Dermal and Soft-Tissue Fillers: Principles, Materials, and Techniques
Z. Paul Lorenc
INTRODUCTION
As Baby Boomer and Gen X populations transition to mid- and later life, numerous nonsurgical cosmetic procedures and materials have been developed for this demographic. The American Society for Aesthetic Plastic Surgery reported a 155% increase in cosmetic procedures from 1997 to 2010. Nine and a half million of these procedures were performed in the United States. Second only to injections of neuromodulators (Chapter 43), such as OnabotulinumtoxinA (Botox), AbobotulinumtoxinA (Dysport), and IncobotulinumtoxinA (Xeomin), the use of dermal filler/volumizing agents topped the list for nonsurgical rejuvenation.1
Dermal fillers have been used for decades. Early applications involved autologous fat grafting with only short-term benefits.2 The popularity (and profit) of bovine collagen as a filler material in the 1980s generated an explosion in the development of injectable soft-tissue fillers, with varying properties, longevity, and ease of use that have significantly altered the landscape of minimally invasive cosmetic correction.2
In the past decade alone, the vast array of dermal fillers/volumizing agents on the market, coupled with their efficacy, versatility, biocompatibility, resorbability, cost-effectiveness, and relative ease of administration, has created both opportunities and challenges for patients and practitioners. Involving little downtime for the patient, minimal adverse events, and generally less than an hour to administer in a practitioner’s office, injectable agents help restore youthful vitality by effacement of wrinkles, repleting volume, and recontouring in the face, hands, and less visible parts of the body.3
The field of facial rejuvenation has evolved along with the growth in noninvasive applications. Once focused on filling wrinkles, lines, and depressions, practitioners now attempt to recontour by volume replacement. The face does not age uniformly, and corrective modalities and materials are selected based on the relationship of the whole to its parts.4,5 The ideal filler/volumizing agent would be safe, biocompatible, noninflammatory, nonallergenic, stable, easily deliverable, pliable, nonmigratory, and durable.6,7 The clinical performance of any agent depends upon its physiochemical characteristics and interaction with the recipient.
PHYSIOCHEMICAL CONSIDERATIONS
The in vivo and in vitro characteristics of soft-tissue fillers are outlined in Table 42.1. An understanding of physiochemical characteristics enables the practitioner to assess their deliverability, performance, immunogenicity, applications, aesthetic effectiveness, and longevity.2,8
The ultimate behavior of soft-tissue fillers is a function of a number of scientific variables. These include biocompatibility/biofilm formation, viscosity, gel/fluid ratio, Hyaluronic Acid (HA) concentration, percentage of cross-linking with degree of pendant cross-linker, particulate versus liquid versus fibrillar constituency, particle size, elastic modulus (G′), complex viscosity (η*), and extrusion characteristics. Especially critical to the mechanism of action are lifting ability/elasticity and viscosity. Recent assessments of HA-based and calcium hydroxylapatite (CaHA) soft-tissue fillers have explored the relationship of their physiochemical properties to performance on rheologic tests, which measure the flow of fluids and physical changes of solids under applied stresses and strains.9,10,11
A soft-tissue filler’s utility is determined in part by its viscosity (η*), elasticity (G′), and plasticity, all of which constitute a product’s rheology.9,11 Rheologic testing replicates the movement and action of gravity on facial tissues, by applying shearing force to popular HAs and CaHA.9,10,11 In one study, investigators found that, largely due to the products’ high G′ value, CaHA (Radiesse, Merz Aesthetics, San Mateo, CA) retained structural integrity, viscosity, and elasticity.10,11 In clinical applications, injecting stiffer (high G′, more lifting capacity) products into the skin could prove more challenging for the practitioner. Agents with low G′ may be more effective for filling superficial wrinkles, areas around the lips, and lips themselves, while gels resistant to dynamic force (high G′) may be more suitable for deeper fold correction, to volumize the malar eminence, augment the mentum, or volumize the temporal hollow9 (Figure 42.1).
Molecular cross-linking confers strength and structural integrity to HA-based gels, allowing the filler to retain its shape until it is degraded and metabolized by the body.9 Cross-linking entails a delicate balance to simultaneously provide effective biomechanical properties while maintaining biocompatibility and tissue interactivity.9 The amount and the degree of cross-linking—not the HA concentration alone—determines filler duration.12,19 When gels undergo similar modifications with like concentration, the higher cross-link to pendant (partially bound cross-linker) ratio will assure a less degradable, more stable, and longer lasting effect.9
TABLE 42.1 PHYSIOCHEMICAL CHARACTERISTICS OF FILLERS/VOLUMIZING AGENTS | |||||||||||||||
---|---|---|---|---|---|---|---|---|---|---|---|---|---|---|---|
|
Particle size, shape, and distribution (G*) have an influence on extrudability and potential bruising. If the particles are dispersed too loosely or closely and still remain large, they may prevent a smooth flow of filler and result in subsequent bruising from sporadic delivery. These inherent physiochemical characteristics affect the forces necessary to inject an agent.8 In general, higher G′ helps assure stability for optimum sculpting/lifting, while higher η* enables materials to withstand applied forces once injected.11
Particle size is another consideration. An evaluation of the impact of particle size on phagocytosis was performed. Polymethylmethacrylate (PMMA) microspheres of <20 μm in diameter promoted phagocytosis, potentially inducing adverse skin reactions and rendering the particles nonviable.7 In another study, PMMA particles of different sizes demonstrated that larger sized microspheres >40.2 μm were not phagocytized.7,13 In addition to particle size, inflammatory response is affected by particle shape, contact angles, collision factors, surface tension, and surface charge. In one study, an in vivo implantation of irregularly shaped polymer implants initiated host inflammatory response in contrast to smoothsurfaced PMMA microspheres.7 For now, particle size >20 μm appears less likely to induce an inflammatory response than smaller sized microspheres.
CLASSIFICATION OF AGENTS BY FUNCTION
In spring 2011, a panel of physicians recommended categorization of injectable fillers by their function and clinical outcomes.14 The categories include neuromodulators, collagen-based agents, replacement agents, biostimulatory agents, long-lasting agents, and autologous fat (Table 42.2). (Neuromodulators are covered in Chapter 43.)
Collagen
Derived from bovine, porcine, and live or cadaveric human dermal tissue, collagen provides support and strength to the skin. Skin testing is required for agents containing bovine collagen.
Cymetra (LifeCell Corporation, Branchburg, NJ), an aseptic particulate, injectable form of AlloDerm prepared for delivery in 0.5% lidocaine with 1:200,000 epinephrine, is derived from isogenic human tissue. Indicated for augmenting lip volume, nasolabial fold injection (NLF) correction, and burn and scar treatment, Cymetra is obtained from tissue banks or cadavers, which have been tested and screened for immunologically threatening risk factors.15
TABLE 42.2 FDA CLEARED FILLER/VOLUMIZING AGENTS (ITEMS IN PARENTHESES ARE NO LONGER AVAILABLE) | ||||||||||||||||||||||||||||||||||||||||||||||||||||||||||||
---|---|---|---|---|---|---|---|---|---|---|---|---|---|---|---|---|---|---|---|---|---|---|---|---|---|---|---|---|---|---|---|---|---|---|---|---|---|---|---|---|---|---|---|---|---|---|---|---|---|---|---|---|---|---|---|---|---|---|---|---|
|
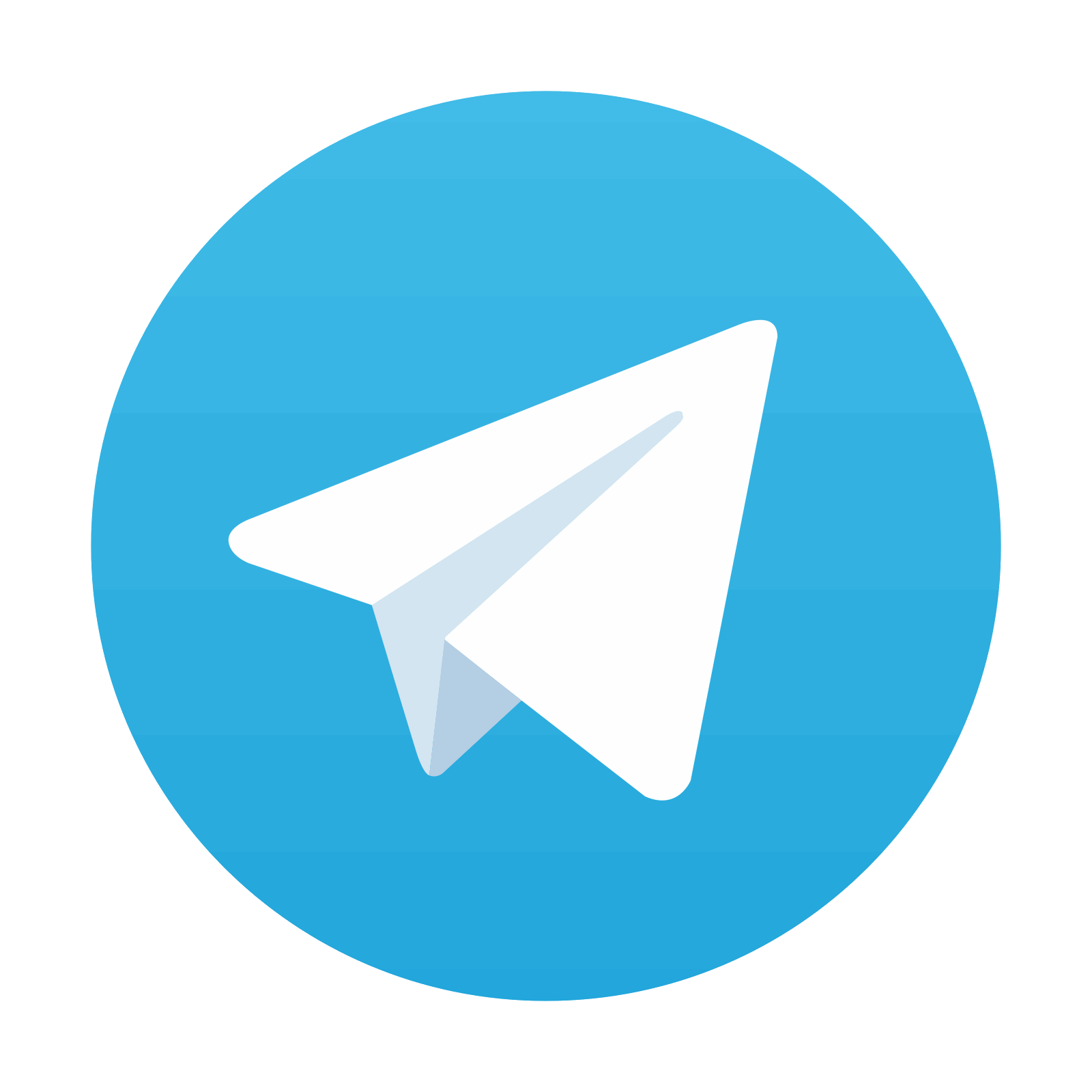
Stay updated, free articles. Join our Telegram channel

Full access? Get Clinical Tree
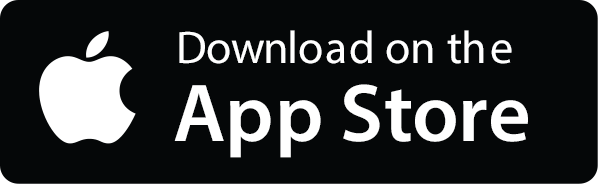
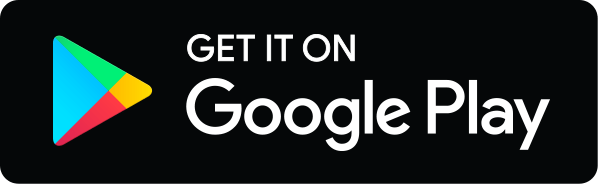