and Maria Z. Siemionow2
(1)
Department of Clinical Immunology, L. Hirszfeld Institute of Immunology and Experimental Therapy Polish Academy of Sciences, Wroclaw, Poland
(2)
Department of Orthopaedics, University of Illinois at Chicago, Chicago, IL, USA
Abstract
Experimental studies on tolerance inducting strategies in vascularized composite allograft (VCA) are widely performed to make them clinically applicable. The idea of hematopoietic chimerism, as a mechanism for tolerance induction, is extensively studied in transplantation procedures. This review summarizes current knowledge on tolerance inducing strategies via donor bone marrow transplantation, T-regulatory cells and mesenchymal stem cells (MSC) application in VCA transplants. Pioneering experimental and clinical studies on transplantation tolerance induction via cellular therapies in solid organ transplantations are encouraging and indicate that similar protocols will be relevant for VCA transplants. Application of donor hematopoietic cells, MSCs or to employ biologic ability of immuno-regulatory cells to inhibit effector cells action may be promising tool for tolerance induction in VCA.
Keywords
Vascularized composite allograft (VCA)Cellular therapies, donor bone marrow transplantationT-regulatory cellsMesenchymal stem cellsChimerismTransplantation toleranceAbbreviations
ALS
Antilymphocyte serum
APC
Antigen presenting cells
BMC
Bone marrow cells
BMT
Bone marrow transplantation
CsA
Cyclosporine A
GvHD
Graft-versus-host disease
MHC
Major histocompatibility complex
MLR
Mixed lymphocyte reaction
MMF
Mycophenolate mofetil
MSC
Mesenchymal stem cells
PBMC
Peripheral blood mononuclear cells
TBI
Total body irradiation
Treg
Regulatory T-cells
VCA
Vascularized composite allograft
αβ-TCR
Alpha/beta T-cell receptor
Introduction
More than 60 years have passed since Billingham, Bernt, and Medawar first demonstrated acquired immunological tolerance to allogeneic skin in an experimental mouse model by intravenous delivery of donor hematopoietic cells into neonatal mice [1]. In this model, the absence of immune activity was achieved due to clonal deletion and was maintained by the persistence of lymphoid chimerism. Since that time, different strategies for tolerance induction via donor bone marrow transplantation (BMT) have been developed in clinical and experimental studies [2–8].
Immunologic tolerance is currently on focus in transplantation procedures including cellular, solid organs and vascularized composite allograft (VCA) transplantations. Pharmacologic agents effectively inhibit immune response after allotransplantation, however, long-term immunosupression may be a risk of side effect. Biologic intervention with donor-origin cells for chimerism induction and short-term pharmacologic action on allograft survival is extensively studied as a promising tool for tolerance induction in transplantation procedures [9].
Chimerism is the concept that different genetically cells coexist in the same organism and induces transplant tolerance while maintaining immunocompetence. Chimerism is defined in three categories: (1) complete chimerism when recipient hematopoietic cells are totally eliminated by myeloablation and 100 % of bone marrow cells (BMC) is replaced by donor-origin cells; (2) mixed chimerism when both donor and recipient cells coexist in the bone marrow compartment of recipients, and donor-origin cells are detectable from 1 to 99 %; and (3) microchimerism which usually occurs spontaneously when donor-origin cells engraft into different lymphoid and non-lymphoid tissues of recipient but their number is less than 1 % and donor cells are detectable by sensitive methods as immunocytochemistry or PCR [10, 11].
The idea of hematopoietic chimerism, as a mechanism for tolerance induction in transplant allograft recipients, is extensively studied in experimental models and recently in clinical practice. However, practical implementation of this strategy in the clinic still requires overcoming the number of barriers before clinical application of chimerism could be successful. The most significant barrier is the conditioning of donors and recipients to generate an environment where both donor and host hematopoietic cells can co-exist [12].
Hematopoietic Cells as an Integral Part of VCA
Early studies on chimerism phenomenon documented that migration and localization of donor passenger leukocytes in heterogenous locations of the recipient are essential for graft acceptance in solid organ transplant recipients [13, 14]. VCA are histologically heterogenous and composed of different tissue types including skin, muscle, bone with BMC, lymph nodes, nerve and vessels, which constitutively contains a different type of passenger leukocytes including cells of hematopoietic origin [15]. When comparing the chimerism level of different VCA models, VCA tissue complexity and its immunogenicity should be considered [16]. Skin is a major component of limb, face and abdominal wall transplants and serves as an abundant source of donor immunocompetent cells which migrate into recipient periphery. The face and neck region in rats is very rich in lymph nodes, and we suggest that the presence of lymph nodes within facial VCA contributes to a high chimerism level in the peripheral blood and lymphoid organs of recipients [17, 18]. Moreover, mucosal tissue, combined with salivary glands, in face transplant model, is also a rich supply of donor-origin cells represented by T cells, B cells, and NK cells, which are distinct from the cells present in peripheral lymphoid organs and, after transplantation, may support chimerism induction and maintenance [18, 19].
The presence of vascularized bone containing hematopoietic cells within VCA represents one of the forms of cellular therapy in VCA. Bone is a key component of limb transplant and contains multi-lineage hematopoietic cells such as myeloid, lymphoid and erythroid at various stages of differentiation and maturation which are capable for lympho-myeloid reconstitution in the recipient body. Experimental limb and face transplant (mandible or calvaria) models carrying bone component containing BMC are examples of vascularized BMT and actively participate in chimerism induction in allogeneic transplantation in rats [2, 3, 18–21].
The potential for VCAs containing a vascularized bone segment has raised attention in the immunologic role of vascularized BMT in more clinically relevant of non-human primates heterotopic partial face transplantation model [22]. Vascularized bone marrow segment of mandible of cynomolgus monkey contains approximately 2 × 108 BMC per kilogram. Barth and colleagues reported rejection-free facial allograft survival from 60 to 177 days on tacrolimus monotherapy. Long-term survival on this protocol was, however, associated in all cases with development of posttransplant lymphoproliferative disorder (PTLD) [22]. Subsequent studies in this model avoided PTLD after addition of mycophenolate mofetil (MMF) to the regimen and specifically addressed the role of the vascularized bone marrow segment for allograft survival [23]. Facial segments containing bone demonstrated prolonged survival from 205 to 430 days, however, when immunosuppression was withdrawn, all grafts were rejected. In contrast, facial transplants without the mandible component experienced acute rejection of the graft by day 15. These results strongly suggest a valuable effect of vascularized bone element in the graft, presumably because of the presence of BMC, since transient chimerism was observed in four out of seven animals containing vascularized bone marrow segment [23].
Detailed description of the beneficial effect of vascularized BMT as a supportive therapy inducing tolerance to VCA is reviewed in the Chap. 73 of this book.
Based on the observation that VCA containing bone with viable bone marrow compartment could function as a vascularized carrier of BMC of donor origin, providing a continuous source of donor hematopoietic cells, many experimental and clinical studies were developed for tolerance induction via donor BMT.
Experimental Models of Supportive Therapy with Donor Bone Marrow Cells (BMC)
Transplanted organs or tissues contain large numbers of passenger leukocytes, including those of bone marrow origin cells, however, their number may be insufficient for chimerism induction. The load of donor leukocytes can be increased in recipients by infusion of donor BMCs. The use of immunomodulatory effect of donor-origin hematopoetic cells for allograft acceptance was proved by delivery of donor BMC in different forms such as cellular BMT or an unprocessed (crude) form of BMT, with or without recipient conditioning [24–26].
The goal of donor BMT-based strategies for induction of transplant tolerance is to achieve the state when donor hematopoietic cells may reach the recipient thymus and promote negative selection of newly developed donor-reactive T cells [4, 12].
Early studies of tolerance induction through mixed chimerism in mice experimental model involved lethal irradiation of recipient mice and reconstitution of bone marrow of these mice with mixed T-cell depleted hematopoietic cells of donor and recipient origin [27]. In this study authors achieved mixed lympho-hematopoietic chimeras, in which all lymphoid and hematopoietic cells were a mixture of host and donor type through the entire follow-up period. These studies provide evidence that mixed chimerism was associated with donor-specific skin graft tolerance and that tolerant state was systemic, as evidenced by in vitro measures of alloreactivity. However, these mice recipient demonstrates side effects of the lethal irradiation used as a part of conditioning regimen.
Therefore, much attention was subsequently devoted to developing nonmyeloablative methods for mixed chimerism induction [26, 28, 29]. In experimental mice model a nonmyeloablative conditioning regimen with T-cell depleting mAb of anti-CD4 and anti-CD8 on days −6 and −1 and lower doses of total body irradiation (3 Gy) on day 0 almost completely eliminated peripheral but not intrathymic T-cells. The addition of thymic irradiation (7 Gy) to the single mAb treatment allows the engraftment of fully major histocompatibility complex (MHC)-mismatched allogeneic bone marrow and the induction of tolerance for the graft. These observations documented that thymic irradiation eliminated host mature alloreactive thymocytes and this downregulation may play a role in the loss of alloreactivity [29]. Thus intrathymic chimerism is necessary and sufficient for the maintenance of tolerance, and this was also proved in rat limb allograft model performed in euthymic and thymecomized rats [3]. Central (intrathymic) clonal deletion provides a robust form of tolerance in all chimerism-related approaches, even to the most immunogenic tissue, such as skin. Clonal deletion is usually considered superior to regulatory or anergic mechanisms since clonal deletion physically eliminates T cells with certain specificity [30].
Donor T-cell depleted BMC transplantation for chimerism induction was reported in a rat heterotopic osteomyocutaneous flap model transplanted to a mixed allogeneic chimera [5]. Mixed allogeneic chimeras were created 4–6 weeks before osteomyocutaneous flap transplantation. Rats were subjected to total body irradiation (TBI) with 600–300 cGy and transplantation of 100 × 106 T-cells depleted with anti αβ-TCRmAb bone marrow cells (day 0), followed by an 11-day course of tacrolimus and ALS (day 10) therapy. The long-term VCA survival was significantly better (57.1 %) in chimeras receiving more than 300 cGy TBI and anti-αβ-TCRmAb as no long-term VCA acceptance was observed in animals treated with 300 cGy TBI without anti-αβ-TCRmAb preconditioning. Higher levels of chimerism, from 38.6 to 45.2 %, were associated with VCA acceptance; however, the majority of flap acceptors lost peripheral blood chimerism within 6 months, but donor-origin cells were still present within transplanted bone [5].
A more clinically relevant model with donor BMC for chimerism induction and limb allograft survival was also studied by simultaneous transplantation of donor vascularized BMT or intravenous BMT. In this study limb allografts between BN donors and Lewis recipients were performed under triple immunosuppressive protocol given for 12 weeks. Authors reported that induction of donor chimerism in the peripheral blood and lymphoid organs of the recipients was accomplished via central and peripheral mechanism and this promoted acceptance of the hind-limb allograft [31]. Recent report of simultaneous VCA and BMT for allograft survival performed under varying doses of TBI, FK-506 and ALS introduced beneficial effect of donor BMT for chimerism induction and allograft survival. Authors suggest that long-term osteomyocutaneous flaps survival was associated with Treg-mediated immunosuppression and thymic deletion of alloreactive T cells [32].
Elimination of memory T-lymphocytes or inhibition of T-cell activation represents a critical mechanism in induction of transplantation tolerance. In experimental model T-cell activation is effectively inhibited by costimulatory blockade. Costimulation blockers represent a targeted approach to modulate alloresponses, preventing activation signals at the early stage of T-cell activation between the antigen presenting cells (APC) and T-lymphocytes. A variety of BMT protocols for chimerism induction using costimulation blockade has been developed in recent years [12, 24, 33–37].
Series of experiments for chimerism induction via BMT and costimulatory blockade as a part of conditioning regimen were introduced by Wekerle and co-workers on mice model [33, 34, 37]. In addition to costimulation blockade of an anti-CD40 ligand antibody and cytotoxic T-lymphocyte antigen 4 immunoglobulin (CTLA4Ig), a low dose (3 Gy) of TBI and fully MHC mismatched allogeneic BMT reliably induced high levels (>40 %) of stable (>8 months) multilineage donor chimerism. Chimeric mice were immunocompetent as permanently accepted donor skin grafts (>100 days), and rapidly rejected third party grafts. Progressive deletion of donor-reactive host T cells occurred among peripheral CD4(+) lymphocytes, beginning as early as 1 week after BMT. Early deletion of peripheral donor-reactive host CD4 cells also occurred in thymectomized, similarly treated marrow recipients, demonstrating a role for peripheral clonal deletion of donor-reactive T cells after allogeneic BMT in the presence of costimulatory blockade [37].
To avoid TBI, subsequent experiment demonstrate that treatment of naive mice with a high dose of fully MHC-mismatched allogeneic bone marrow, followed by one dose each of mAb against CD154 and CTLA-4Ig, resulted in multi-lineage hematopoietic macrochimerism (of about 15 %) that persisted for up to 34 weeks. Long-term chimeras developed donor-specific tolerance (donor skin graft survival of more than 145 days) and demonstrated ongoing intrathymic deletion of donor-reactive T cells [34]. Thus, in both study’s authors have shown that high levels of chimerism and systemic T cell tolerance can be reliably achieved without myeloablation or T cell depletion of the host. Chronic immunosuppression and rejection were avoided with this powerful, nontoxic protocol to inducing tolerance.
To make non-myeloablative conditioning regimen and supportive therapy with BMT clinically applicable for VCA a large-animal models were developed. Non-myeloablative protocol with costimulatory blockade CTLA4-Ig, TBI (100 cGy), thymic irradiation (700 cGy), FK506 and supportive therapy with donor BMT 15, 30, or 60 × 106 cells per kilogram of whole unmodified BMC was used for limb allograft in Yucatan miniature swine model. Microchimerism was established in all animals after BMC infusion and at postoperative day 9, and was significantly increased for animals received 60 × 106 cells per kilogram (P = .0001). Animals in this group demonstrated significantly prolonged muscle and skin survival beyond 150 days posttransplant in two of three animals. Authors concluded that donor BMC infusion and immunomodulatory protocols with costimulatory blockade (CTLA4lg) might facilitate immune tolerance and eliminate the need for multidrug immunosuppression to maintain VCA survival [38]. Clinical attempts to reduce the immunosuppression required by induction of an immunologically permissive state have so far been unsuccessful. Earlier report of tolerance induction via donor BMT in VCA was introduced by Horner et al. in a preclinical large animal model [39]. Donor hematopoietic stem cell engraftment was induced by T-cell depletion, irradiation, and a short course of CsA administered to the recipient, along with a cytokine mobilized hematopoietic stem cell infusion (15 × 109 per kg bw) from a single haplotype MHC mismatched miniature swine donor. Following confirmation of donor hematopoietic cell engraftment primarily vascularized skin flaps and secondarily vascularized conventional skin grafts were transplanted from the donor. Tolerance was defined as no evidence of rejection at 90 days following transplantation. Conventional skin grafts only achieved prolonged survival (<65 days), in contrast, there was indefinite vascularized skin flap survival (over 300 days) with the achievement of tolerance in hematopoietic stem cell engrafted animals. Acceptance of a subsequent donor split thickness skin graft transplanted 124 days following the original skin flap demonstrated robust tolerance.
Tolerance to VCA in a model with higher clinical relevance, heterotopic limb transplantation miniature swine model across both haploidentical and fully mismatched MHC barriers after a non-myeolablative, radiation-free conditioning protocol was introduced by Hettiaratchy et al. [40]. Limb transplant and donor hematopoietic cell infusion with either bone marrow or cytokine-mobilized peripheral blood mononuclear cells (PBMC) were performed under 30 days’ of CsA protocol. Mixed chimerism was detected in recipients of PBMC but not in bone marrow recipients; however, animals from both groups developed split tolerance to musculoskeletal tissues but skin was rejected by day 60. In addition, all animals with evident chimerism developed cutaneous GvHD approximately 70 days post-transplant. GvHD was resolved with a short course of CsA or methylprednisolone; however, this was a significant complication, which would not likely be considered acceptable in a clinical setting.
Supportive therapy with donor BMT for VCA in large animal canine model of myocutaneous rectus flap was studied by Mathes et al. [41]. Stable mixed chimerism was established in dogs by given a sublethal dose (1–2 Gy) TBI before unselected donor BMT, and a short course of immunosuppression consisted with MMF and CsA or MMF and sirolimus after dog leukocyte antigen-identical marrow transplantation. Vascularized composite allografts of myocutaneous rectus flap from marrow donors were performed based on the deep inferior epigastric vessels, after a median of 36 months (range, 4–54 months) after BMT without post-transplant immunosuppresion. Donor chimerism was established in granulocytes ranged from 20 to 98 % and in lymphocytes population from 40 to 100 %. All bone marrow recipients maintained mixed donor-host hematopoietic chimerism and accepted VCA including skin for periods ranging between 52 and 90 weeks; in contrast, marrow donors rejected VCA from their respective marrow recipients within 18–29 days. Interestingly, increased levels of FoxP3 expression were detected in both the skin and muscle of VCA in chimeric animals, and in regional draining lymph nodes, suggesting a role of Treg in VCA tolerance. These observations suggest that nonmyeloablative BMT may form the basis for future clinical applications rather for solid organ transplantation from living HLA-identical donors, since VCA never will be performed by chimera creation from living HLA-matched donors.
Protolerogenic T-Regulatory Cells Therapies
Regulatory cells are defined by their functional capacity to suppress immune response. This concept was initially proposed following observation in experimental transplantation models that donor-specific tolerance could be transferred to naïve host via adoptive transfer of cells [42]. Identification of regulatory T-cells (Treg) CD4+/CD25+ initiated extensive research of CD4+/CD25+ cells and their ability to control variety of immune responses. Tregs are produced in the thymus as functionally mature subpopulation of T-cells or Tregs can be induced from naïve T-cells in the periphery [43]. Naturally occurring CD4+/CD25+/FoxP3+ T-regulatory cells (nTreg) derive from thymus and comprise 3–10 % of the peripheral CD4+ subpopulation of T-cells in humans. Expression of transcriptional factor forkhead box P3 (FoxP3) is required to confer the suppressive capacity of Treg [44]. Induced Treg (iTreg) can be generated from naïve T cells in vitro or induced in the periphery in vivo independently from thymic selection after alloantigen stimulation, and their differentiation is depend on cytokine milieu [45]. In the presence of IL-10 or TGF-β naïve T-cells upon activation acquire phenotypical and functional characteristics of Treg by FoxP3 expression and regulatory cytokines production.
Suppressive function of Treg on naïve or effector T cells can be accomplish by different mechanisms including: interaction between stimulatory signal coming from IL-2 and inhibitory signal derived from glucocorticoid-induced TNF receptor family related gene (GITR) and CTLA-4; functional modification of APC by downregulation of co-stimulatory molecules CD80, CD86 expression; and cell-contact-dependent immunosuppression and secretion by the Treg of immunosuppressive cytokines (TGF-β and IL-10) [42, 46–48].
Regulatory T cells are currently on focus in transplantation procedures as cells with tolerogenic properties. They plays an important role in maintain immunological unresponsiveness to self-antigens and in suppressing excessive immune responses deleterious to the host. Many studies confirmed that activation of CD4+/CD25+ Tregs represents an essential factor of the immunoregulatory pathways that create peripheral tolerance to allograft [49].
The therapeutic application of Treg in VCA was recently reported by Wekerle and colleagues by using MHC-mismatched skin transplantation model in mice [50]. The infusion of recipient nTreg and iTreg together with donor hematopoietic cells resulted in induction of long-term mixed chimerism and donor-specific transplantation tolerance to the skin allograft. The recipient mice were conditioned only with short-course of costimulatory blockers and rapamycin. Authors concluded that supportive therapy with Treg in this VCA model allow for tolerance induction without cytoreductive conditioning, thus eliminating risk of toxicity, that would be easily translated into clinical application.
In clinical experience of VCA the presence of FoxP3 positive cells and mRNA FoxP3 expression in skin biopsies taken from hand and first face transplant recipients were reported by French team. The presence of Treg within the graft was associated with the level of suppressive cytokine profile TGF-β and IL-10 in well accepted hand and face transplant recipients [51, 52]. Furthermore, our own observation of first USA near-total face transplant patient documented the presence of FoxP3 positive cells within cellular infiltrates in the skin component of the graft at the time of clinical manifestation of rejection [53
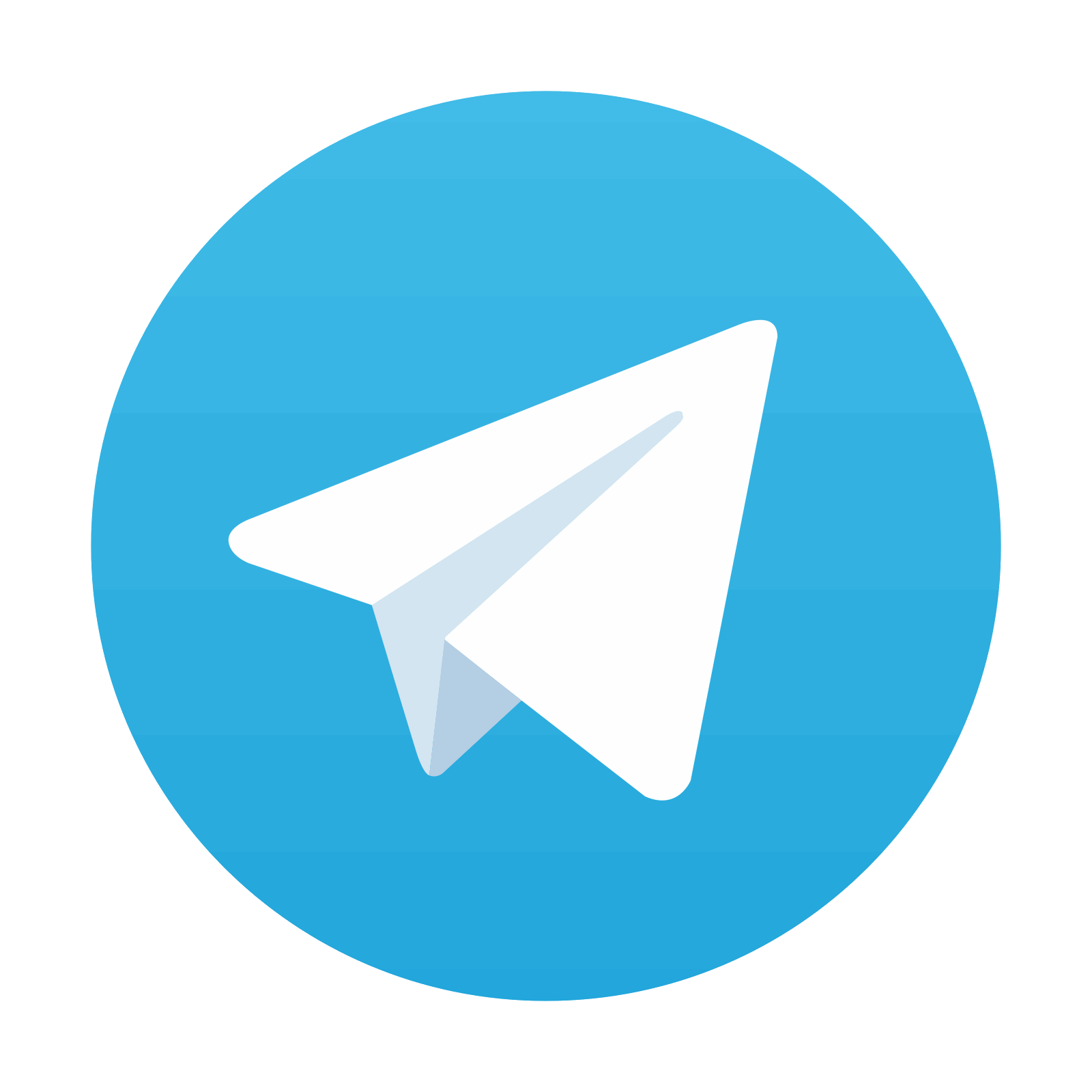
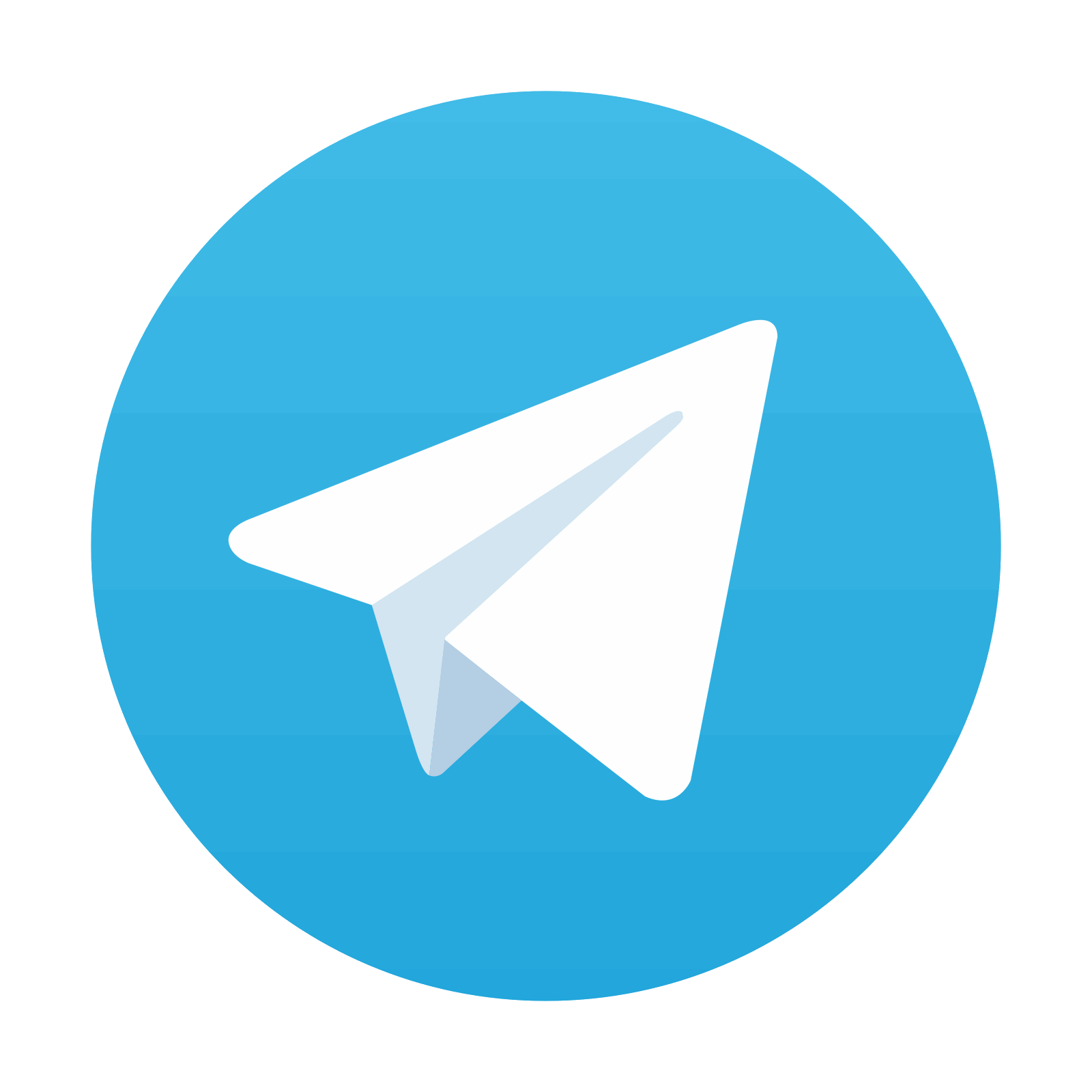
Stay updated, free articles. Join our Telegram channel

Full access? Get Clinical Tree
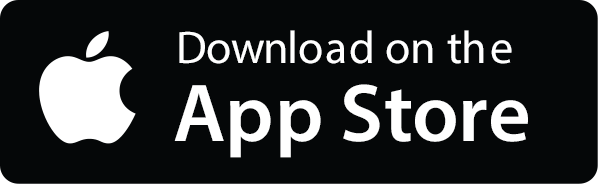
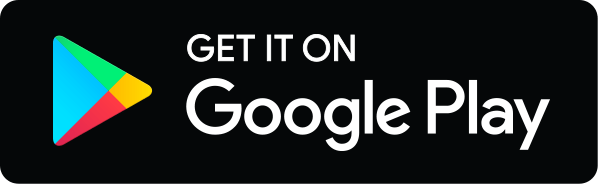