Fig. 19.1
Rat orthotopic hind-limb transplant model for peripheral nerve regeneration. The sciatic nerve is repaired with 10–0 epineurial suture 1 cm proximal to the trifurcation into the tibial, peroneal, and sural nerves
The rat orthotopic hind-limb transplant model with sciatic nerve approximation provides the opportunity to utilize a number of assessment tools for the measurement of peripheral nerve regeneration, muscle innervation, and functional outcomes. Nerve histomorphometry allows for direct measurement of axonal regeneration beyond the repair site [10]. At the time of sacrifice, the sciatic nerve harvested and fixed, typically in glutaraldehyde. Ultrafine cross sections taken distal to the approximation site are then stained for myelin, allowing for visualization and quantification of myelinated axons. Measurement parameters include total axon number, width, and density, among others. Results from nerve histomorphometry taken in isolation can be misleading, as it is impossible to differentiate meaningful axonal regeneration resulting in end-organ innervation from axonal sprouting which is of little benefit [11]. For this reason, nerve histomorphometric results should be considered in the context of other data related to end-organ reinnervation and functional outcomes (Fig. 19.2).
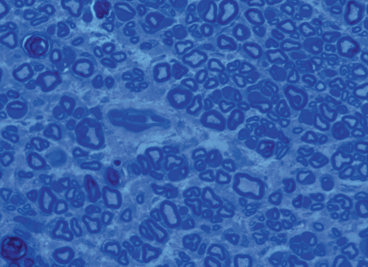
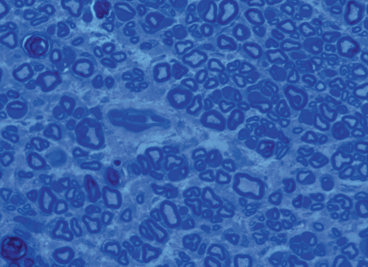
Fig. 19.2
Nerve cross-sectional histomorphometry demonstrating concentration of myelinated axons allowing for objective quantification
Retrograde labeling techniques allow for measurement of the number of cell bodies within the recipient’s spinal cord that are contributing to axonal regeneration into the graft [12, 13]. This technique involves severing the sciatic nerve distal to the approximation site and applying fluorescent tracer to the proximal nerve end. Over the course of the next 2–3 days, any viable axons that have regenerated into the graft will transport the tracer in a retrograde fashion into their cell bodies within the spinal cord ventral horn or dorsal root, for motor or sensory axons, respectively. At the time of sacrifice, the fixed spinal cord can then be sectioned and imaged for quantification of the number of fluorescing cell bodies (Fig. 19.3).
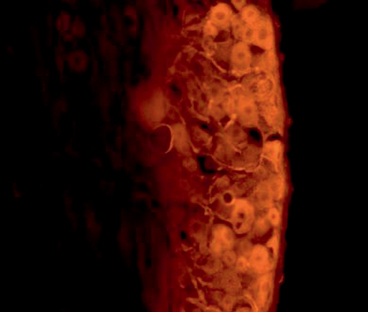
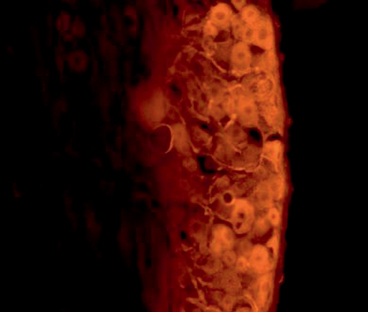
Fig. 19.3
Example of retrograde labeling technique demonstrating cell bodies in the ventral root of the spinal cord which exhibit fluorescently radiolabeled substrate administered at the site of repair
Direct visualization and quantification of neuromuscular junctions can also be performed as a measure of muscle reinnervation [14]. To do this, motor end plates are stained with α-bungarotoxin and axonal neurofilaments stained with synaptophysin. Alternatively, transgenic rats that express axonal green fluorescent protein (GFP) can be used for this purpose. Following staining, the number of neuromuscular junctions, indicated by overlapping staining of neurofilament and motor end plate, can be counted (Fig. 19.4).
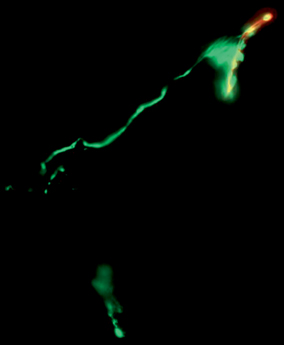
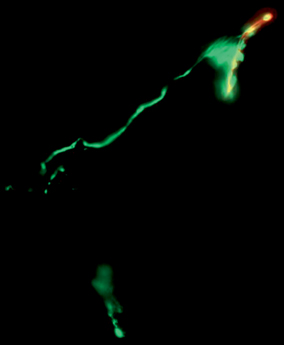
Fig. 19.4
Neuromuscular junction staining demonstrating reinnervation at the motor end plate (stained in red with alpha-bungaratoxin) by terminal axons (stained in green with synaptophysin)
Perhaps the most important outcome measures related to peripheral nerve regeneration are those that measure the ultimate functional outcomes resulting from the end-organ reinnervation. A number of functional assessments are behavioral in nature, in that they require rats to perform a specific activity. The sciatic functional index (SFI) is a measure of distal muscle innervation [15, 16]. It is based on the premise that the progressive reinnervation of ankle plantar flexors and the intrinsic muscles of the foot will gradually affect the footprint left during walking in a predictable manner. Specifically, the footprint length should progressively shorten with improved plantar flexion, and toe spread should increase with reinnervation of the intrinsic muscles of the foot. These measurements are used to calculate the SFI, which is reported as a ratio of the operated to the unoperated side. This technique is limited by a number of potential complicating factors. Relying or a rat to walk in a consistent manner without dragging the injured leg can be difficult. Furthermore, foot contractures occur frequently as the extrinsic muscles of the foot become reinnervated, and these contractures preclude the use of the SFI because the toes remain fully flexed, obscuring the toe spread that would otherwise be observed with distal reinnervation [17]. Lastly, this technique typically involves applying paint, or another type of dye, to the feet of the rat and can therefore be complicated by smudging and smearing of the paint.
More recently, a number of dynamic gait-analysis techniques have been developed that allow for measurement of a number of gait-related parameters. Some of these methods involve video recording the rat’s gait with sensors in place on each hind-limb joint. The location of each sensor can then be measured in relation to the other sensors over time. From these measurements, a number of derivative calculations can be made regarding angular velocity and displacement at each joint at different phases of the gait cycle [18]. Calculation of the time the foot is in contact with floor throughout the gait cycle has also been shown to correlate well with reinnervation [19, 20]. The application of this technology can be difficult due to the behavioral variables involved. Variability in gait can occur for a number of reasons unrelated to nerve regeneration and achieving consistent and reliable results from gait analysis is challenging, often requiring behavioral training. However, some have been able to achieve reliable and consistent data using these techniques [21].
Electrophysiologic neuromuscular testing provides another useful option for assessing functional outcomes in animal models [22, 23]. As opposed to other modalities used to assess a function, electrophysiologic studies do not rely on behavioral input from the animal and benefit from the lack of behavioral variability. Nerve conduction studies (NCS) can be used to measure compound nerve action potentials (CNAPs) [24]. CNAP latency is a measure of the conduction velocity generated by the fasted conducting fibers, and CNAP amplitude is a measure of the number of conducting axons. Electromyographic measurement of compound muscle action potentials (CMAPs) can also be obtained to assess the relative number of functional neuromuscular junctions that have been formed. The results, however, must be interpreted with caution as motor unit size can increase following injury resulting in larger CMAP readings. This phenomenon only masks differences in the number of reinnervated motor units up to ~ 25 % [25, 26]. Muscle contractile force can also be performed to assess the number of functional neuromuscular units present. This can be done by dis-inserting the gastrocnemius muscle and attaching it to a force transducer. The tibial nerve is then stimulated and the generated twitch and tetanic force are recorded [27].
Assessment of sensory recovery resulting from reinnervation of cutaneous sensory organs is performed by measuring the behavioral response to mechanical and thermal cutaneous stimuli [28, 29]. Following sensory reinnervation, rats demonstrate an involuntary withdrawal response to noxious stimuli. The withdrawal response can be recorded in a binary fashion to set stimuli, or alternatively, the threshold of stimulus required to elicit withdrawal can be measured. In the setting of VCA, complete sensory integration of the graft relies not only on peripheral innervation of target sensory organs but also on cortical plasticity. The process of cortical sensory reintegration can be measured utilizing a rat hemifacial transplant model [30]. This involves stimulating individual whiskers following transplantation and assessing for neural signals in the corresponding areas of the somatosensory cortex.
The effects of the acute rejection on peripheral nerve regeneration are still poorly understood in the setting of VCA. Utilizing a full major histocompatibility complex (MHC) mismatch between donor and recipient rats (i.e., Brown Norway to Lewis) provides an opportunity to assess the alloimmune response seen clinically in VCA. With withdrawal and reintroduction of immunosuppression, rejection episodes can be introduced in a controlled manner and the resulting effects on peripheral nerve regeneration and functional outcomes can be assessed [31].
Clinical Evaluation of Nerve Regeneration in VCA
Peripheral nerve regeneration continues to be one of the most difficult problems faced in the regenerative medicine today [32, 33]. Traditional axioms have set the bar low for functional expectations after severe nerve injuries and/or reconstructions, though more recent clinical experience and newer techniques may begin to challenge this notion.
Clinical evaluation of nerve regeneration is not as straightforward as one may think due to the multiple problems that nonfunctioning nerves may cause. A nonfunctional nerve does not only cause problems from its absence of function but may impart its own impairment from pain, sympathetic, and/or sudomotor dysfunction. The clinical evaluation of nerve regeneration should combine functional testing, patient outcome reported testing, and more objective neurophysiologic and/or imaging-based testing (Table 19.1). Additional information that is helpful in understanding and predicting clinical outcomes in nerve regeneration are the underlying principles of the speed of nerve regeneration, expected time course for possible motor reinnervation, and expected time course for possible sensory reinnervation.
Table 19.1
Clinical evaluation of nerve regeneration
Category | Test |
---|---|
Functional testing | Sensory: Semmes–Weinstein monofilaments, static and moving two-point discrimination, vibration, temperature, British MRC score of sensory recovery, sharp/dull discrimination, texture and shape discrimination, etc Motor: British MRC score of motor recovery, specific strength measurements per body part affected (e.g.,. pinch and grip strength for forearm/hand) Sudomotor: quantitative sudomotor axon reflex test (QSART), thermoregulatory sweat test |
Pain | Visual analogue scale (VAS), numerical rating for pain, McGill pain Questionnaire, cold intolerance questionnaires |
Patient reported/outcomes testing | Self-reported disability and pain measures (e.g., disabilities of the hand, arm, and shoulder (DASH) for upper extremity nerve injuries, Michigan Hand Outcomes Questionnaire, etc) |
Objective testing | Neurophysiologic: Nerve conduction studies (NCS), electromyography (EMG) Imaging: Myelography, magnetic resonance neurography (MRN) with and without diffusion tension imaging (DTI), high-resolution ultrasound (HRUS) |
On a fundamental level, we estimate the speed of nerve regeneration to occur at approximately 1 mm/day in healthy young adults [34, 35]. This rate has not been objectively or prospectively studied and can vary greatly. Nature of the injury, location, wound bed, age of the patient, and technique used for repair or reconstruction have all been demonstrated to affect velocity or nerve regeneration [36, 37]. This process does not begin until several weeks after nerve injury, when inflammatory and axonal regenerative processes occur to result in endoneural tube formation that allows neurite growth cone progression. This process may take 3–6 weeks to occur.
Clinical evaluation of nerve regeneration begins with thorough history , physical examination, and evaluation of all studies already performed to understand or diagnose the nerve injury in question and interpret the findings within the context of and the time course from injury. In general, motor nerve fibers must reinnervate motor end plates within 12–18 months before an irreversible loss of the motor end plate occurs preventing further muscle fiber reinnervation [26, 38]. Sensory reinnervation was long though to not involve a time-dependent mechanism, but has now been recognized to have diminishing ability for sensory mechanoreceptor reinnervation after approximately 5 years. Other factors that help establish the “context” of the injury include the suitability of the wound bed, history of radiation , infection, the suitability of distal reinnervation targets, patient age, comorbidities, and the mechanism of injury to the nerve.
Analysis of the ongoing regeneration process typically consists of observation or return of function with application of the measures listed in Table 19.1. In addition, utilization of the advancing “Tinel’s” sign has long been considered a standard measure of nerve regeneration progression [39–41]. The “Tinel” sign is simply tapping over the regenerating nerve and the point at which maximal paresthesias can be elicited into the distribution of the nerve being tested is considered that length to which axons have regenerated (Fig. 19.5). However, focal pain is frequently misinterpreted for paresthesias, and many patients exhibit paresthesias over a long distance, not just a focal location which can lead to an erroneous misinterpretation of regeneration.
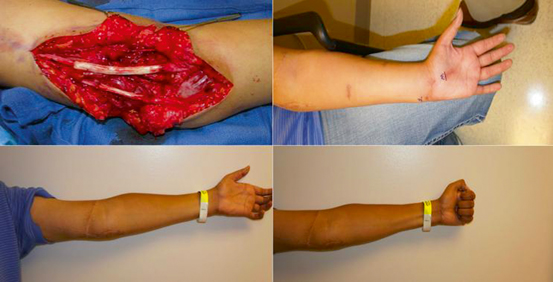
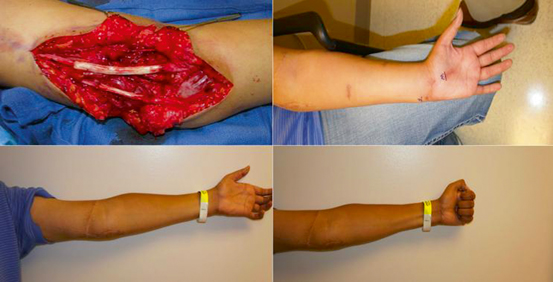
Fig. 19.5
Advancing Tinel’s sign of regenerating axons 12 months after a nerve graft repair of a proximal ulnar and median nerve injury. (Above, Left) Repair of proximal median and ulnar nerve with nerve allografts after resection of neuroma-in-continuity approximately 12 months after a traumatic laceration. (Above, Right) Advancing Tinel’s sign marked in proximal palm demonstrating reinnervation of median and ulnar nerves. (Below) Functional return of median and ulnar nerves demonstrating ability to form composite fist
Sensory recovery measurement can be superficially tested by simply ascertaining the presence of sensation to a light touch or sharp, painful stimulation. However, more standardized or objective measurements serve to give more meaningful and reproducible data. Objective sensory testing can evaluate sensory threshold or sensory density. Threshold testing typically utilizes measures such as the Semmes–Weinstein monofilaments which measure the threshold of perceptible pressure exerted against the skin of an innervated area. Standard limits for what is considered normal perception of light touch, diminished normal perception but protective sensation, diminished protective sensation, loss of protective sensation, and presence/absence of deep-pressure perception have already been established [42].
Sensory density discrimination can be performed with static and moving two-point discrimination. Two-point discrimination is the measurement of the minimal distance at which two points can be discriminated as separate sites simultaneously. This can be done gently pushing the measurement device (such as calipers) onto the skin until the skin blanches (static two-point discrimination, S2PD) or by moving the calipers of the skin surface at a set distance (moving two-point discrimination M2PD). The British Medical Research Council (MRC) has further defined overall “functional” grades of sensory recovery that have been since modified by Mackinnon and Dellon [43–45] (Table 19.2).
Table 19.2
British medical research council grades of functional sensory recovery
Grade | Sensation present |
---|---|
S0 | Absence of sensibility in the isolated nerve region of sensation |
S1 | Recovery of deep cutaneous pain and tactile sensibility |
S1 + | Recovery of superficial pain sensibility |
S2 | Recovery of some degree of superficial cutaneous pain and tactile sensibility |
S2 + | Recovery of some degree of superficial cutaneous pain and tactile sensibility, but with over response |