This article introduces the plastic surgeon to the basics of high-resolution ultrasound (HRUS) for screening breast implants. It describes how HRUS has become an accepted alternative to MRI for screening and diagnosis, and how plastic surgeons should use this new technology for the benefit of their patients and their practices. Basic principles, technology, and nomenclature are presented. Key steps to obtaining diagnostic images of breast implants are reviewed, including typical artifacts and sources of error. Imaging examples are presented. The article ends with a review and a step-by-step guide for plastic surgeons looking to use the technology.
Key points
- •
High-resolution ultrasound (HRUS) is a safe, effective modality for screening breast implants.
- •
The basic concepts are straightforward and the images are intuitive once those concepts are understood.
- •
Plastic surgeons are fully capable of performing HRUS examinations for breast implant screening purposes, given their understanding of the anatomy and the physical construction of the implants.
- •
HRUS provides benefits to the surgeon’s patients and their practice.
Clinical and regulatory background
Silicone gel breast implants are approved by the US Food and Drug Administration (FDA) for use in cosmetic breast augmentation, breast reconstruction procedures following cancer or trauma, and correction of severe breast abnormalities or previous reconstructive failures. Largely due to their more natural feel and aesthetic look, silicone gel implants are elected in more than 95% of breast reconstruction cases that use an implant and 87% of cosmetic breast augmentation cases. Despite their prevalence and popularity of use, however, there are some drawbacks of silicone gel implants, including the possibility of silent rupture (rupture undetectable by physical examination). The FDA recommends the surgical removal and possible replacement of any ruptured implant.
As with other medical devices, silicone gel breast implants have a finite life span. Clinically apparent implant rupture is reported in published literature to occur at rates up to 35% or as low as 2%. The FDA had removed all silicone gel–filled breast implants from the market in 1992, and when the FDA allowed silicone breast implants back on the market in 2006, it included a monitoring provision that recommended that patients undergo MRI scans to detect silent rupture 3 years after implantation, and every 2 years thereafter for life. However, a 2018 postimplantation study of nearly 100,000 patients found that such MRI surveillance occurs at a rate of less than 5%, likely due to the prohibitive costs associated with MRI scans.
As an alternative to MRIs, high-resolution ultrasound (HRUS) is cost-effective, quick, and suitable for routine use in an office setting. Although ultrasound has been used to study silicone breast implants for decades, recent advances have improved the image quality, affordability, and convenience. HRUS is also proven to be as accurate as MRI scans for silent rupture of silicone gel implants. In 2012, Bengtson and Eaves showed that MRI, surgeon-performed HRUS, and radiologist-performed HRUS scans were all accurate in predicting implant shell integrity in 29 of 29 imaged breasts (100%), as confirmed at the time of surgery in both symptomatic and asymptomatic patients. Rietjens and colleagues showed that the negative predictive value of ultrasound when evaluating breast implants for ruptures was 85%, meaning that in the case of negative ultrasound findings, MRI may be avoided.
In a recent study, the sensitivity and specificity of ultrasound were 90% and 80%, respectively, whereas the MRI sensitivity and specificity were 87% and 85%, respectively. The investigators of this study concluded that ultrasound evaluation may be the first-level examination in case of suspicious symptomatic breast implant rupture. Further, in a study whereby the implants that were evaluated using ultrasound had ultrasound-specific raised markers, all those markers were found in all 69 patients.
Most recently, the FDA issued a draft Guidance Document with new recommendations on screening patients for implant rupture. Notably, the FDA has accepted ultrasound as an alternative for screening asymptomatic patients, based on the studies described previously. The Guidance replaces the current FDA MRI screening recommendations with a new protocol requiring that, for asymptomatic patients, the first ultrasound or MRI should be performed at 5 to 6 years postoperatively, then every 2 years thereafter. For symptomatic patients or patients with equivocal ultrasound results for rupture at any time postoperatively, an MRI is recommended. These recommendations are to be incorporated into both the Physician Labeling and the Patient Labeling for silicone implants.
Based on this, it is incumbent on the plastic surgeon community to become more familiar with ultrasound technology, how it is used to screen for breast implant issues, and the benefits that it brings to patients and to physician practices. Ultrasound screening for breast implant rupture does not require a radiologist’s interpretation, just basic familiarity with ultrasound concepts, sufficient hands-on training on the part of the surgeon or their staff, and repetitive clinical use. Because plastic surgeons are intimately familiar with the specific anatomy of the breast, the physical construction of breast implants, and the nature of implant ruptures, they are uniquely qualified to leverage basic ultrasound capabilities for screening purposes. Because the relationship between the ultrasound image and the implant is very intuitive, a routine screening examination can be done in just a few minutes and incorporated into preoperative and postoperative visits. Breast implants present a relatively straightforward ultrasound imaging situation: (1) they are very distinct from the surrounding tissue; (2) their general appearance is consistent in terms of depth, location, brightness, and imaging characteristics; (3) ruptured implants can be differentiated from intact ones because of the unique “image signatures” of the shell and implant material; (4) unlike static MRIs, ultrasound examinations are dynamic, and specific physical manipulations can be done during the examination to confirm questionable findings such as folds and creases.
This article presents the basics of ultrasound technology as applied to scanning breast implants. The technology description is only a brief survey of the topic, and the reader is directed to other references for more in-depth treatments of basic ultrasound principles , or more advanced techniques applied to breast implant monitoring.
Ultrasound technology
For millennia, animals, such as bats, dolphins, and whales, have used sound to avoid obstacles and detect prey. They make sounds that bounce off of targets they are interested in, such as food, and they listen for the echoes. Based on the direction of the echo and the time taken for its return, they can estimate how to turn and how far to go. Scientists and biologists have only recently begun to appreciate the sophistication of their techniques for creating, focusing, receiving and processing sound.
Medical ultrasound systems work on this same basic principle. An electronic system generates a focused, pulsed sound wave, transmits it into a patient, and then waits for the echoes to return. Because sound travels at a known speed in tissue, the time between sending out the sound and receiving it back can be converted into a distance. (Note that this is the same as counting the time between seeing a lightning flash and hearing the thunder to estimate the distance to the storm. The time delay caused by the sound traveling at a fixed speed is directly related to the distance.) Based on the direction and depth of the echo, the system converts the information into an image representing the sound reflection pattern. Specifically, the system determines the time to a specific received echo, and puts a corresponding bright spot on the image at a depth corresponding to that time. The brightness of the spot matches the strength of the received echo, so stronger echoes (from larger or more prominent tissue structures) are brighter. The longer the time between sending out the pulse and receiving the echo, the deeper the spot appears on the image.
The specific components of the ultrasound system ( Fig. 1 ) include the imaging display and control console, the ultrasound unit itself containing electronics to generate and process the ultrasound signals, and a transducer that converts electrical signals into sound energy and back again. Depending on the clinical requirements, ultrasound systems can be as large as several hundred pounds, with multiple displays and transducers, or small enough that the entire system is handheld. The basic ultrasound imaging technology is called “B-mode,” and the image itself is called a B-scan. Other modes include A (used in ophthalmology), M or TM (used in cardiology), C (rarely used clinically), and D (Doppler, used to detect bloodflow). More sophisticated systems not only produce images of tissue structures, but can also detect bloodflow or tissue stiffness or provide 3-dimensional images. Irrespective of their specific design, all these systems rely on the same fundamental principles of ultrasound.

Basics of ultrasound imaging
Ultrasound, or sound above the range of human hearing, is a vibration or pressure wave, traveling through media. It is composed of higher pressure (compression) and lower pressure (rarefaction) regions that alternate as the wave travels. The wave is therefore a form of mechanical energy. To travel properly, there needs to be a continuous mechanical connection between the source of the ultrasound (the transducer) and the imaging target (tissue structures). Thus ultrasound will not travel through air, and all medical ultrasound scanning requires coupling gel to maintain good mechanical connection.
Ultrasound is characterized by the frequency of the wave, that is, the number pressure variations per unit of time. The unit of frequency is the Hertz (Hz), which is the same as cycles per second, so for example, ultrasound at 1 MHz oscillates at 1 million cycles per second. Diagnostic medical ultrasound operates in the frequency range of 2 to 30 MHz, and ultrasound transducers are usually referred to by their operating frequency, that is, “a 12-MHz transducer.” The choice of the transducer frequency is critically important in ultrasound imaging, because the higher the frequency, the better the resolution. This is because the frequency determines the wavelength, which is the volume of space occupied by one wave. The smaller this volume, the better the resolution, as the imaging system cannot distinguish among targets that are closer together than the wavelength. Put more formally, the frequency and the wavelength are related by the speed of sound in tissue:
λ=c/f
However, higher resolution comes at a cost: the higher the frequency, the greater the attenuation (loss of energy) in the wave. Ultrasound attenuation is the combination of the absorption (related to the dynamic viscoelastic damping of the tissue), and its scattering or inhomogeneous properties. Energy loss in the body can be extremely high (bone) or low (amniotic fluid). For example, when imaging through 2 cm of breast tissue, the attenuation is more than 60 times greater at 10 MHz than at 1 MHz. So, the higher the frequency, the shorter the range of tissue depth that can be imaged successfully. Table 1 summarizes the ultrasound energy loss per centimeter due to attenuation and the resultant imaging depth as a function of frequency. HRUS is generally classified as having a frequency of 10 MHz or higher. This provides resolution of 150 μm or better, but at the same time, is limited to a maximum “penetration depth” in tissue of 5 to 6 cm. Thus, it is well suited for imaging of breast implants, but not for deep abdominal regions.
Frequency, MHz | Energy Lost Every Centimeter, % | Imaging Depth, cm |
---|---|---|
2.0 | 21 | 30 |
5.0 | 44 | 12 |
10.0 | 68 | 6 |
12.0 | 75 | 5 |
15.0 | 82 | 4 |
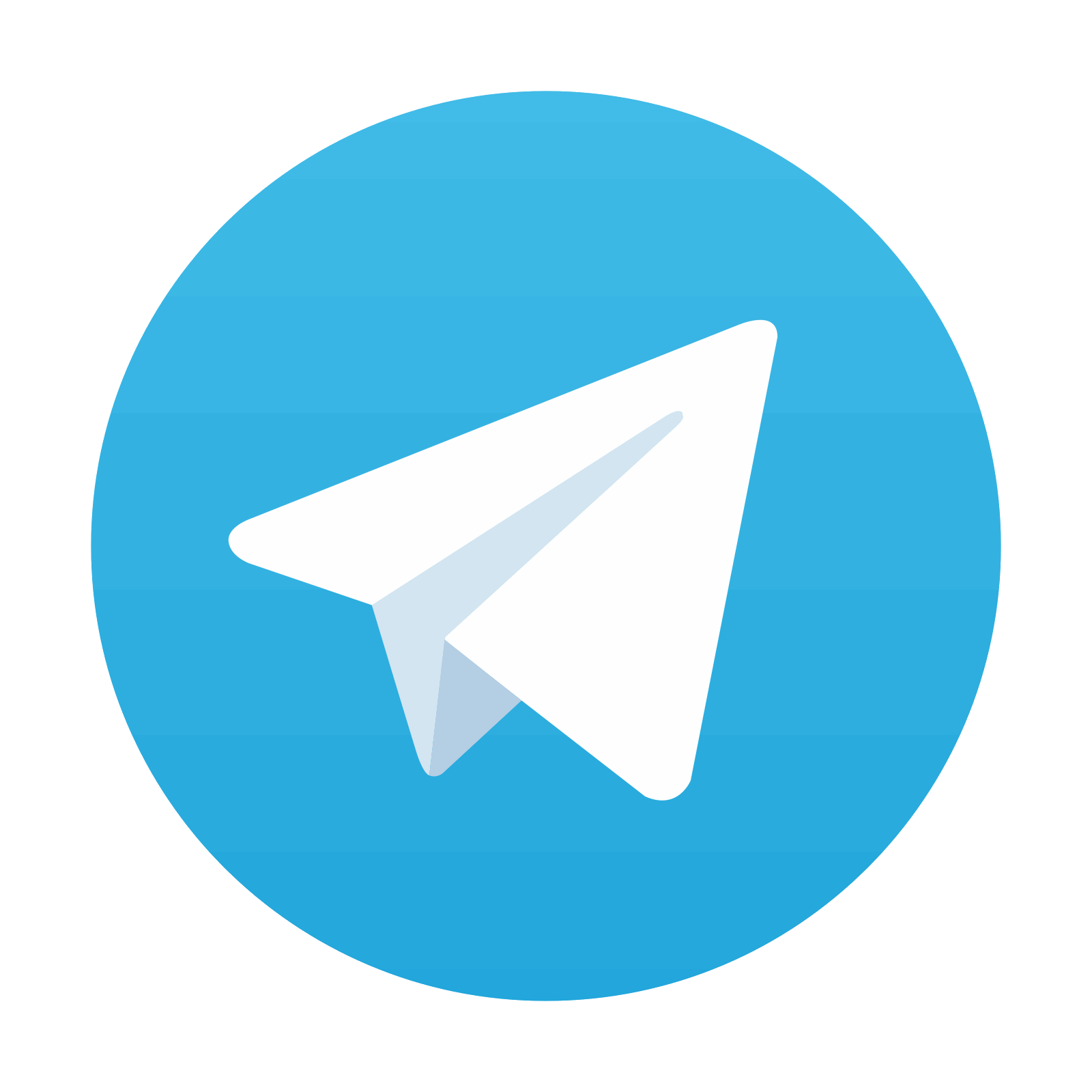
Stay updated, free articles. Join our Telegram channel

Full access? Get Clinical Tree
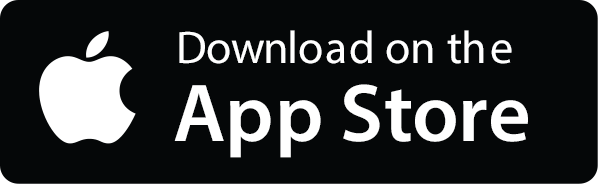
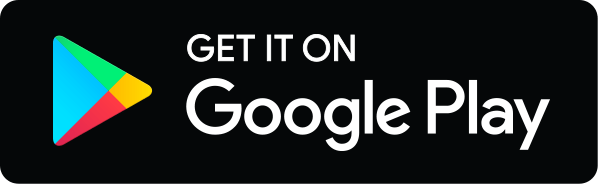
