Chapter 38 Electrical injuries
Access the complete reference list online at http://www.expertconsult.com
Electrical burns
Introduction
Electricity is an indispensable part of civilization, invisible and often taken for granted. Electrical burns typically comprise only a small percentage of total admissions to major burn centers – reportedly about 5–7%.1–3 They are, however, the most devastating of all thermal injuries on a size-for-size basis, usually involving both the skin and deeper tissues. They primarily affect young, working men, often have legal involvement, and are the most frequent cause of amputations on the Burn Service. In addition to power company linemen, electricians, construction workers, laborers, and crane operators are at special risk.4 These devastating injuries account for nearly 6% of all occupational fatalities annually.5
Pathophysiology
Electrical burn severity is determined by voltage, current (amperage), type of current (alternating or direct), path of current flow, duration of contact, resistance at the point of contact and individual susceptibility. Electrical burns are arbitrarily classified as low-voltage (<1000 V) and high-voltage injuries (1000 V and higher). Whereas low-voltage burns are generally localized to the area immediately surrounding the injury, the high-voltage burn is associated with deep extension and underlying tissue damage, very closely resembling a crush injury.6 Domestic wiring in the United States operates on alternating current (AC) at 120 V. Therefore, nearly all burns occurring indoors, except in specialized industrial settings, are of the low-voltage type. Although the victim or witness(es) often knows the voltage involved, the amount of current is unknown, with current flow related to voltage by Ohm’s Law where:
Animal experiments have demonstrated that resistance varies continuously with time, initially dropping slowly, then much more rapidly until arcing occurs at the contact sites. Resistance then rises to infinity and current flow ceases.7 Temperature measurements taken simultaneously showed that the rate of temperature rise parallels changes in amperage. Interestingly, tissue temperature, the critical factor in the magnitude of tissue damage, does not increase distal to the contact points.
In North America, more than 99% of all electrical burns are caused by 60 cycle-per-second commercial alternating current, which reverses its polarity 120 times per second. Only an occasional low- or high-frequency industrial injury is encountered. With half of the time spent positive with respect to ground and one half spent negative, the terms ‘entrance’ and ‘exit’ wounds are archaic, as one does not know whether a given point on the body contacted the wire or the ground. These terms have now been replaced by ‘contact points.’ One or more contact points may be clearly visible or at times pinpoint, subtle, or hidden, for example beneath the hairline (Fig. 38.1). A descriptive term such as blow-out type injury describes concentration of current and not its causation.
The path of current makes a significant difference in prehospital fatalities, but in patients reaching the hospital alive, current path determinations are often less than precise and meaningful. The patient may have no, one or many visible contact points and an untold number of invisible contacts. An example of this is surgical electrocautery, where the large grounding pad contact site is not visible (hopefully). Despite some misconceptions, the term ‘electrocution’ does not apply to these living patients, as electrocution is defined as ‘to kill by electric shock.’8
Alternating current causes tetanic muscle contractions, which may either throw victims away from the contact or draw them into continued contact with the electrical source – the ‘no-let-go’ phenomenon, creating the potential for continually increasing severity. This phenomenon occurs because both flexors and extensors of the forearm are stimulated by current flow. However, the muscles of flexion are stronger, making the person unable to let go voluntarily. Altered levels of consciousness, reported in about half of high-voltage victims, also contribute to prolonged periods of contact.9 More than 99% of the body’s resistance to electric current flow is at the skin. Resistance is measured in ohms (Ω). Resistance at the point of contact varies from very low values for sweat-soaked hands or skin in the summer to more than 100 000 Ω for heavily calloused hands or feet during very dry winter weather. Individual susceptibility is a non-quantifiable term to explain why two or more individuals exposed to the same situation have extremely varied injuries.
The burn injury has the potential for three different components: the true electrical injury caused by current flow, an arc injury resulting from the electrical arc generated as the current passes from the source to an object, and a flame injury caused by ignition of clothing and/or surroundings. Electricity arcs at temperatures of up to 4000°C create a flash type injury,10 most often seen in electricians working with metal objects in close proximity to an electrical source. The victim may be thrown by the force, and suffer ruptured eardrums and contused internal organs. When electrical injuries occur without actual current flow through the victim, they are treated and classified in the same manner as any flash burn.
Tissue resistance is from lowest to highest in nerves, blood vessels, muscle, skin, tendon, fat and bone, respectively. Theoretically, current flow would be distributed in proportion to resistance, with tissues having the highest resistance generating the most heat. However, in animal models the body acts as a single uniform resistance rather than a collection of different resistances, i.e., it behaves as a volume conductor.7 Severity of injury is inversely proportional to the cross-sectional area of the body part involved. Thus the most severe injuries are often seen at the wrist and ankle, with severity decreasing proximally. Deep tissues appear to retain heat so that the periosseous tissues, especially between two bones (i.e. tibia–fibula, radius–ulna) often sustain a more severe injury than superficial tissue. The associated macro- and microscopic vascular injury appears to occur nearly immediately and is not reversible.11 A recent study employing a rabbit animal model and optical microscopy demonstrated severe injury to blood vessels. Necrosis of vascular walls and thrombosis with destruction of arterial endothelium, pyknosis of vascular smooth muscle and fibrinous exudates accompanying the thrombotic changes was noted. This study also showed progressive muscle necrosis over the first 72 hours after injury that the investigators surmised may have been related to the vascular injury.12 Of note, there was also pathologic evidence of injury to organs remote from the contact points (heart, lung, liver and kidney).
Direct and indirect electrical destruction of cells also plays a role in tissue injury. The major mechanisms of injury include Joule heating, electroporation, and electroconformational denaturation of transmembrane proteins.13,14 In all of these mechanisms damage is caused by disruption or modulation of cell membranes. Joule heating can be thought of as the frying of tissue. This is the transfer of energy from charged particles bumping up against tissue molecules and losing energy to the tissue in the form of heat. Temperature causes denaturation of macromolecules, which is usually irreversible. Electroporation is the formation of aqueous pores in lipid bilayers exposed to a supraphysiologic electrical field. The formation of these pores allows calcium influx into the cytoplasm and triggers a subsequent cascade leading to apoptosis. Electroconformational denaturation of transmembrane proteins refers to the changes in polarity of amino acids in response to exposure to electrical fields. Experimentally, voltage-gated channel proteins were found to change their conductance and ion specificity after exposure to a powerful pulsed field.15
Acute care
Electrocardiographic monitoring
All studies reviewed in a recent American Burn Association Guidelines paper confirmed that cardiac abnormalities, including dysrhythmias and myocardial damage, occur after both low-voltage and high-voltage injuries, thus reinforcing the need for ECG evaluation as part of the initial evaluation in all patients.16 Non-specific ST-T changes are the most common ECG abnormality and atrial fibrillation is the most common dysrhythmia.17,18 This has also been the authors’ experience, and all dysrhythmias have responded to appropriate medical management as per ACLS protocols.
Direct myocardial injury may also result. This injury behaves more like a traumatic myocardial contusion than a true myocardial infarction, not having the hemodynamic or recurrence consequences of atherosclerotic myocardial infarctions. Housinger et al. have shown that creatine kinase (CK) and MB-creatine kinase (MB-CK) levels are poor indicators of myocardial injury in the absence of ECG findings of myocardial damage, especially in the presence of significant skeletal muscle injury.19–21 Myocardial damage and dysrhythmias are manifested very soon after injury.22 Although cardiac troponins are the preferred biomarkers for detecting myocardial injury in most clinical settings, this has not been adequately studied in the setting of electrical injuries. A recent study using serum troponin levels and serial echocardiography in 20 patients surviving high-voltage injury concluded that this was not a useful diagnostic test for predicting impaired left ventricular contractility.23 The interpretation of cardiac biomarkers is problematic and needs to be correlated with other clinical findings. All patients should be monitored during transport and in the emergency room. Rather than a policy of more prolonged cardiac monitoring for all patients, a selective policy makes most efficient use of expensive medical resources, without patient risk.4,22
No published studies have directly studied the appropriate duration of telemetry monitoring, but most series indicate 24–48 hours.24–27 Our institutional bias is to monitor for approximately 24 hours post injury in those patients with an indication. Low-voltage injuries not meeting the criteria for cardiac monitoring and no other indication for admission can be safely discharged from the emergency room. This is not applicable to high-voltage injuries, although there is retrospective evidence that dysrhythmias will occur early if at all in these patients.22
Traumatic injuries
Approximately 15% of electrical burn victims sustain traumatic injuries in addition to their burn, a rate nearly double that of other burn patients. Most of these injuries are caused by a fall from a height or being thrown against an object, but some resulting from the tetanic muscle contractions associated with the electrical shock itself, forces strong enough to cause compression fractures.28 A careful history and physical examination should select those patients who require a full trauma evaluation.
Compartment syndrome
Patients with high-voltage electrical injuries of the extremities are at risk for the development of compartment syndromes during the first 48 hours post injury. Damaged muscle, and swelling in the investing fascia of the extremity, may increase pressures to the point where muscle blood flow is compromised. Loss of pulses is one of the last signs of a compartment syndrome, unlike the early loss of pulses occurring in a circumferentially burned extremity requiring escharotomy. A high index of suspicion is paramount for an early diagnosis, usually by serial examinations. Compartment pressure measurement is generally not necessary and may even be misleading (Figs 38.2 and 38.3). Whereas a very aggressive approach to fasciotomy has been advocated in the past, significant morbidity attends a fasciotomy and its closure. Amputation rates were generally reported to be in the 35–40% range in most of these early series.29–33
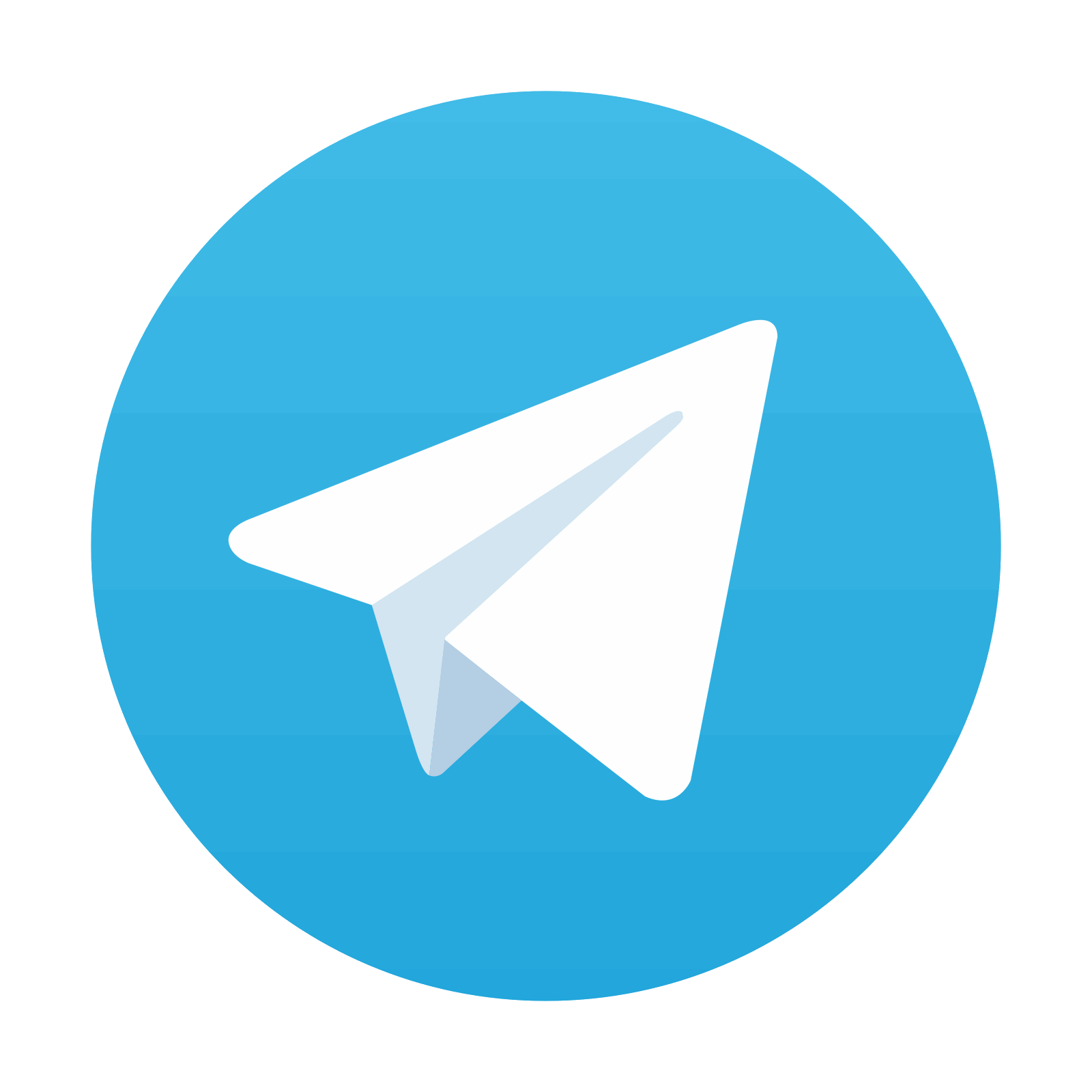
Stay updated, free articles. Join our Telegram channel

Full access? Get Clinical Tree
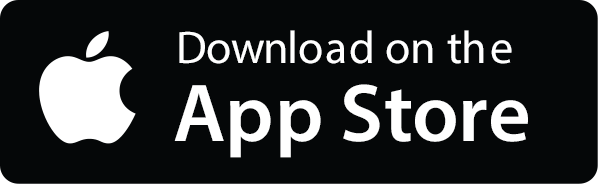
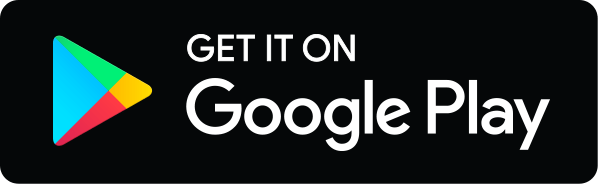