Chapter 16 IMPROVING SKIN QUALITY: AN EXPERIMENTAL AND CLINICAL STUDY OF THE EFFECTS OF FAT AND STROMAL CELLS ON THE SKIN
Adipose tissue is the most abundant source of stem cells in the human organism. 1 When transfered to a recipient site, adipose tissue interacts with surrounding tissue through the secretion of cytokines by the adipocytes and the actions of the stem cells and various growth factors present. Thus adipose tissue acts as an active filler. 2 – 4 The endothelial growth factor found in adipose tissue can improve ischemia. 5 Some authors have even used adipose tissue transfer technique for treatment of poorly vascularized radiodystrophic lesions 3 and chronic ulcer lesions. 6
In this chapter we will present our work on nude mice demonstrating histologic and immunohistologic analysis of skin changes at the recipient site after human adipose tissue transfer 7 and the skin regenerative potential of stromal cells in a wound-healing model. 8 We will also provide clinical cases of radiotherapy-induced skin atrophy or aesthetic surgery demonstrating improved skin quality in the treated areas. 8 , 9
Animal Study Demonstrating the Improvement of Skin Quality
To date, the effects of adipose tissue on the skin have only been demonstrated in a clinical setting. 2 In an attempt to further our understanding of the mechanisms of action underlying this technique, we performed an experimental study. Our aim was to use histologic and immunohistologic analyses to compare skin before and after fat grafting. 6 To simulate clinical conditions, adipose tissue was harvested, purified, and reinjected according to the protocol described by Coleman. 10 – 12 Nude immunodeficient mice were chosen for this study for their immunologic tolerance of human cells. Histologic and immunohistologic analyses were used to investigate the structure of the transplanted adipose tissue, the different subcutaneous layers, and the space between the adipose tissue and the skin.
Ten male nude mice aged 42 days were used for this study. Thirty cubic centimeters of adipose tissue were harvested from the abdominal region of a 36-year-old patient-volunteer using a 3 mm diameter Coleman harvesting cannula attached to a 10 ml Luer-Lok syringe. The adipose tissue was then treated according to the protocol described by Coleman 10 – 12 and sent directly to the animal laboratory for the experiment in mice. One milliliter of purified adipose tissue was then injected using a 1 mm diameter blunt-tipped cannula into the left parascapular region of each mouse. Eight weeks after implantation, a skin biopsy, including subcutaneous tissue, was performed in the injected area. A control biopsy in the same region was performed on the opposite noninjected side.

The regions of biopsy are shown, on the left side at the site of injection, and on the right in the control region. Note the visible growth only on the left side.
HISTOLOGIC AND IMMUNOHISTOLOGIC ANALYSES
Hematoxylin-phloxin-saffron (HPS) staining was performed on 5 µm deparaffinated slices. The hematoxylin is a basic dye that stains the nucleus of the cells blue. The phloxin is an acid dye staining the cytoplasm pink, and the saffron stains the connective tissue orange.
The samples were also embedded in Tissue Teck; 7 µm slices were next fixed in isopropanol and then stained with a solution of Oil Red O (Sigma), an adipocyte-specific dye which stains the lipid-filled vacuoles red.
Slices 5 µm thick were deparaffinated and then incubated with anti-mouse collagen I antibodies. We used anti-mouse IgG antibodies coupled to peroxidase to detect the immunocomplexes, using diaminobenzidine as the substrate. Counterstaining was performed using Harris hematoxylin. This labeling enabled us to detect cells of mouse origin. Human skin was used as a negative control.
Other slices were incubated with anti-human collagen I specific primary antibodies, followed by a secondary antibody coupled to a fluorochrome to reveal the binding sites of the primary antibody. These could thereby be observed under a fluorescent microscope to visualize collagen fibers of human origin.
HISTOLOGIC AND IMMUNOHISTOLOGIC RESULTS
Eight weeks after implantation, all 10 mice had a left parascapular growth corresponding to the site of transplantation of the adipose tissue. Histologic analysis demonstrated the presence of mature adipocytes stained by Oil Red O. The HPS staining revealed a considerable increase in density of the extracellular matrix surrounding the adipose tissue and between it and the dermis. The dermis also showed an increased thickness after injection of adipose tissue. This increase in density of the extracellular matrix was made up of collagen I fibers, an absence of which could be noted on the control noninjected side.

Histologic slices of control skin (A) and skin with grafted adipose tissue (B) are shown. Note the thickening of the dermis and the abundance of extracellular matrix between skin and adipose tissue, as well as that surrounding the fat lobules after the transfer of adipose tissue. Immunohistochemistry, using an anti-mouse collagen I antibody to label in brown the extracellular matrix, shows that the mass of collagen fibers are of murine origin (C and D). The negative control (human skin) shows an absence of labeling at this level (E). Adipocyte viability is confirmed by Oil Red O staining (F).
Immunohistochemical analysis using a labeling technique with anti-mouse collagen I allowed us to demonstrate the origin of the newly formed cells. The neosynthesized extracellular matrix was found to be of murine origin and not human, confirmed by the human skin negative control. Concordantly, immunofluorescence using an anti-human collagen I antibody confirmed the murine origin of collagen fibers, since no labeling was found in the mice.

This immunofluorescent slice of mouse skin following fat tissue grafting (A) shows the absence of human collagen fibers after labeling with an anti-human collagen I–specific antibody. In contrast, the human skin (B) shows a considerable density of human collagen fibers, stained green.
CONCLUSIONS
The results of our experimental study in mice agree with clinical observations made in patients treated by the same technique. Adipose tissue grafts survive and have a volume-increasing effect. Moreover, the presence of human adipose tissue increases murine dermis thickness at the recipient site by induction of collagen neosynthesis, likely as a result of the introduction of stem cells, growth factors, and cytokines. This collagen, mostly type I but also types V and VI, is of murine origin. Our study also demonstrated that adipose tissue can stimulate production of collagen by the host cells at the recipient site. This is supported by other studies showing that ADSCs present in adipose tissue promote proliferation and migration of fibroblasts and activation of extracellular matrix synthesis. 13 – 16 However, our study did not allow us to determine whether this induction is directly caused by the adipose tissue or is the result of an indirect passage through an intermediary inflammatory reaction. The presence of the adipose tissue graft causes an increase in local vascularization, resulting in a new physiologic equilibrium at the recipient site. Together, these observations demonstrate active filling properties of adipose tissue. Further studies are needed to show the long-term effects of fat grafting at the recipient site.
Animal Study Demonstrating the Wound-Healing Properties of Adipose-Derived Stem Cells
Cutaneous healing can be impaired by many situations such as diabetes, chronic renal failure, irradiation and advancing age. 17 Because of our aging population, today chronic wounds constitute a real public health problem. The main goals of treatment are rapid closure of the wound to restore the skin’s barrier function and prevent infection, suppression of pain, and recovery of function. During the past decade, many reconstructive therapies based on the use of stem cells have been introduced. These are undifferentiated cells with self-renewing properties and are able to divide to generate specialized cells, including skin cells, fibroblasts, and endothelial cells. 18 The stromal vascular fraction (SVF) of adipose tissue contains ADSCs, and its efficacy has been shown in experimental healing models. The suggested mechanism of action is the modulation of inflammation and tissue regeneration by the differentiation of stem cells. 19 However, SVF is a heterogeneous cell suspension containing a small percentage (1% to 3%) of ADSCs. 20 Through cell processing, it is now possible to obtain a homogeneous cell therapy product containing more than 90% ADSCs. 21 The possibility of freezing ADSCs offers (1) the potential to perform all safety checks before releasing the product and (2) the ability to repeat the treatment if necessary.
We performed a study to evaluate the effectiveness of a controlled dose of injected cultured fresh or frozen ADSCs compared with a stromal vascular fraction (SVF) on wound healing in an animal model. To avoid their dispersion in the subcutaneous tissue, ADSCs were injected in a noncrosslinked hyaluronic acid gel (Cytocare 532).
METHOD
Human SVF was isolated from lipoaspirate obtained from a randomly chosen donor who was undergoing liposuction. Adipose tissue was digested with collagenase and the reaction stopped by adding Dulbecco’s Modified Eagle’s Medium (DMEM). Floating adipocytes were discarded, and cells from the stromal vascular fraction (SVF) were pelleted, rinsed, centrifuged, and counted before being mixed with noncrosslinked hyaluronic acid, Cytocare 532 (Cyt 532), for injection into nude mice. Cyt 532 is a noncrosslinked hyaluronic acid and a rejuvenating complex containing 50 components. It was used as a vehicle in our study because previous published work 22 showed that it was the best culture medium for ADSCs. Crosslinked hyaluronic acid prevents stem cells from multiplying and differentiating.
Freshly isolated cell suspensions containing ADSCs were cultured in ADSC medium until 80% confluence was reached. At confluence, cells were trypsinized and centrifuged. A portion of the ADSCs was obtained using the technique described earlier and these were frozen. The cells were thawed 1 week before surgery and cultured in ADSC medium.

Sixteen male nude 42-day-old mice were anesthetized, and surgery was performed under standard sterile conditions. Two circular full-thickness wounds 12 mm in diameter were created on the backs of the mice and a ring of silicone was sutured around the wound to prevent skin retraction. Each healing wound received 1.10 6 ADSCs, or SVF with half the cells intradermally injected around the wounds into four injection sites, and the other half applied to the wound surface. Each nude mouse received a different treatment for each wound. Cyt 532 was used as vehicle for the cells.
Two sets of experiments were designed, comprising 32 wounds on 16 mice (two wounds per mouse). Five excisional wounds were carried out in each group as follows:
Negative control (spontaneous healing)
Vehicle alone (Cyt 532)
Cultured frozen ADSCs (c.fr.ADSC + Cyt 532) cryopreserved at passage 1, then thawed
SVF (SVF + Cyt 532)
Cultured ADSCs (passage 2) (c.ADSC + Cyt 532) without cryopreservation before injection
Every day the mice were observed and digital images taken. The wound area was measured by tracing the wound margin and calculating the pixel area using image analysis software. The percentage of wound healing was calculated using reepithelialization surface measurements.
At day 27, all scar tissue was harvested with a rim of healthy normal skin for histologic analyses. Tissue samples were fixed, embedded in paraffin, and stained. Inflammation was evaluated on a semiquantitative scale. Sections were examined in a blinded fashion by two independent observers. Perfusion evaluation was done by means of laser Doppler imaging on day 27, when all wounds had closed and biomechanical tests were conducted to evaluate the properties of the scarring mouse tissue.
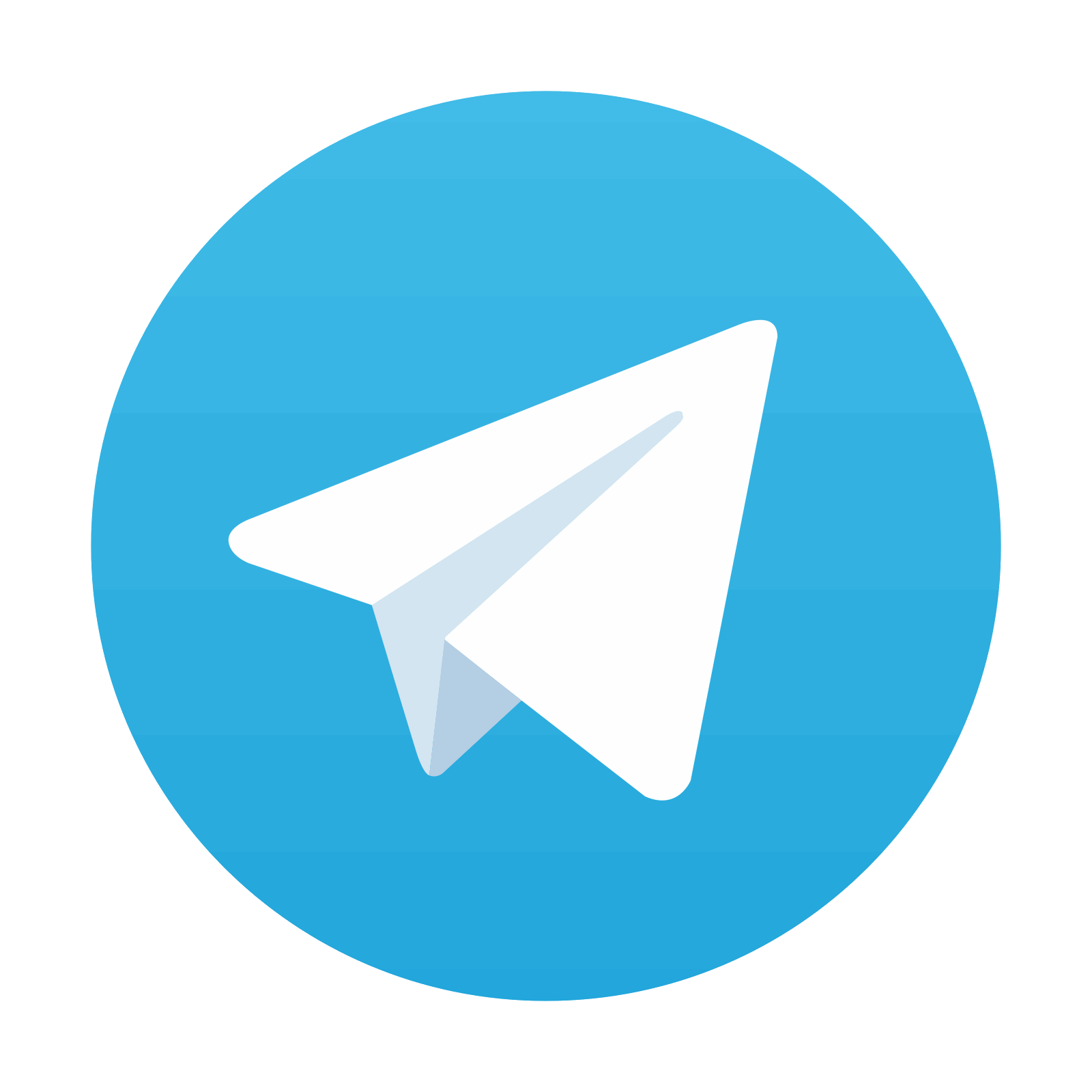
Stay updated, free articles. Join our Telegram channel

Full access? Get Clinical Tree
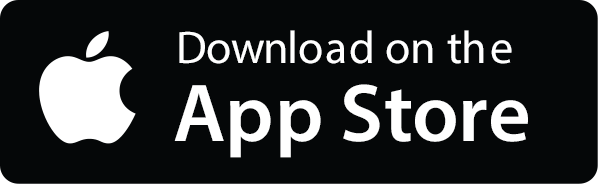
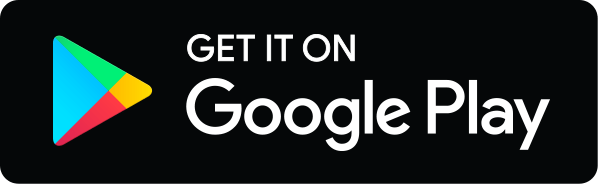
