Autoimmune disease
Percent female patients (highest estimate)
X chromosome
MHC/HLA association (selected)
Non-MHC gene associations
Autoimmune hepatitis
Type I 9:1
NA
A1-88
IgA [5]
Type II: 3:1 [5]
DRB* 0301
DRB* 0401
DRB* 0404 [5]
Crohn’s diseasea
58 % [6]
NA
HLA-DRB1*07 [7]
IL23R, NOD2 CARD9 IL18RAP, CUL2, C1orf106, PTPN22, and MUC19 [8]
Hyperthyroidism/Graves’ disease
95 % [9]
(Skewed) inactivation [10]
DR3
CTLA4 [11]
DRB108 [12]
Multiple sclerosis
60 % [13]
NA
DR2
CTLA4 [14]
DR3
DRA
DRB1 [9]
Primary biliary cirrhosis
88 % [15]
NA [16]
DRB1*0801 [17]
IL12A and IL12RB2, CTLA4 [17]
Psoriasis
38 % [18]
NA
HLA-C [19]
IL12B
IL23A [19]
Rheumatoid arthritis
72 % [15]
Yes [20]
DRB1-0101, DRB1-0102, DRB1-0404, others [12]
PTPN22, STAT4 [21],
Scleroderma
84 % [15]
Yes [22]
HLA-DRB1*01 and HLA-DRB1*11 [23]
BANK1, C8orf13-BLK, IL-23R, IRF5, STAT4, TBX21, and TNFSF4 [24]
Sjögren’s syndrome
95 % [5]
Gene dosage [25]
–[26]
–
Systemic lupus erythematosus
90 % [13]
[27]
DR3, DR8, DR15 [12]
STAT4, IRF5, ITGAM [28]
Type I diabetes
50 % [9]
NA
DR3
Lymphoid tyrosine phosphatase (PTPN22) and the cytotoxic T lymphocyte-associated antigen-4 (CTLA-4) gene [29]
DR4 [12]
Ulcerative colitis
50 % [5]
NA
B27b [30]
ECM1, CDH1, HNF4a, and laminin B1 [30]
Table 24.1b
Part 2: selected gender influences implied or positively associated with specific autoimmune diseases
Autoimmune disease | MZ/DZ | Geo-epidemiology prevalence/100,000 | Associated environmental exposures |
---|---|---|---|
Autoimmune hepatitis | NA | NA | |
Crohn’s diseasea | NA | Highest: North America (201) [9] | Tobacco smoke [33] |
Lowest: Israel 2b [34] | |||
Hyperthyroidism/Graves’ disease | 31/4.7 % [35] | Highest: N. Europe, China (1200) [9] | Tobacco smoke [33] |
Lowest: Africa (<10) [9] | Radiation [36] | ||
Multiple sclerosis | 31 %/5 % [35] | Highest: N. Europe (200) | Tobacco smoke [33] |
Lowest: South and Central America and the Caribbean (<20) [9] | |||
Primary biliary cirrhosis | 62.5 %/0 [39] | NA | Tobacco smoke [33] |
Psoriasis | 67 %/15 % [35] | Highest: France (3058) | Periodontal disease [40] |
Lowest: indigenous S. American: 0 [9] | |||
Rheumatoid arthritis | 15/3.5 % [35] | – | Tobacco smoke [33] |
Scleroderma | – | – | Tobacco smoke [33] |
Systemic lupus erythematosus | 33 %/2 % [35] | – | |
Type I diabetes | 70/13 % [35] | Highest: N. Europe (700) | – |
Lowest: Central America (<20) [9] | |||
Ulcerative colitis | 18.7/3 % [35] | Highest: North America (300) | – |
Lowest: Central and South America (<3) [9] |
The mechanisms which underlie this diverse group of diseases are still poorly understood. Many autoimmune diseases display both genetic predisposition and familial associations, firmly establishing a genetic foundation for their eventual development [2,41].
Most also have obvious environmental triggers as well; a wide variety of environmental insults including infectious agents, pharmaceuticals, dietary factors, smoking, chemicals, and pollutants are shown to increase the risk [2,41].
In addition, since autoimmune disease affects predominantly women (80 % of autoimmune disease sufferers are female) [13], with the activity of disease fluctuating in parallel with estrogen levels, sex steroids are widely proposed as a key component of autoimmune pathology [42].
Finally, as both the levels of circulating of autoantibodies (in association with a general dysregulation of immune function) and the risk of autoimmune disease are observed to increase as humans age [43], it is probable that immunosenescence also plays a role.
Although the precise pathways through which a genetic predisposition to autoimmune disease becomes a full-blown autoimmune disease (through the influence of endogenous hormones, environmental insults, and the natural decay of the aging immune system) are still unknown [3], progress suggests that lifelong epigenetic changes to deoxyribonucleic acid (DNA) are a primary means to gene regulation. Because of this, autoimmune diseases, potentially devastating conditions with complex etiology and paradoxical nature, are better understood.
24.2 The Genetic Underpinnings of Autoimmune Disease
The existence of a genetic platform that confers susceptibility to or protection against autoimmune disease has been long suspected. Only a handful of these diseases display strict Mendelian inheritance [44]. Certain autoimmune diseases, nonetheless, consistently occur at statistically improbable levels in certain families [45].
Apart from Mendelian inheritance, familial aggregation is modest [44]. For example, despite the fact that a family history of systemic lupus erythematosus (SLE) increases the risk 25-fold, only 2 % of an AID patient’s close relatives actually develop the disease [46]. Thus, the manner in which the genome confers protection or susceptibility through modulation of immune tolerance is not solidly identified, and patients in which a genetic profile has been firmly linked to the development of disease are a substantial minority [47]. This situation arises because many of the identified genetic associations have odds ratios (typically 1.1–1.5) that are too small to meaningfully increase individual risk [44].
The genetic contribution to autoimmunity is “extraordinarily complex” [44]. Refinements in methodologies in molecular biology, however, are beginning to unlock the genetic basis of autoimmunity. Culpable genes in the pathogenesis of autoimmune diseases were first pursued through candidate gene association studies, then by linkage analysis in affected families, and then through genome-wide association scans [44] (Table 24.2). Genome-wide association studies, built on the completion of the Human Genome and the haplotype map (HapMap) projects, have particularly accelerated solid associations between disease and specific genes, courtesy of their ability to scan the entire genome for polymorphisms implicated in disease [44].
Table 24.2
Ability to establish genetic foundation spurred by ongoing innovations in molecular methodology
Technique | Strength | Weakness | Approximate year of innovation | References |
---|---|---|---|---|
Linkage analysis | Solid identification of AID with Mendelian inheritance | AIDs with strict Mendelian inheritance make up a small fraction of known AID | 1953 | [44] |
Candidate gene studies | Useful for identifying gene associations in disease with more complex, non-Mendelian inheritance | Requires large sample sizes as well as an existing understanding of disease mechanisms with implied plausibility of specific gene. Also requires case/control matching | 1986 | [44] |
Genome-wide association scans | Does not require prior plausibility for specific genes | Requires huge databases (Human Genome Project and HapMap project made those available) | 2005 | [44] |
More than 200 genetic loci have now been documented as contributing to various autoimmune diseases [4]. Some genetic variants, that is, human leukocyte antigens (HLAs), have been reliably associated with several different autoimmune diseases, including type I diabetes mellitus (T1DM), rheumatoid arthritis (RA), and SLE [3]; the signal transducer and activator of transcription 4 (STAT4) (a transcription factor gene) haplotype is associated with an increased risk of both RA and SLE [21]. Through these genetic techniques, multiple potential pathways for the origin of autoimmunity have emerged. These are primarily related to innate immunity, cytokine signaling, or lymphocyte activation [3], and include molecules like intracellular tyrosine phosphatases, tumor necrosis factor(s), intracellular pattern recognition receptors, nuclear factor kappa-light-chain-enhancer (NF-Кβ) and other transcription factors, cytokines (and their receptors), cell-surface receptors, signaling molecules, enzymes, autoantigens, and major histocompatibility complex (MHC) profiles [44].
Intriguingly, the loci identified are often shown to be common to a number of autoimmune diseases [4], and the presence of an autoimmune disease in one individual increases the risk in close family members to a variety of others. For example, analysis of variants of the protein tyrosine phosphatase (PTPN) gene in Swedish patients with autoimmune diseases such as RA, T1DM, or Crohn’s disease (N = 172,242) found that the relative risk of several autoimmune diseases increased in offspring when the parent suffered from an existing autoimmune disease [45] (Table 24.3). Pairwise analyses demonstrated a shared familial risk for RA, SLE, T1DM, ankylosing spondylitis (AS), Crohn’s disease, celiac disease, and ulcerative colitis (UC) (see Table 24.3).
Table 24.3
Familial clustering associated with high-risk gene variants in autoimmune disease (selected associations)
Disease diagnosed in parent | Relative risk (as standardized incidence ratio [SIR]) of disease in offspring | ||||||
---|---|---|---|---|---|---|---|
AS | CD | CE | RA | SLE | T1DM | UC | |
Ankylosing spondylitis | – | NIR | NIR | 1.41 | NIR | NIR | NIR |
Celiac disease | NIR | 1.61 | – | 1.31 | 2.72 | 1.91 | 1.27 |
Crohn’s disease | 1.86 | – | NIR | 1.14 | NIR | NIR | NIR |
Systemic lupus erythematosus | NIR | NIR | NIR | 1.77 | – | NIR | NIR |
Type I diabetes mellitus | 1.44 | NIR | NIR | 1.72 | 1.87 | – | NIR |
Ulcerative colitis | 1.72 | 2.55 | NIR | 1.15 | NIR | 1.29 | – |
A genome-wide association study looked at single-nucleotide polymorphisms (SNPs) outside the MHC and identified a total of 107 SNPs that were associated with an increased risk for at least one of seven individual autoimmune diseases: celiac disease, Crohn’s disease, multiple sclerosis (MS), psoriasis, RA, SLE, and T1DM. Again, a substantial proportion (44 %) of the SNPs identified were also implicated in more than one disease [48]. The implicated SNPs clustered near deoxyribonucleic acid (DNA) sequences that encoded proteins also implicated in the same subsets of diseases [49].
Such proteins, with genes located in proximity to genes implicated in the increased risk for multiple autoimmune diseases, may represent an underlying shared mechanism that constitutes the physiological basis for disease risk. This is a theory supported by the observation that autoimmune patients frequently suffer from more than one autoimmune disorder at a time or from different autoimmune diseases during different stages of their lives [3].
Genome-wide association studies ostensibly reveal a set of genes common to many autoimmune diseases which represent little increased risk on their own [44]. Multiple polymorphisms, however, each carry a slight risk on their own, but together create a lower threshold for the development of an autoimmune event [3]. It is also possible that in rare instances, copy number [44] or somatic mutations de novo can create a genetic platform for autoimmune disease [50,51]. It is reported that somatic mutation can, in adults, generate B cells with autoreactive antigen receptors [52].
Concordance rates among monozygotic (MZ) twins, although consistently higher than those in dizygotic (DZ) twins, are low (see Table 24.1b, Part 2). This observation and the observation that in animal studies the gender bias characteristic of autoimmune disease was revealed to be strain specific suggest an interaction between sex chromosomes and the background genes [36]. It makes it clear that AID requires more than just a genetic foundation. Despite the fact that genome-wide association studies have identified multiple significant associations with specific genetic loci, concordance rates for MZ twins are still below 50 % (with a few exceptions), a statistic that suggests additional complementary mechanisms in the genesis of AID [47].
24.3 Contributions of Gender to Autoimmune Disease
The most striking feature in the diverse group of disorders that make up AID, arguably, is their definitive predominance for females [53] (see Tables 24.1a, Part 1 and 24.1b Part 2). Autoimmune disease is one of the top ten causes of death in women under the age of 65 [54], the second highest cause of chronic illness, and the top cause of morbidity in women in the United States [55]. Suspicion with regard to the physiological basis for the dramatic surfeit of female AID patients has rested primarily on the female sex hormone estrogen [56], with lots of evidence to support it [46]. The role of estrogen in immune function as well as autoimmune disease is supported by a substantial body of evidence [42].
24.3.1 Estrogen
The immune response is heightened in women as compared to men [57]. Both cellular and humoral immune responses are more vigorous in women than men, making women more resistant to infection, but also more subject to autoimmune diseases [41]. Women typically respond to infection (natural and by vaccination) as well as trauma with the increased antibody production characteristic of a T-helper 2 cell (Th2) immune response, while men respond with a T-helper 1 cell (Th1) response, characterized predominantly by inflammation [58]. Estrogen’s effect on T-helper cells appears to be estrogen dependent. Low doses of estrogen stimulate Th1 response and higher doses, Th2 [59], a phenomenon also driven by increasing levels of circulating estrogen of pregnancy. Such a shift is also observed during pregnancy [60]. The Th2 response, wherein estrogen stimulates Th lymphocytes to secrete type II cytokines, thereby promotes synthesis of antibodies [61].
Estrogen drastically reduces the size of the bone marrow cavity and induces significant atrophy of the thymus, sites where deletions of autoreactive cells occur. Estrogens are known to stimulate lymphopoiesis outside of marrow (promoting auto reactivity by bypassing the normal selection process) [62], so B cells may develop at alternative sites (liver and spleen) where less stringent selection is occurring. Mice treated with endogenous estrogen have extensive hemopoietic centers in the liver and spleen with B-cell activation including autoantibody-rousing cells in the spleen and liver [42].
Estrogen, a principal regulator of proinflammatory molecules [52], is known to exert numerous effects on individual immune parameters (Table 24.4).
Table 24.4
Biological functions of estrogen in the immune system
Immune function | Immune characteristic | References |
---|---|---|
B cell | Induction of antibody production | [41] |
Produces higher overall immunoglobulin levels | [42] | |
Promotes antibody response to foreign antigens | [42] | |
Increases IgG and IgM levels | [61] | |
Produces polyclonal induction of B cells in vitro | [63] | |
Alters existing thresholds for B-cell apoptosis as well as B-cell activation | [64] | |
Induces rapid maturation of B lymphocytes | [41] | |
T cell | Downregulates the number of immature T lymphocytes | [65] |
Stimulates involution of thymus | [65] | |
Stimulates production of regulatory T cells (Tregs) | [66] | |
Downregulates CD4 T cells | [42] | |
Stimulates an increase CD4/CD8 ratios | [42] | |
Stimulates T-cell activation markers | [52] | |
Stimulates proliferation of T cells | [52] | |
Other cell types | Stimulate proliferation of macrophages | [52] |
Decrease the number of monocytes | [67] | |
Cytokines and effector molecules | Stimulate proinflammatory serum markers | [68] |
Downregulate soluble cell adhesion molecules in women | [69] | |
Inhibit expression of monocyte chemoattractant protein-1 | [70] | |
Stimulate interleukin production (IL-1, IL-4, IL-6, and IL-10 in macrophages; IL-4, IL-5, IL-6, and 1-0 in Th2 cells | [41] | |
Stimulate cellular response to cytokines | [42] | |
Stimulate secretion of IL-4, IFN-γ and IL-1 | [42] | |
Stimulate production of antiapoptosis protein Bcl-2 | [71] | |
Inhibit IL-1-induced expression of cell adhesion molecules | [70] | |
Inhibit of TNF-α expression. | [72] | |
Downregulate soluble TNF-α | [73] | |
Suppress IL-2 secretion by T cells as well as receptor expression in activated peripheral blood T cells | [5] | |
Induce Th2 pathway cytokines, downregulate Th1 | [52] | |
Inflammatory chemokines (MCP-1, MCP-5 as well as NO synthase [iNOS4] and cyclooxygenase-2) | [74] | |
Systemic | Stimulates resistance to certain infections | [42] |
Increases antibody-secreting cells (IgG and IgA) | [46] | |
Downregulates induction of tolerance | [42] | |
Stimulates graft rejection | [42] | |
Suppresses apoptosis | [52] |
Numerous intrinsic (e.g., pregnancy, menopause, disease states) and extrinsic (e.g., oral contraceptives, hormone replacement therapy [HRT]) factors influence serum estrogen levels. The course of autoimmune diseases typically fluctuates in parallel to changes in estrogen levels [46]; the role that sex hormones play is clearly evidenced by dramatic differences in prevalence related to circulating levels of estrogen over the female life span [42].
Autoimmune hepatitis (AIH), for example, often ameliorates during pregnancy, with an increase in first-time diagnoses in the postpartum period [75,76]. The severity of MS as well as RA decreases during pregnancy, particularly in the third trimester when hormone levels are highest [46].
SLE varies over the course of the menstrual cycle, with flares much more likely during peak estrogen periods such as the high estrogen levels of pregnancy [52]. Similarly, in vitro fertilization procedures and drugs that induce ovulation also induce SLE flares or the onset of SLE symptoms [52]. New diagnoses and flares of existing SLE are rare in the postmenopausal period [52].
Treatment of human peripheral blood mononuclear cells (PBMCs) from SLE patients enhanced total immunoglobulin G (IgG) production as well as anti-double-stranded DNA (dsDNA) autoantibody levels; PBMCs from healthy individuals, however, did not; the disparity proved to be interleukin (IL)-10 dependent [77]. Testosterone significantly inhibited IgM and IgG production by PBMC [78].
Similar estrogen effects were observed in animal models [46]. Animal studies confirm that hormonal manipulations can influence disease expression [53]. In a murine lupus model of experimental autoimmune encephalomyelitis (EAE) in which male mice were castrated, castration produced SLE with disease parameters very similar to that in female mice, while ovariectomized females produced disease parameters similar to male mice of the same strain (NZBxNZW) F1 [46]. Estradiol protected the female mice from EAE.
In other animal studies, five different genes, all regulated by either estradiol or dihydrotestosterone, contributed to autoimmunity (in vivo mouse model with lupus) [79].
The importance of the hormonal component in autoimmunity was illuminated by an experiment in mice, which isolated the chromosomal from the hormonal component by moving the testis-determining gene SRY from the Y chromosome to an autosomal chromosome [80]. This approach revealed that although the XY genotype alone stimulated autoantibody proliferation, androgens exerted an overriding suppressive effect [81]. Male sex hormones appear to mask the otherwise immunostimulatory influence of the XY genotype.
In contrast, however, estradiol increases susceptibility to experimental myasthenia gravis, experimental autoimmune uveoretinitis, and lupus [5]. The effects of estrogen on female-predominant AIDs, however, are not generalizable—some are abrogated by testosterone and some are amplified [82]. Gender differences in autoimmunity are at least partially mediated by regulation at the level of the estrogen receptor.
24.3.2 Estrogen Receptors
Nuclear receptors are factors that bind to DNA and act to regulate gene expression at the transcription level (thus known also as transcription factors) and include receptors for estrogens and androgens [52]. Estrogen (17-β estradiol), which is the principal estrogen in circulation during the reproductive years, acts as a ligand for two different nuclear receptor proteins, thus forming two functionally distinct estrogen receptors, estrogen receptor alpha (ERα) and estrogen receptor beta (ERβ), which exist in both homo- and heterodimers.
Estrogen receptors translocate to the nucleus where they bind to estrogen-responsive elements (EREs) in gene promoters and thus act as an on/off switch for gene transcription. The estrogen/estrogen-receptor (E/ER) complex may also interact with other transcription factors such as NF-Κβ, which is capable of binding to non-ERE sites in other promoters [83].
Both types of estrogen receptors are found all over the body, including the ovary, womb, breast, bone, and immune cells, T and B cells, dendritic cells, neutrophils, macrophages, natural killer (NK) cells, thymic stroma cells, bone marrow, and endothelial cells [83].
Although both ERα and ERβ both act to modulate gene expression of estrogen-responsive genes, they modulate that expression in very different ways [52]. ERα deficiency in a murine model of lupus, for example, resulted in disease abrogation and prolonged survival, while ERβ deficiency had minimal effect [84].
Estrogen receptors also occur in two places, with distinctive purposes. Membrane-associated ERs amplify signal transduction cascades, where nuclear ones induce gene transcription. Mature lymphocytes express membrane-associated ERs which are activated by estrogen to invoke increased calcium flex in antigen-activated cells [83].
The effects of estrogen-receptor regulation of immunity and their implications for autoimmunity are many. In one study, estrogen-receptor blockers blocked estrogen-receptor activation in lupus T cells but not in healthy controls [85]. Decreased ERα in macrophages resulted in the stimulation of a cluster of differentiation 4 (CD4) cells [82]. Estradiol (E2), acting via ERα, increased proinflammatory cytokine expression, enhanced proliferative and interferon alpha (INF-α) production by CD4+T cells [82], and differential expression of ER-α and ER-β observed in SLE and RA [83].
The presence of two ERs, occurring in two different places, adds another layer to hormonal regulation of immune processes since the same estrogen produces different effects at one receptor versus another [41], giving the body the ability to fine-tune immune response.
Estrogen, then, is believed to be involved in the regulation of a wide variety of immune cells and a key player in the dysregulation of immunity that leads to both autoimmune and autoinflammatory diseases. Paradoxically, however, comparisons of estrogen levels in female patients with different autoimmune diseases (including AID patients) showed no difference between patients and healthy controls, an indication that estrogen is just part of a more complex etiology [78,86]. In fact, estrogen is not the only component of female gender with the potential to contribute to autoimmunity.
24.3.3 Microchimerism
Fetomaternal microchimerism is the presence, after pregnancy and delivery, of foreign fetal cells in the mother. These include hemapoietic progenitor cells with the capacity to induce autoimmunity and which have been implicated in autoimmune disease, in particular, Graves’ disease [87]. It is known that fetal cells are found in peripheral blood of nearly all women during pregnancy, including hematopoietic progenitor cells with the potential to become both effectors and targets of immune processes, and that maternal DNA can be detected by polymerase chain reaction (PCR) in a majority of cord blood samples [87].
Female subjects with autoimmune thyroid disease (Graves and Hashimoto’s) are often shown to have microchimeric fetal cells in their thyroid glands [87]. In addition, women with autoimmune thyroid disease with sons had higher prevalence of male cells in their thyroid than women with sons but healthy thyroids [87].
Sixty percent of women with Graves’ disease with onset during reproductive years developed the disease in the postpartum period [87]. Also supporting microchimerism as the etiological basis of Graves’ disease is a large study that found a significant increase in thyroid peroxidase autoantibody (TPO) associated with increase in parity [88].
24.3.4 X Chromosome
Interestingly, in a murine model in which the authors artificially produced XX, XY mice without alteration of actual gonads, the XX genotype was associated with greater severity of EAE and pristane-induced lupus than XY [46], demonstrating that the sex chromosomes beyond the influence of the sex hormones themselves, contribute to autoimmunity.
The X chromosome itself is implicated in the genesis of autoimmunity, with the presence of two copies of the X chromosome in women held responsible for the observed female predominance in AID. Aberrant, congenital X-chromosome doses, as in Klinefelter’s syndrome (XXY) in men, which greatly increases the risk for female-predominant autoimmune disease, underlines the X chromosome’s importance [15]. Gene expression in X and Y, however, is not well understood. The fact that the X chromosome itself contains 1,000 unique genes, including genes associated with systemic sclerosis, autoimmune thyroid disease, and SLE [89], in and of itself infers a substantial role of the X chromosome in the regulation of autoimmunity.
In women, one X chromosome is habitually but randomly inactivated to preserve equal gene expression in men and women. However, the inactivated X is not completely inactivated, meaning that some X-associated genes are overexpressed in women [46] as compared to males. Partial inactivation of X, then, may contribute to gender disparity in AID. The mouse strain BXSB, with a translocation mutation in which part of the X chromosome has been transferred to the Y, develops lupus-like syndrome with high frequency in males, shown to involve overexpression of Toll-like receptors (TLR)-7, shown to be a key player in lupus development [46].
In addition, although the choice of which X chromosome to inactivate is random, certain cell types are preferentially inactivated or maintained, resulting in “skewed inactivation” that acts to modulate gene expression. A high percentage of women with systemic sclerosis display skewed X inactivation [46]; skewed X-chromosome inactivation is also implicated in scleroderma in association with reduced regulatory T cell (Treg) activity [83].
Finally, there is sometimes spontaneous loss of the X chromosome with age, observed in increasing frequency with age in blood cells of patients with systemic sclerosis and autoimmune thyroid disease [46]. The origin and the effect of this spontaneous loss are unclear.
Beyond issues of gender created by the gene complement of sex chromosomes and hormones that the complement stimulates, environmental interactions contribute to autoimmunity as well.
24.4 The Contribution of Environmental Agents in Autoimmune Disease
The fact that monozygotic pairs of female twins (with identical genetic and hormonal constitutions) can attribute only 20 % of their phenotypic variance to their shared genetic polymorphisms implicates environmental exposures in the development of autoimmune disease [3]. These exposures are numerous and contribute to a variety of autoimmune diseases. Geographic clusterings of specific diseases are observed, with wide disparities in prevalence in different parts of the world. Even in the confines of the US, multiple sclerosis has dramatic geographical specificity, with a prevalence of 61/100,000 in the West, compared to only 3/100,000 in the South [33].
Autoimmunity is associated with seemingly improbable environmental factors like season of birth or weather patterns [3].
Many environmental factors, both intrinsic and extrinsic, are associated with the development of autoimmune disorders (Table 24.5). The internal biological environment (e.g., endogenous hormones and aging) obviously contributes to autoimmune disease; extrinsic insults, including environmental agents like ultraviolet (UV) light, chemical or other occupational exposures, pollutants, or health habits like smoking or alcohol consumption, are now recognized as important components of autoimmune disease as well. Environmental factors may initiate immunity through nonspecific activation of resting T cells, modification or release of previously sequestered proteins, cross-reactivity between virus and self-protein (molecular mimicry), and modulation of gene expression [5]. Viruses and other infectious agents are also potential environmental inducers of AID as well, although, since viral infection can occur years before the actual onset of a particular AID, definitive associations are difficult to establish [5].
Table 24.5
Environmental triggers for autoimmune disease and their epigenetic effects
Environmental exposures | Action | References |
---|---|---|
Methionine, S-adenosylmethionine | Cofactor involved in DNA methylation | [90] |
Folic acid | Cofactor involved in DNA methylation | [91] |
Choline | Source of methyl group in DNA methylation | [92] |
Betaine | Methyl donor in DNA methylation | [92] |
Resveratrol | Inhibits HDAC | [93] |
Dietary fat | DNA methylation | [94] |
Nickel | Inhibits DNA methyltransferase | [80] |
Vinclozolin (fungicide) | DNA methylation in germ line | [95] |
Methoxychlor (pesticide) | DNA methylation in germ line | [95] |
Pollutants | DNA methylation | [96] |
Asbestos | Increases methylation | [80] |
Alcohol consumption | Hypermethylation of promoter for HERP gene | [80] |
Tobacco | Hypermethylation of tumor-suppressor gene in lungs, methylation of other tissues | [80] |
Marijuana | NA | |
Stress (mice) | Increase methylation in brain-derived neurotrophic factor gene in hippocampus | [98] |
Potential contributions to autoimmunity from the environment are virtually endless and differ widely with occupation, place of residence, hobbies, and medical history. Environmental interactions, like diet, occupation, exposure to environmental chemicals, radiation, UV light, pathogenic organisms, and medications, can also differ by gender [36] and contribute to gender disparities in AID prevalence [99]. Interestingly, the lupus-like syndrome produced by the drug procainamide has a male to female ratio of 2:1, while in asymptomatic patients, the ratio is 5:1, implying an as yet unresolved interaction between gender determinants and susceptibility to an environmental exposure, procainamide, in the development of lupus [36].
Environmental insults may increase with age, including cortisol levels, sleep dysregulation, decrease in physical activity, increase in oxidative stress, or nutritional deficiencies [100].
24.5 Immunosenescence and Autoimmune Disease
The immune system undergoes extensive remodeling as humans age, characterized by a progressive deterioration in the ability to mount an effective immune response. However, an unexplained paradox is a simultaneous increase in autoantibody production [43]. The clinical consequences of this remodeling include a risk of neoplasia and infectious disease, as well as autoimmune disease [43]. Immunosenescence is a subject of increasing interest in medicine, as life expectancy, especially in developed countries, increases without a parallel improvement in health in old age [101].
The immune system, a complex and exquisitely regulated collection of interdependent molecular pathways of both innate and adaptive defense, begins to undergo dysregulation of cell homeostasis. This causes multiple changes in immune function which increase susceptibility to the development of autoimmune diseases [43] (Table 24.6). Old age is characterized by the rising incidence of autoimmunity [103] and an associated increase in both levels of circulating antibodies and numbers of specific antibodies in circulation. Persistent high antigen levels activate memory cells, and costimulatory T cells become less susceptible to downregulation [117].
Table 24.6
Changes in immune function with age (both men and women)
Immune function | Change | References |
---|---|---|
B cell | Reduction in early progenitor B cells in bone marrow | [102] |
Increase in polyclonal response against diverse mitogens | [43] | |
Decrease of specific-antigen response | [43] | |
Production of antibodies independent of T-cell activation | [101] | |
Oligoclonal expansion of B cells | [101] | |
Increase in B1 cell fraction of B cells | [103] | |
Maturation of B cells blocked (believed to have inability to rearrange Ig genes because of the decrease in expression of RAG-1 and RAG-2 recombinases) | [104] | |
Reduced output of naïve B cells | [102] | |
Accumulation of oligoclonally expanded, functionally incompetent memory lymphocytes | [102] | |
Decreased numbers of circulating B cells | [105] | |
CD20+ B cells decrease | [106] | |
Reduced antigen-recognition repertoire of B cells | [107] | |
Class switch alterations in immunoglobulins produced | [108] | |
Stimulated B cells show significant alterations in cellular structures with a role in signal transduction | [109] | |
Increase of IgA and several IgG subclasses | [110] | |
Lymphocytes | Reduction in total numbers | [111] |
Molecular | Alterations in cell-surface receptors (e.g., loss of costimulatory receptor CD28 expression in CD8+ cells) | [112] |
Alterations in cytokine and cytokine receptor alterations in T cells | [113] | |
Alterations in effector molecules | ||
Interleukin 6 levels increase | [116] | |
Alterations in transcriptional regulators | [114] |
Although immunosenescence affects all cell types and results in deterioration of both humoral and cellular immunity and multiple cell types, age-associated cumulative effects on T-cell function are the most dramatic and most detrimental [118]. With age, there is a decrease in thymic epithelial space and thymic cellularity, called thymic involution; in humans, the increase in perivascular thymic space is replaced progressively with fat [101]. Humoral immunity as well is severely compromised in the aged as a result of decreased production of long-term Ig-producing B cells and the loss of immunoglobulin diversity and affinities [101].
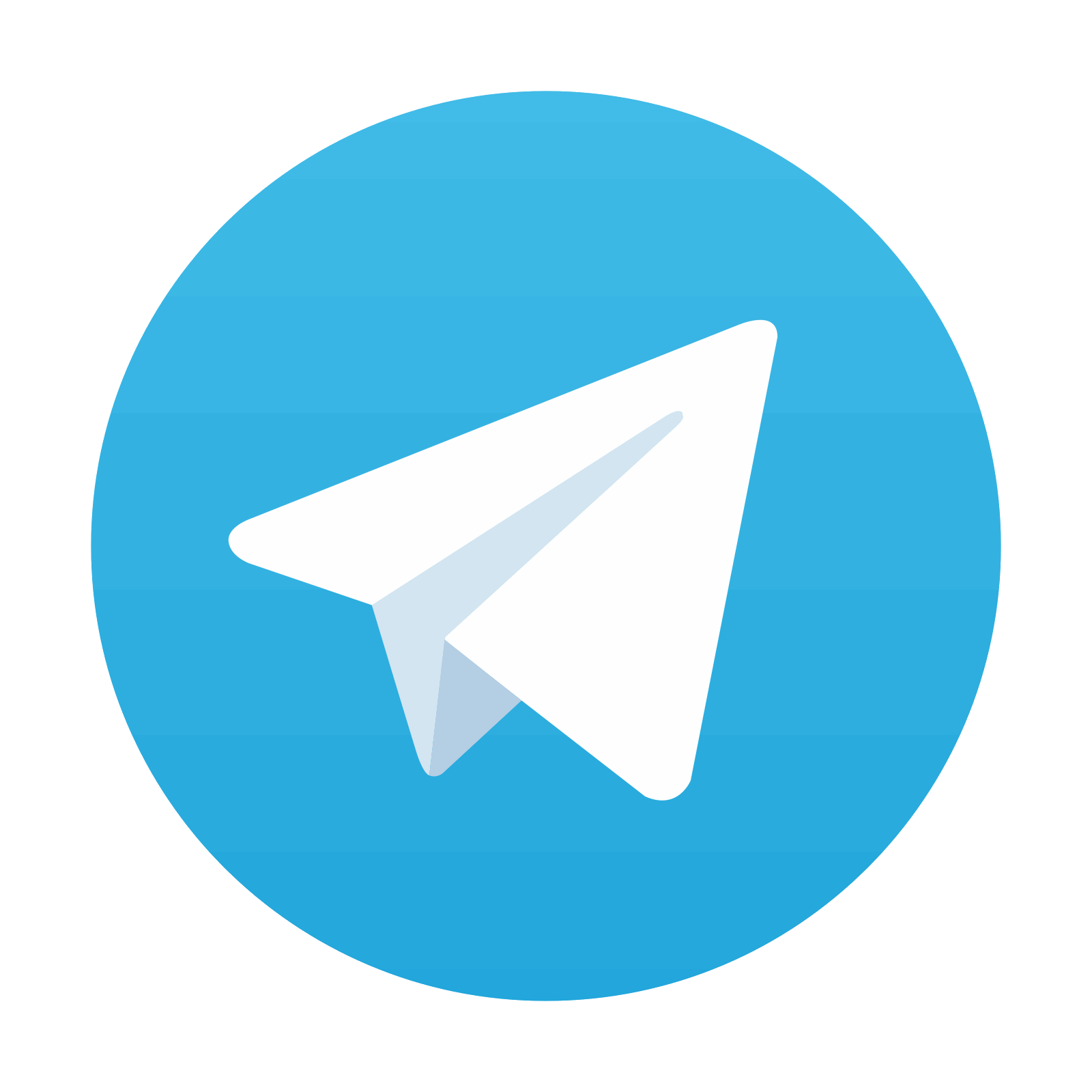
Stay updated, free articles. Join our Telegram channel

Full access? Get Clinical Tree
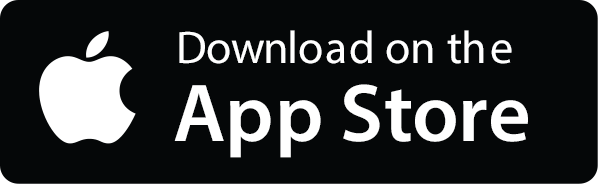
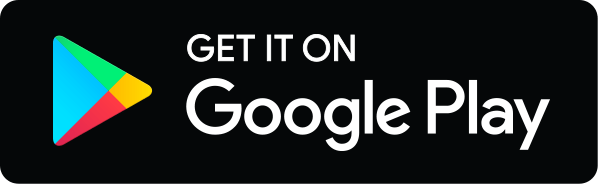