal. Cells have an active mechanism based on an enzyme system called Glyoxalase -1 and -2, which detoxifies the glycotoxins via glutathion GSH (Rabbani & Thornalley 2009). Experimental overexpression of glyoxalase-1 in diabetic animals increases their lifespan, whereas any reduction in its activity reduces survival.
As is so often the case with the organism’s defense mechanisms: the very systems (enzymes) that exist to protect cells and organs are equally affected by the very agents they are designed to inactivate. The efficacy of antioxidant enzymes (SOD, catalase, glutathion peroxidase) is decreased by oxidative attack (and by glycation!), and the glyoxalase enzymes are equally subject to these destructive processes: glycation products tend to reduce glyoxalase activity.
Diabetes is a major and widespread disease, justifying extensive pharmacological research in preventing and treating it. Cosmetic research, interested in the aspects of skin aging, has indirectly benefited from the learnings and has generated a great number of studies and research projects attempting to prevent and/or reverse skin-based glycation/glycoxidation. In the following paragraphs, we shall briefly present some of the ideas and results obtained in recent years.
Given the fact that many papers on the mechanisms of glycation mention oxydation as an essential element in the chemical reactions of glycation/glycoxidation (e.g., Baynes 1991), it is not surprising that many if not most anti-glycation proposals are based more or less consciously on antioxidant activity. The other major idea is to supply “sacrifice” substances that compete with the reactive sugar molecules, thus preventing their attachment to proteins. Aspirine for example attaches acetyl groups to the amines of lysine, thus blocking the reaction sites (Watala et al. 2005).
A great number of plant extracts with more or less defined content of specific active substances, but consistently tested for antioxidant activity, are thus proposed as “anti-glycation” anti-age “actives.” The AGE-Reader™ technology, not yet being widely used, appears to be best suited to substantiate the anti-glycation efficacy based on in vitro studies.
Schmid et al. (2002) relate the effects of a combination of grape seed procyanidines and a-g-d-tocopherol on AGE and Amadori production with bovine serum albumin incubated with glucose. Aminoguanidine, a well-known benchmark for anti-glycation activity, was used as control. Inhibition of AGE formation achieved 90% with the antioxidant mixture.
Pauly et al. (2011) and Jeanmaire (2011) showed Manilkara multinervis leaf extract1 to be active in both in vitro and ex vivo protocols; the same team (Jeanmaire et al. 2005) had already investigated the bark extract of Pterocarpus marsupium Roxb. on its activity in isolated hair follicles, assuming that glycation contributes to hair loss and baldness. A clinical study appeared to confirm the hypothesis.
Yashihiki (2012) focused on UME extract (Japanese Prune mume, often presented as a health drink) for improving skin dullness. This result mirrors the radiance improvement demonstrated in vivo by the cosmetic topical use of fermented black tea (Kombucha, the traditional Mongolian beverage),2 tested in similar ways.
Further plant-derived in vitro tested anti-glycation ingredients are puerarin and chlorogenic acid (Gasser et al. 2011), sunflower (Helianthus annuus) seed cake extract3 (Lenaers 2007), an antioxidant series of garlic (Allium sepa) skin, Illicum religiosum (bark and wood), rosemary leaf extract, Origanum officinals, etc. (Kim 2003), Panshanbheda and pomegranate flower and rose apple leaf (Kanazawa 2012), Paenia suffruticosa and Sanguisorba officinalis (Okano 2001).
Additional interesting papers on glycation were presented at the 2010 IFSCC congress in Buenos Aires. Fitoussi et al. (2010a) were interested by the aforementioned observation on vimentin being a prime target of glycation (Kueper 2007) and carried out a study to show the ability of a Chrysophyllum extract to prevent the glycation phenomenon and the glycation consequences in human skin fibroblasts. On cultured normal human fibroblasts, the authors show that by inhibiting type I collagen and vimentin glycation, Chrysophyllum extract retards the cellular aging process and enables dermal fibroblasts in vitro to retain their motility and their important contractile power.
D’Arcangelis et al. (2010) sought to study the consequences of lowering pH from 7.0 to 3.5 on protein glycation in vitro. Reaction of reducing sugars with bovine serum albumin (BSA) or a Gly-Lys dipeptide to produce fluorescent glycation products was decreased proportionally with decreasing pH. The decrease observed as the pH is lowered to <7.0 is amplified by the addition of glycation inhibitors, aminoguanidine, or Bay cedar extract, which further lower the accumulation of fluorescent products in an additive manner.
This group of researchers had previously shown that this Bay cedar extract acted by stimulating the skin’s own cellular defense/repair system composed of fructoseamine-3-kinase (FN3K), an apparent de-glycation system. An observed increase in FN3K after Bay cedar extract incubation led to partial restoration of glycation-related damages (Federova et al. 2008).
A rather surprising approach is described by Kim et al. (2012),using gold nanoparticles, was shown to prevent collagen glycation, albeit again just in vitro.
Other nonplant-based approaches to inhibiting glycation should be mentioned:
Rather than just preventing oxidative steps, scientists from Exsymol developed Decarboxy Carnosine (Carnicine), which acts as a transglycation agent able to detach sugars from proteins and scavenging them on the NH2 group of beta-alanine (Lafitte 2008, Nicolay 2010). Although interesting, this original approach still requires field-testing in a clinical trial.
Monsan (1998, 2001) was probably the first to try oligopeptides composed of lysine and arginine only, obtained by enzymatic synthesis4 as sacrifice molecules to prevent glycation of the skin’s proteins. Much more recently, Guglielmini et al. (2012) described an azeoloyl-tetrapeptide5 that possesses antioxidant and anti-glycation activity. The peptide reduces AGE formation in fibroblasts by ≈50% and ROS in keratinocytes by similar amounts. A vehicle-controlled clinical study documents improved skin parameters (wrinkles, elasticity) after six weeks of treatment.
Merino (2010) took a step in a different direction and measured glycation on DNA, rather than proteins, also using a peptide (Tripeptide 9 Citrulline).6 The preparation chelates harmful copper ions and prevents the reaction of methylglyoxal with DNA in vitro.
Draelos et al. (2009) carried out one of the first cosmetically inspired clinical studies on diabetic skin, using several parameters to judge the potential beneficial effect of a topical product containing C-xyloside and blueberry extract. C-xyloside was chosen as a GAG synthesis stimulator and blueberry extract (an antioxidant) for its anti-glycation potential. Dermatological scoring of wrinkles, fine lines, smoothness, skin tone, sagging, etc. was coupled with the measurement of AGE associated skin autofluorescence. Most of the scores improved significantly over 12 weeks, including skin moisture (Corneometry, p<0.001), which in itself might be the cause of the overall improvement. Unfortunately no control formula without the “actives” included was used in the study. The AGE autofluorescence values did not change significantly over the time studied, a fact that might be explained by the self-imposed tough challenge of using diabetic skin. It was previously mentioned that the skin appears to have an intrinsic defense and repair system for glycation-related phenomena. Deglycating enzymes (called Amadoriases) have been evidenced in vertebrates (Monnier and Sell 2006), but the discovery has not yet led to development of true AGE-breakers. Alagebrium®7 once considered to be a promising prescription drug with evidence for being able to revert AGE formation, but has not succeeded in establishing a market. Tada et al. (2008) presented intriguing possibilities for achieving the breakage of cross-linked proteins using YAC extract (Artemisia princeps). On the other hand, Matsuura et al. (2006) studied plantago side and penathydroxy flavanones, which cleave a-diketones and AGE cross-links in vitro.
The most recent offering to reduce the AGE related symptoms and skin damages, is again plant based: An extract of Albizia julibrissin.8 Numerous endpoints were measured extensively both in vitro, ex vivo (3D model), and in vehicle-controlled clinical trails, including the skin’s autofluorescence in vivo (Mondon et al. 2012).It is important to understand that in almost all studies described in the previous paragraphs, the observed anti-glycation effect was followed in protocols of “prevention.”As the following data show, the Albizia extract provides both protective (preventive) and curative (reversal) activity.
In all following cellular and skin model experiments, a sufficient preincubation period of the cells and/or the ex vivo skin with methylglyoxal preceded the contact with a control medium or the Albizia-containing medium. Thus, glycation and the catalytic production of AGEs had already been set in motion before any preventive and/or curative action of the plant extract was initiated. This is a more challenging and more reality-relevant protocol than simultaneous incubation of cells or skin with MGO (the “aggressor”) and the active ingredient (the “protector”).
An extract of Albizia julibrissin (called the “night sleeper” in Persia) was prepared by lixiviation. In screening assays it turned out that this particular extract inhibited the glycation of BSA (bovine serum albumin) with glucose by close to 50%.
From this initial observation, further studies were undertaken to investigate the mechanisms and efficacy of anti-glycation activity. Carboxymethyl-lysine (CML) and Carboxyethyl-lysine (CEL) are typical AGE products of collagen aging and glycation via a reaction with methylglyoxal (a first oxidized derivative of sugar). Treating fibroblasts in culture with MGO leads to a strong reduction in cell viability (–44%) and a huge increase in CML (+124%, p<0.01). When the cells are subsequently in contact with the Albizia extract for five days and CML levels are again checked in comparison to untreated cells, they decrease by about 43% in the Albizia-contacted cells.
product | AGEs (AFU*) | % change; significance |
MGO, then vehicle cream | 9.56± 1.30 | baseline |
MGO, then 4% cream | 7.33± 1.40 | –23.4%; p<0.01 |
* AFU: arbitrary fluorescence units
Human abdominal skin explants from a 34-year-old woman were placed in contact with MGO dissolved in the survival medium. After the glycation phase, the skin (n=5/case) received an application of a cream containing 4% of the Albizia extract preparation or the vehicle cream (both of which were used in the following clinical tests). Topical applications were administered once per day for 4.5 days. The skin sections were cut with a microtome before being labeled with a fluorescent anti-AGE antibody. Ten photos/cases were taken and analyzed on image analysis software. The results (Table 1) show that AGE labeling was located particularly in the dermis, an area rich in stable matrix proteins, and significantly reduced in the treated skins.
This is confirmed by the quantification of glyoxalase-1 synthesis in these MGO-treated fibroblasts where a Western blot analysis shows a 56% drop in detoxifying glyoxalase-1 in the control media, whereas the Albizia-treated cells maintain their initial glyoxalase-1 level.
We have previously mentioned Vimentin as a major target of glycation. MGO-incubated and then vehicle-treated skin samples showed a drastic reduction in Vimentin, whereas Albizia extract prevented/repaired this effect and maintained Vimentin staining 75% higher (p<0.01), which in a parallel experiment preserved fibroblast contractile capacities (data not shown).
44 panelists with stressed skin (as determined by AGEs present in adhesive strippings) were enrolled in randomized vehicle-controlled clinical trials on three subgroups. Endpoints measured were autofluorescence (AGE-Reader™), AGE content in tape strippings of the forearm, skin fatigue analysis using the Reviscosimeter™ method, and a daily and weekly self-assessment of facial fatigue every morning. Creams with either 2% (day cream) or 4% (night cream) of the Albizia preparation were tested against the identical vehicle without the plant extract. Application sites were the two forearms; frequency of application was twice a day over a two-month period.
Fig. 3: Decrease in AF values after two months’ treatment
The graph shown in Figure 3 indicates the difference in AGE-Reader™ Autofluorescence (AF) values between the Albizia-treated skins and the vehicle-treated ones, after two months. AF values are converted into AGEs, glycated collagen, or Pentosidine according to Lutgers et al. (2006), Greven (2010),and Samborski (2011) and can further be interpreted in age differences based on a large datafile linking AF values to skin age (Koetsier 2010).
Similar significant results were obtained with the Reviscosimeter method. Self-assessment (scoring of various facial features such as baggy eyes, dark circles, and drawn faces every morning at wakening, in front of a mirror), established the visible benefits of a novel cosmetic ingredient to combat aging and skin fatigue both via preventive and curative treatment of glycation, and confirmed that Albizia is not without reason called “night sleeper,” as skin treatment with this extract appears to give similar results as a good “beauty sleep.”
The example presented here shows, on the basis of the extensive in vitro, ex vivo, and clinical data, that an approach to simultaneous immediate skin beautifying and long-term physiological skin care is possible and justifiable. The measurable results on the three panels of volunteers are in good correlation with the proposed in vitro mechanisms of activity of the ingredients formulated in these preparations.
The study of glycation and the fight against this age-related phenomenon in skin care has come a long way. There is still much room for further discoveries and development of increasingly efficacious ingredients, especially now that easily measured values can be obtained in clinical studies, which after all are the final judge of cosmetic efficacy.
The skin is exposed to many external stresses: UV, oxidizing agents, pollution, and biological agents, inducing more or less chronic micro-inflammatory effects and the formation of noxious products in or around the epidermal cells, including pre-inflammatory mediators and carbonylated proteins, lipoperoxides, and lipofuscin, which disturb cellular physiology and homeostasis, adversely affecting mitochondrial respiration, membrane integrity, enzyme activity, and purification mechanisms such as the proteasome. Accumulation of these noxious products, which can be seen in a simple keratinocyte culture, (see Figure 4) can be assessed both from their harmful effects and also from their ability to absorb light and reduce epidermal transparency.
Figure 4: Keratinocyte cultures without (left) or with (right) accumulations of noxious products
Fig 5: A view down the central core of the proteasome complex
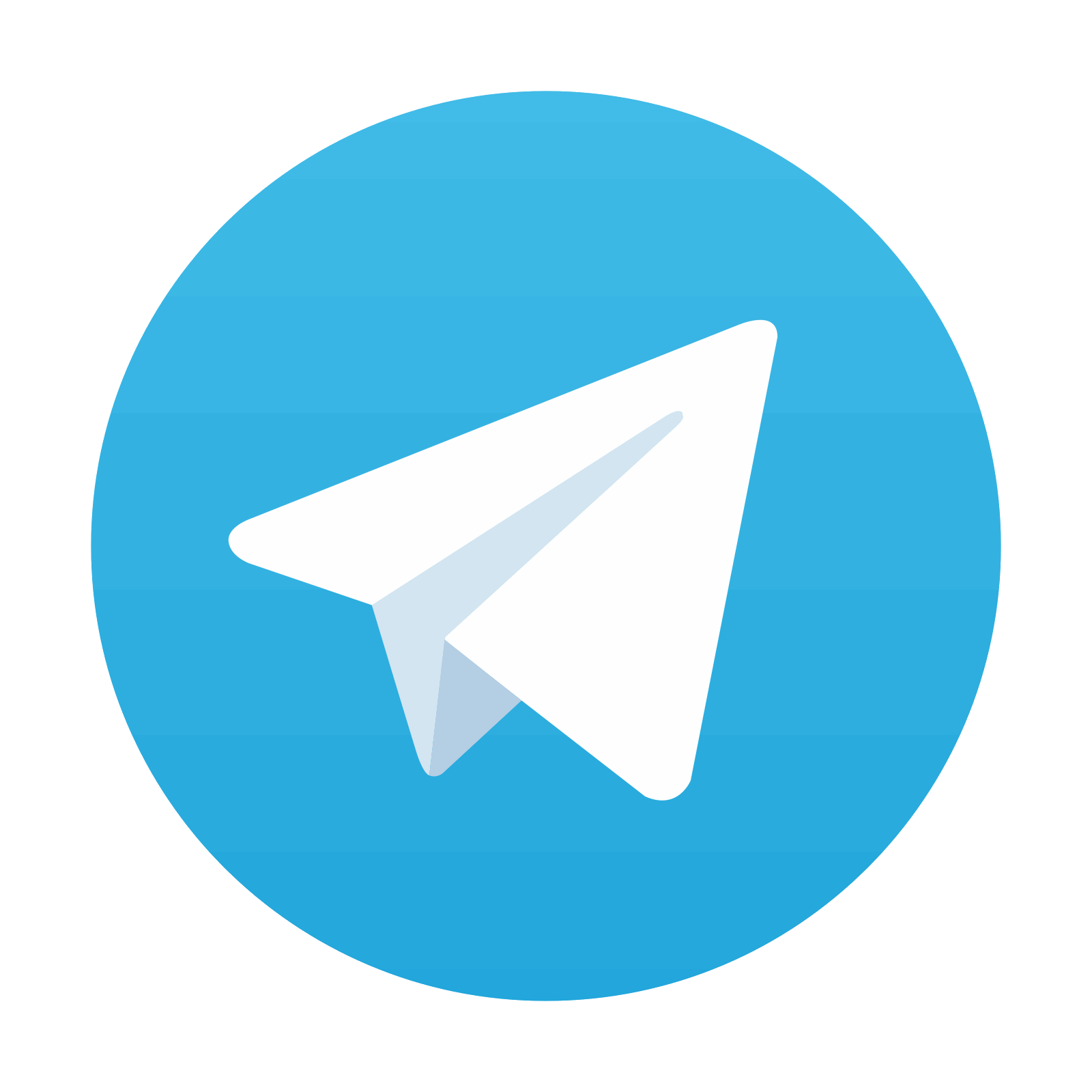
Stay updated, free articles. Join our Telegram channel

Full access? Get Clinical Tree
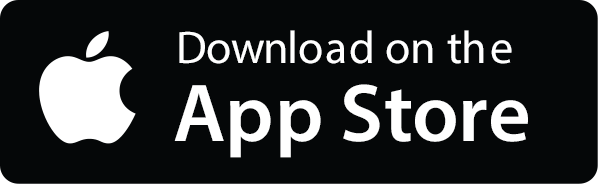
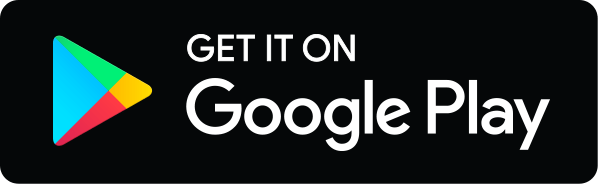