Fig. 76.1
Experimentally tested mesenchymal stem cell therapy sources and their delivery routes for improved nerve regeneration. Isolated from human bone marrow-derived mesenchymal stem cells (BM-MSC), umbilical cord-derived mesenchymal stem cells (UC-MSC), adipose tissue-derived stem cells (ADSC), and muscle-derived stem/progenitor cells (MDSPC) can be applied directly through injection into reconstructed nerve conduit in vivo (upper right panel) or cultured in vitro inside biologic nerve conduit and transplanted to the patient as an off – the – shelf construct (lower right panel)
Mesenchymal stem cells are multipotent stromal cells that can differentiate into a variety of cell types, including myocytes, chondrocytes, osteoblasts, and adipocytes. The properties of MSC vary depending on their tissue source origin, cell harvest method, cell culture passage number as well as MSC donor itself which can affect their clinical applications [11]. MSC are immunologically privileged as they present low expression of major histocompability complex (MHC) class I and are negative for MHC class II markers. They can also modulate immune response by suppressing T-cell proliferation. These properties make them a great candidate for tissue engineering applications or supportive cellular therapies which can be administered either locally or systemically. It has been shown that MSC derived either from bone marrow or umbilical cord blood can differentiate into Schwann cell-like phenotype and promote axonal regeneration and myelin reconstruction [12]. The therapeutic effect of MSC transplantation on nerve regeneration was confirmed both in animal models and in humans [8].
It has been shown that nerve regeneration can also be enhanced by MSCs derived from umbilical cord. Under specific conditions, these cells can differentiate into Schwann cells (UC-SCs) and as such promote functional recovery of damaged nerve [13]. Adipose tissue-derived stem cells represent another source of stem cells used for nerve regeneration. These cells have similar phenotypic and gene expression profiles to BM-MSC and can be easily and safely harvested from subcutaneous fat tissue by conventional liposuction procedure. It has been shown that ADSC can be stably differentiated into Schwann-like cells and promote peripheral nerve repair through secretion of a range of neurotrophic factors, such as nerve growth factor (NGF) and brain-derived nerve factor (BDNF) [14, 15].
Muscle-derived stem/progenitor cells are a population of slowly adhering cells. They are characterized by sustained self-renewal, long-term proliferation, and multipotent differentiation capacity [16]. It has been demonstrated that MDSPC isolated from human skeletal muscle (hMDSPC) have the capacity to gain neuronal and glial phenotypes and ameliorate sciatic nerve injury in mice [16]. Alternative sources of MSC include amniotic membrane, amniotic fluid, umbilical cord matrix (perinatal MSC), dental pulp stem cells (DPSC), stem cells from exfoliated deciduous teeth (SHED), periodontal ligament stem cells (PDLSC), stem cells from apical papilla (SCAP), dental follicle progenitor cells (DFPC), as well as hair follicle derived stem cells [17–20]. Further studies investigating differences in the biological properties between perinatal and adult MSC as well as their relevance for the clinical application are required. Introduction of a new MSC sources will complement the current MSC inventory and help determine the optimal MSC therapy for a specific disease.
Future Potential Sources of Cells for Nerve Regeneration
The future cellular therapies for peripheral nerve injuries treatment may include the use of two distinct populations of stem cells: very small embryonic-like cells and induced pluripotent stem cells.
Very Small Embryonic-Like Stem Cells
Very small embryonic-like stem cells (VSEL) are a population of pluripotent stem cells residing in a variety of adult organs and tissues [21]. It has been proposed that these cells represent a reserve pool of precursors for tissue-committed stem cells that can be accessed in response to physiological stress [22]. They are larger than platelets but smaller than erythrocytes (3–5 μm in diameter in mice and 5–7 μm in diameter in humans) and display several features typical for embryonic stem cells such as small size, a large nucleus surrounded by a narrow rim of cytoplasm, and open-type chromatin (euchromatin). These cells are enriched in the Sca1+Lin−CD45− cell fraction in mice and the CD133+Lin−CD45− cell fraction in humans [23]. Little is known about the ability of these cells to differentiate into Schwann cells and their role in peripheral nerve regeneration. So far VSEL have demonstrated the potential to differentiate into neurons, oligodendrocytes, and microglia and have been proposed to be harnessed for regeneration of damaged Central Nervous System [24].
Induced Pluripotent Stem Cells
Induced Pluripotent Stem Cells (iPSC) are created via genetic reprogramming of normal somatic cells (i.e. fibroblasts, hematopoietic cells). In this approach, somatic cells are genetically modified to express several genes specific for embryonic stem cells (i.e. Oct-4, Nanog, Klf-4 and c-Myc) [25]. It has been shown that coating the nerve conduits with iPSC-derived neurospheres can accelerate peripheral nerve regeneration in mice [26]. Another study has shown that nerve regeneration can also be promoted by application of nerve conduits coated with neural crest stem cells (NCSC) derived from iPSC [27]. Despite positive results of iPSC-based therapy on nerve regeneration in animal models, clinical application of this approach remains controversial as it was reported that transplanted iPSC can form teratomas and other primitive tumors [28].
The Effects of Biological Conduit and Cellular Therapy Interplay
Studies on conduits clearly indicated the importance of favorable environment to optimally enhance nerve regeneration. Both synthetic and biological conduits demonstrated various degree of success when augmented with trophic factors [29]. For the past 20 years, Siemionow’s group has aimed to use cellular therapies as a more effective long-term delivery system of nerve-specific growth factors. Siemionow’s group focused on bone marrow-derived stromal cell (BMSC), alternatively named bone marrow mesenchymal stem cell, therapy due to its reported immunomodulatory and nerve supporting properties [12, 13]. In a study comparing the effect of a 20 mm nerve defect repair using isogenic epineural tube with or without the support of isogenic BMSC on the regeneration of the rat sciatic nerve, Siemionow’s group found evidence of successful sensory and functional recovery in both groups at 12 weeks post-repair [30]. However, histomorphological MSC assessment of the group supported by BMSC presented significantly increased myelin thickness and larger diameter nerve fibers. Immunostaining confirmed expression of nerve growth factor (NGF) and glial fibrillary acidic protein (GFAP) by the isogenic BMSC cells observed in the conduit. The expression of these two neuronal markers indicated that BMSC differentiated in vivo into cells presenting neural phenotype [30]. Phenotype change of BMSC could be facilitated by interaction of cells with the epineural tube, which expresses VEGF and laminin B, an extracellular protein constituting a biologically active part of the basal laminae, influencing cell differentiation, migration, and adhesion. Improved nerve regeneration was also obtained in a study where isogenic venous graft supported with isogenic BMSC was applied to bridge 20 mm nerve gap [31]. BMSC filled venous graft showed significantly better regeneration of the nerve defect compared to controls, as confirmed by functional recovery measured by somatosensory evoked potential (SSEP), toe-spread, pin prick, and gastrocnemius muscle index (GMI) [31]. Study comparing the effectiveness of autologous venous graft augmented with muscle graft to autologous venous-muscle graft augmented with BMSC showed a higher number of Schwann cells in the group supported with BMSC [32]. To further study the interplay between the BMSC and epineural tube and its effect on nerve regeneration, Siemionow’s group created a conduit filled with BMSC and cultured it for three weeks. The results showed that the secretion of NGF by epineural conduit filled with BMSC was the highest at day 10 of culturing (1,000 pg/ml) and enhanced compared to BMSC and epineural tube cultured separately [33]. In the second part of this study, cultured constructs were used in vivo in rat model and showed improved sensory and comparable functional regeneration to the epineural tube filled with saline, as confirmed by toe-spread, GMI, and SSEP assessments. Better nerve regeneration following implantation was accompanied by expression of H-neurofilaments and expression of CD31, VEGF, and NGF by BMSC inside the epineural conduit [34, 35]. The use of the conduit showed improved nerve regeneration in pathological conditions as well. Using diabetic ZDF rat model, Siemionow’s group showed nerve recovery comparable to the gold standard of autograft repair [36].
Application of epineural tube supported by BMSC presented promising results when tested in the large animal model [37, 38]. Siemionow’s group selected the sheep model to test the restoration of 6 cm long median nerve defect due to the similarity of the nerve anatomical structure to human nerve. Autogenic/allogenic epineural sheath conduit was created using sheep median nerve and augmented by 70–80 × 106 of cultured autogenic/allogenic sheep BMSC. Nerve conduction velocity and SSEP performed three and six months after nerve repair confirmed the neurosensory response and regenerative properties of the auto- and allogenic conduits. Immunofluorescent staining of saline and BMSC filled conduit showed the presence of fascicle-like structures in the proximal, middle, and distal parts of the conduit. However, smaller diameter fascicles were observed in BMSC filled conduits compared to conduits filled with saline.
Currently, Siemionow’s group is testing in vivo the effects of human conduit consisting of human epineural sheath and 3–4 × 106 human mesenchymal stem cells [39]. Preliminary results are encouraging. Clinical evaluation and SSEP analysis confirmed sciatic nerve recovery with outcomes comparable to nerve autograft repair. GMI analysis and muscle histomorphometry showed comparable muscle mass restoration and mean muscle fiber area to the autograft control. Immunostaining showed the presence of human MSC in the conduit at 12 weeks post-transplantation [40]. The role of local human MSC function is currently under investigation.
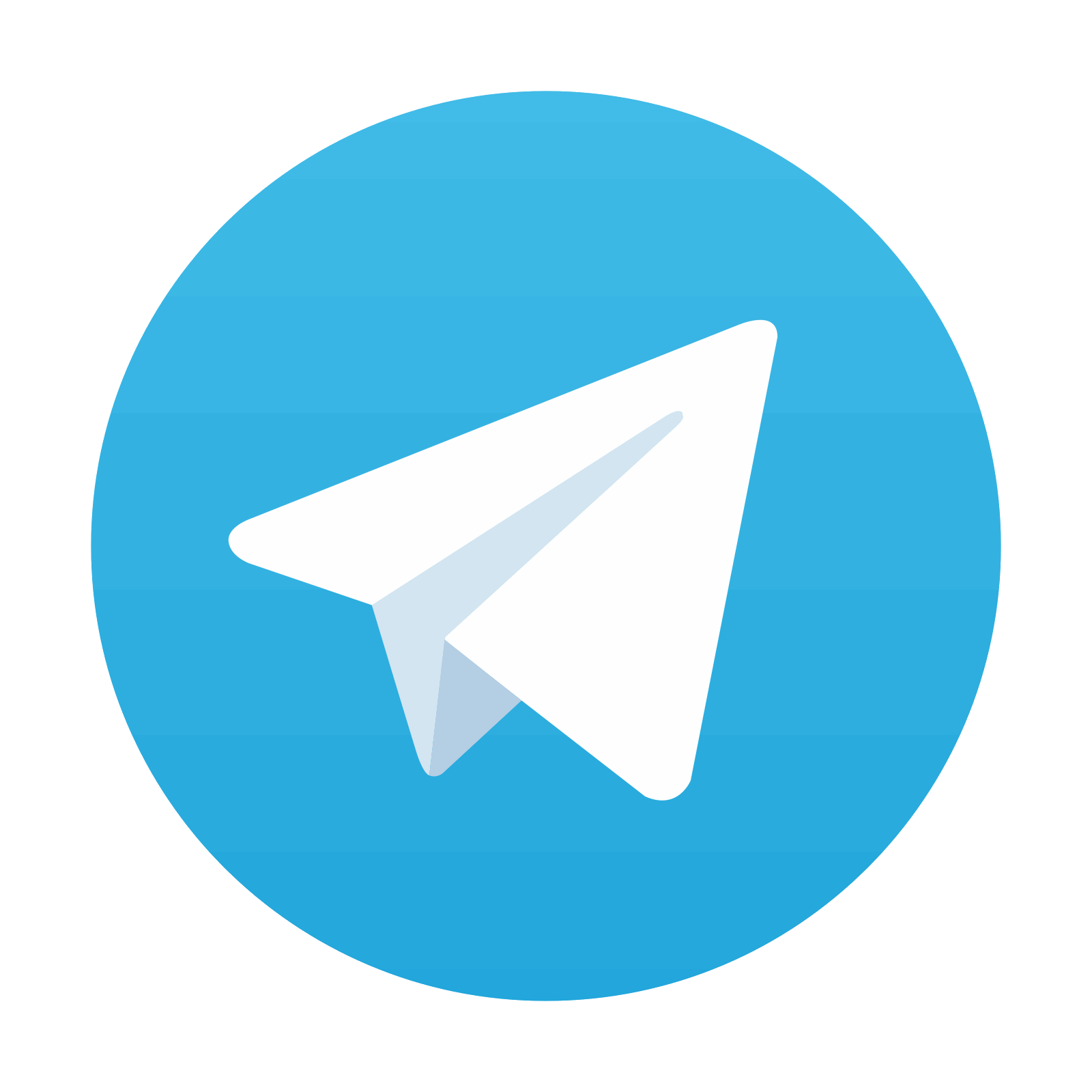
Stay updated, free articles. Join our Telegram channel

Full access? Get Clinical Tree
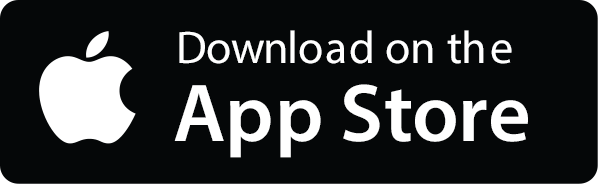
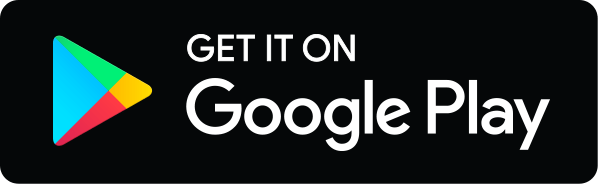