Botulinum Toxin: Introduction
On April 15, 2002, the US Food and Drug Administration (FDA) approved Allergan, Inc.’s Botox Cosmetic* (botulinum toxin type A) for “temporary improvement in the appearance of moderate-to-severe glabellar lines in adult men and women 65 or younger.” This was the first elective cosmetic indication for which any commercially available botulinum toxin on the US market had been approved.
The toxin had previously been approved and was marketed as Botox® for the treatment of strabismus, blepharospasm, and cervical dystonia but existing off-label cosmetic use of the toxin had already propelled annual sales to $250 million per year in 2002. With FDA recognition of the cosmetic indication, sales of both Botox® and Botox Cosmetic® surpassed $1 billion per year by the end of 2009.
The commercial introduction of Allergan’s product into the US market has given unprecedented brand name equity to the drug, surpassed only by Pfizer’s product Viagra® (sildenafil citrate). Botox has passed into vernacular usage as a trademark used to loosely refer to a class of biologic neurotoxins in the manner of other well-known trademarks such as Coke® and Kleenex®. The clinical utility of the neurotoxin is so widely known today that the word Botox has become a generic term in the public mind for all agents used in cosmetic neurotoxin therapy, although the term is properly reserved for Allergan’s trademarked commercial version of the type A neurotoxin complex.
In April 2009, the FDA approved a third commercial neurotoxin, a serotype A product, Dysport® (Ipsen [UK]/Medicis [US]) to join Allergan’s Botox®/Botox® Cosmetic and a previously approved serotype B product, Myobloc® (Solstice Neurosciences [US]). In July 2011, the FDA approved a fourth commercial neurotoxin for the US market, a serotype A product, Xeomin® (Merz Pharma GmbH [Germany]). To emphasize the noninterchangeability of these biological toxins, the FDA in August 2009 required the manufacturers to adopt new drug names: onabotulinum toxin A (Botox®/Botox® Cosmetic), abobotulinum toxin A (Dysport®), rimabotulinum toxin B (Myobloc®), and incobotulinum toxin A (Xeomin®).
One other commercial serotype A toxins is presently awaiting FDA approval: PurTox® (Mentor [US]). There are at least two other commercial serotype A toxins in use outside of the United States: Meditoxin®/Neuronox® (Medy-Tox [Korea]) and Hengli®/Prosigne® (Lanzhou [China]). Presumably the FDA will supply new drug names for these products when and if they complete the approval process.
History
The medical history of botulinum toxin dates back to the Holy Roman Empire at the beginning of the nineteenth century in central Europe. The Napoleonic wars eroded rural hygiene and spread poverty throughout what is now southern Germany from 1795 to 1813.1 A 29-year-old health official, Justinus Kerner, observed a connection between spoiled sausage fat and a paralytic illness, which he reported to medical authorities in 1815; his findings were published as a monograph in 1820. His observations provided compelling epidemiologic evidence for the cause of a disease eventually known as botulism, after botulus, the Latin word for sausage.
In 1822, Kerner reported on a series of experiments, first on animals and later on himself, in which he demonstrated that a poison could be extracted from affected sausage that would induce weakness and anhidrosis. In a fascinating example of forward thinking, Kerner speculated that minimal doses of the toxin might be useful to treat a variety of disorders of the nervous system. Seventy-five years later, Emile-Pierre van Ermengem published the initial description of the causative organism, Clostridium botulinum, demonstrating production of a toxin that produced weakness in animals.
In the 1920s, Dr. Herman Sommer at the University of California, San Francisco, isolated the toxin as a stable acid precipitate.2 In 1944, Edward J. Schantz, PhD, began purification of botulinum toxin A in crystalline form,3 and in the 1950s, Dr. Vernon Brooks first demonstrated that botulinum toxin A blocked release of acetylcholine from motor neurons at the neuromuscular junction.2
In the early 1970s, Dr. Alan Scott, a San Francisco pediatric ophthalmologist, together with Schantz, sought to develop a biologic toxin that would be useful in paralyzing muscular action around the eyes. He identified botulinum toxin as an agent useful in treating human strabismus,4 and predicted that it would be used to treat other conditions of muscle spasm or hyperactivity. Scott originally named this product Oculinum.
In the early 1980s, Dr. Jean Carruthers, a Vancouver-based oculoplastic surgeon, visited Scott’s laboratory and reported her experience with botulinum toxin in the treatment of ocular disorders.5 Her husband, Dr. J. Alastair Carruthers, and Drs. Theodore Tromovitch, Samuel Stegman, and Dr. Richard Glogau were already investigating the use of solubilized bovine collagen as a soft-tissue filler to soften glabellar frown lines for cosmetic improvement.6
The era of the use of botulinum toxin for reduction of skin lines began when Dr. Jean Carruthers observed a smoothing effect on the glabellar brow furrows in patients injected with toxin for blepharospasm.5 The effect was much more profound than that which could be achieved using collagen fillers. Carruthers and Carruthers published a report on their novel therapeutic approach in 1992.7
By this time, the product was being produced commercially under the brand name Botox® and was being used to treat blepharospasm, strabismus, torticollis, hemifacial spasm, and other focal dystonias.8 There was initial resistance to the use of botulinum toxin for cosmetic indications, because physicians as a group were wary about using a paralytic agent, the most potent known to medical science, for elective cosmetic purposes. But the careful clinical observations and persuasive teaching of the Drs. Carruthers, acknowledged pioneers in the cosmetic use of botulinum toxin, gradually recruited more and more practitioners to the implementation of their techniques. The benefits as well as the risk-benefit ratio were better understood, and broad acceptance followed.
Myriad therapeutic uses for botulinum toxin—apart from the treatment of blepharospasm, strabismus, torticollis, hemifacial spasm, and other facial dystonias8—have evolved since 1992, including treatment of hyperhidrosis,9 migraine headaches,10 and chronic conditions such as lower back pain,11,12 limb spasticity secondary to disease or injury of the central nervous system, incontinence due to neurogenic or overactive bladder, vocal cord dystonias, achalasia (failure of lower esophageal-gastric sphincter to relax), temporal-mandibular joint disorders, neuralgias and focal neuropathies, and aesthetic facial sculpting through reduction of masseter muscle volume.
Pharmacology
Botulinum neurotoxins are currently categorized into seven distinct serotypes: A, B, C1, D, E, F, and G.13 The molecules vary in their biosynthesis, size, cellular sites of action, binding kinetics, duration of effect, and stability. The serotypes currently commercially available, serotypes A and B, are derived from different strains of Clostridium botulinum. They both have 150-kDa dichain polypeptides with a heavy chain and light chain linked by disulfide bonds. During biosynthesis, the molecules of A and B can be surrounded by proteins to form a neurotoxin complex, ranging from 500 kDa to 900 kDa (Fig. 255-1). Xeomin® (incobotulinum toxin A) consists of the 150 kDa dichain without accessory proteins.
Figure 255-1
Schematic of the botulinum complex showing the binding domain with the N-terminus (yellow) and the C-terminus (red), together with the translocation domain (green) and the light chain (blue). (Used with permission from Turton K, Chaddock JA, Acharya KR: Botulinum and tetanus neurotoxins: structure, function and therapeutic utility. Trends Biochem Sci 27:554, 2002.)
The toxin enters the nerves by binding to surface protein receptors and undergoing endocytosis into internalized vesicles. The light chain is released into the nerve cytosol, and the SNARE (soluble N-ethylmaleimide-sensitive factor attachment protein receptor) protein complex is cleaved to inhibit exocytosis of the neurotransmitters such as acetylcholine (Fig. 255-2). Type A toxin cleaves SNAP-25 (synaptosome-associated protein of 25 kDa), whereas type B cleaves VAMP (vesicle-associated membrane protein), also called synaptobrevin (Fig. 255-3). These proteins are necessary for the release of acetylcholine from vesicles within the cytoplasm of the motor nerve endings. The binding characteristics of each serotype dictate the locus of action on the intracellular SNARE protein complex (Table 255-1).
Figure 255-2
The heavy chain domain of the botulinum neurotoxin complex binds to the plasma membrane receptor (1) and the complex is internalized (2). The light chain fragment is then released into the cytoplasm (3), where it cleaves the SNARE (soluble N-ethylmaleimide-sensitive factor attachment protein receptor) protein complex at a site determined by the neurotoxin serotype (4). This disruption of the SNARE complex prevents exocytosis of acetylcholine (ACh) into the synaptic space of the neuromuscular junction. A through G = neurotoxin serotypes; AChR = acetylcholine receptor; LC = light chain; HC = heavy chain C-terminus; HN = heavy chain N-terminus; SNAP-25 = synaptosome-associated protein of 25 kDa; VAMP = vesicle-associated membrane protein. (Used with permission from Turton K, Chaddock JA, Acharya KR: Botulinum and tetanus neurotoxins: Structure, function and therapeutic utility. Trends Biochem Sci 27:555, 2002.)
Figure 255-3
Schematic representation showing the sites of action of the different botulinum serotypes on the intracellular protein complex known as the SNARE (soluble N-ethylmaleimide-sensitive factor attachment protein receptor) proteins responsible for exocytosis of acetylcholine from the nerve. Botulinum A serotype cleaves SNAP-25 (synaptosome-associated protein of 25 kDa). Other serotypes impact VAMP (vesicle-associated membrane protein), synaptobrevin, or syntaxin. BoNT/A through BoNT/G = botulinum neurotoxin serotypes A through G; TeNT = tetanus neurotoxin.
Serotype | Intracellular Protein |
---|---|
A | SNAP-25 |
B | VAMP/synaptobrevin |
C1 | SNAP-25 and syntaxin |
D | VAMP/synaptobrevin |
E | SNAP-25 |
F | VAMP/synaptobrevin |
G | VAMP/synaptobrevin |
The end result is a chemodenervation of the cholinergic neurons, either motor nerves or autonomic nerves, leading to localized absence of skeletal muscle activity or autonomic control of target organs such as the eccrine sweat glands. The way in which the nerves escape the effect of the neurotoxin is partially understood.14 The chemodenervated nerve endings develop collateral sprouting near the primary terminus of the nerve. These sprouts eventually make proximate contact with the targets, either muscle or gland, and begin to overcome the loss of neurotransmitter at the end organ synaptic junctions.
Once these sprouts have reestablished chemical contact with their targets, muscles resume activity and glands begin to secrete. Simultaneously, the original chemodenervated terminal nerve ending begins to degrade the blocked SNARE proteins and develop new proteins to resume the chemical exocytosis of acetylcholine. While these repairs are underway, and while the original terminal nerve ending reacquires communication with the target organ, the collateral sprouts begin to slowly resorb until anatomically nothing is left but the original terminal ending with restored junctional activity.
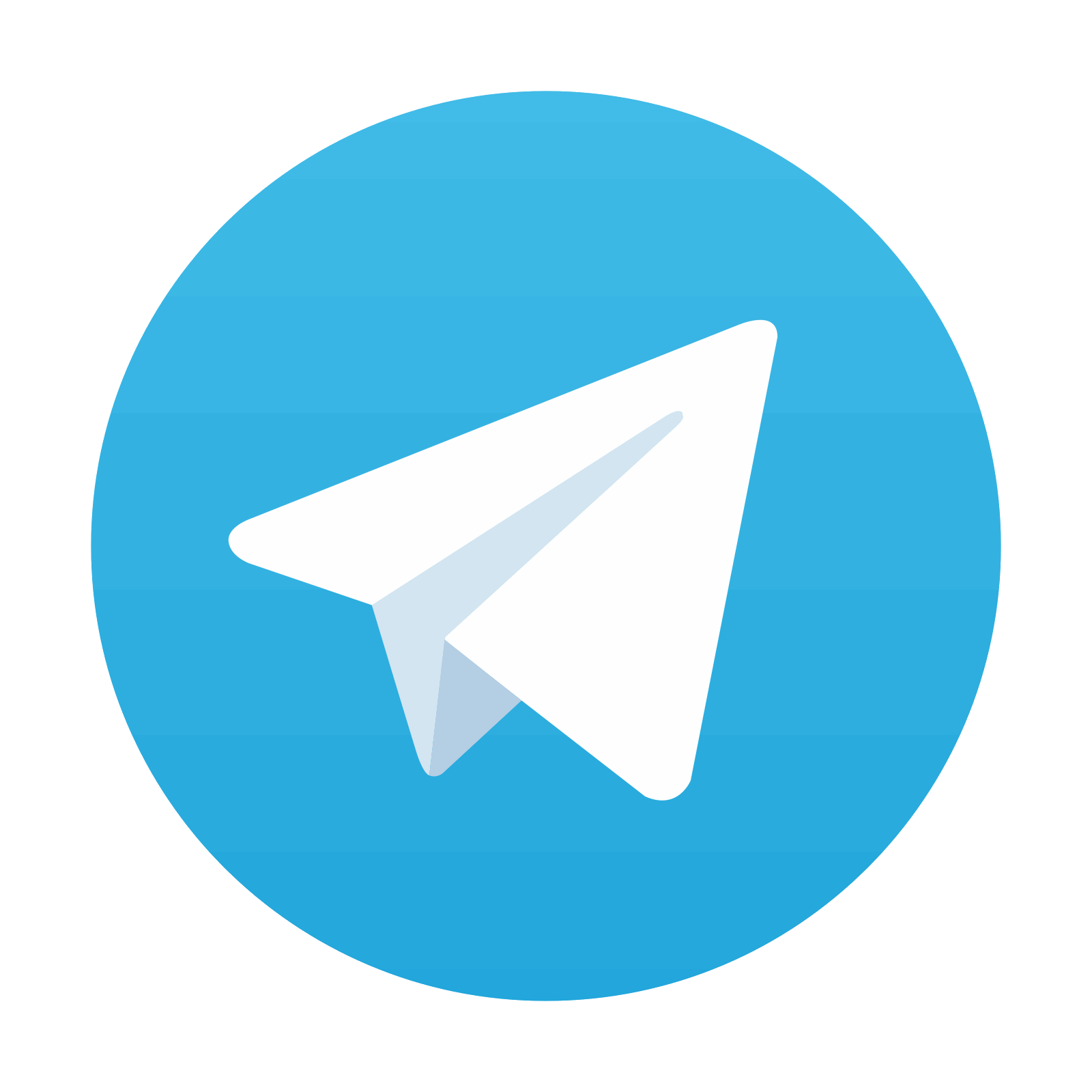
Stay updated, free articles. Join our Telegram channel

Full access? Get Clinical Tree
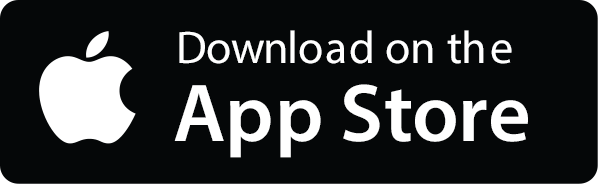
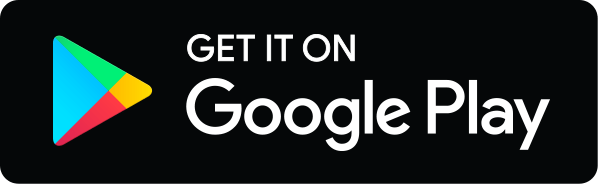