Bioderm Research
Linda Walker
CoValence, Inc.
ABSTRACT
This chapter focuses on one of humanity’s greatest fears in life: old age, wrinkles, and skin aging. While the primary focus has heretofore been on the treatment of women, we see an accelerating shift towards enhanced interest by men as well. This overall trend offers stimulation for new product development that works based upon a continued search for understanding and thwarting the inevitability of the aging phenomenon. This is not a boring, knee-deep science filled with sky-high concepts, and this chapter is not meant to induce sleep.
Approaches to the beautification of facial skin includes lightening of skin color, smoothing of wrinkles and fine lines, reduction of surface disfigurements and blemishes, and reduction or disappearance of dark spots and circles around the eyes. These have been consumer-desirable attributes since ancient times. An arsenal of anti-wrinkle, anti-aging, skin brightening, skin-tone enhancement, and dermabrasion treatments have now become available for skin anti-aging cosmetics. While such treatments offer a plethora of marketing opportunities, they also tend to confuse the consumer, relative to their benefit claims. Consumers thus tend to select multiple products to obtain their combined benefits. In reality, however, such practice may cause an overload of ingredients on skin, thereby reducing their perceived efficacy.
A “single-bullet” treatment (a bundle of ingredients that perform multiple functions) that provides a highly desirable facial glow for the multinational consumer has been a focus of current active research for some time. The first such single-bullet treatment is based on anti-senescence, a fundamental approach to retarding aging described in recent patents by Gupta and Walker (1).
Aging at the cellular level is characterized by oxidative stress, inflammation, and cell senescence (2). Cells are the fundamental structure composing our bodies, and cellular senescence in humans causes the cells to stop replicating themselves through the process of mitosis. This process results in cellular degradation over a period of time, thus contributing to the aging process. Cellular senescence also initiates oxidative stress and inflammation. Such changes range from those affecting cells and their function to the whole organism. The management of cellular senescence is thus essential for any single-bullet skin anti-aging regimen (3).
5.3.1 Role of Cellular Senescence and Apoptosis in Skin Aging1
5.3.2 Role of Enzyme Dysfunction in Skin Aging
a. Oxidative Stress and Free Radicals
d. Advanced Glycation End Products (Ages)
e. Proteasomes in Cellular Anti-Senescence
f. Mitochondrial Free Radical Theory of Aging (Mfrta)
5.3.3 Anhydrobiosis and Skin Aging
5.3.4 Osmoprotection, Cellular Anti-Senescence, and Skin Anti-Aging
a. Hyperosmarity, Inflammation, and Cellular Senescence
b. Chemical Basis of Hyperosmarity
5.3.5 New Peptide Derivatives for Anti-Senescence and Skin Anti-Aging
5.3.6 Consumer Perception and Marketing of Enzyme Biology-Based Skin Care Products
5.3.1 ROLE of CELLULAR SENESCENCE AND APOPTOSIS IN SKIN AGING
Higher organisms contain two types of cells: postmitotic cells, which never divide, and mitotic cells, which can divide. Postmitotic cells include mature nerve, muscle, and fat cells, some of which persist for life. Mitotic cells include epithelial and stromal cells of organs such as the skin. Normal mitotic cells do not divide indefinitely. The process that limits the cell division number is termed cellular or replicative senescence. Senescent keratinocytes and fibroblasts appear to accumulate with age in human skin. Moreover, senescent cells express genes that may have pleiotropic effects, such as degradative enzymes and inflammatory cytokines. Thus, relatively few senescent cells might compromise skin function and integrity. Moreover, by altering the tissue microenvironment, senescent cells may also contribute to the rise in ailments that occur with age, such as cancer, diabetes, and arthritis (4).
Cellular senescence is the phenomenon by which normal cells lose the ability to divide and produce new cells. It differs from apoptosis, which is the process of programmed cell death that occurs in multicellular organisms. Aging causes distinctive changes in cells and certain cellular molecules. Two cellular processes, apoptosis and cellular senescence, may be examples of antagonistic pleiotropy wherein the action of a gene results in multiple competing effects, some beneficial but others detrimental to the organism. (Pleiotropy is the phenomenon where one gene controls for more than one phenotypic trait in an organism. Antagonistic pleiotropy is when one gene controls for more than one trait where at least one of these traits is beneficial to the organism’s fitness and at least one is detrimental to the organism’s fitness.) Both processes are essential for the viability and fitness of young organisms, but may contribute to aging and certain age-related diseases (5) and their possible treatment via chaperone proteins (6). The apparent result of both, the cellular senescence and the apoptosis, is the net loss of cells. With chronological aging the rate of both the apoptosis and the senescence increases. Intrinsic skin aging is characterized by the progressive accumulation of senescent cells, thinning of the epidermis, decline in cell-to-cell adherence, reduction of collagen content, formation of fine wrinkles, and gradual loss of elastic material (7). The treatment of these signs of skin aging—for a “forever young” physical appearance—has been one of the foremost areas of consumer interest.
5.3.2 ROLE OF ENZYME DYSFUNCTION IN SKIN AGING
Cellular senescence has been ascribed to dysfunction of certain key enzymes and biological functions of the cell that have a direct role in the chronological process of skin aging, as illustrated below. A discussion of a select number of these follows.
a. Oxidative Stress and Free Radicals
The proof for the participation of oxidative stress in senescence are specific diseases that to some extent are gender dependent and appear more frequently in males or females (8). Redox active metals like iron (Fe), copper (Cu), chromium (Cr), cobalt (Co), and other metals undergo redox cycling reactions and possess the ability to produce reactive radicals such as superoxide anion radicals and nitric oxide in biological systems. Disruption of metal ion homeostasis may lead to oxidative stress, a state where increased formation of reactive oxygen species (ROS) overwhelms body antioxidant protection and subsequently induces DNA damage, lipid peroxidation, inflammation, protein modification, and other effects. Paradoxically, two major antioxidant enzymes, superoxide dismutase (SOD) and catalase, contain as an integral part of their active sites metal ions to battle against toxic effects of metal-induced free radicals (9).
The role of free radicals has long been proposed as a cause for the aging process. Oxidative stress is considered a major factor for altering many physiological processes and enzymatic activities during aging and is also known to play a major role in the development of several age-dependent diseases. Oxidative damage caused by free radicals includes mitochondrial dysfunction, dopamine auto-oxidation, α-synuclein aggregation, glial cell activation, alterations in calcium signaling, and excess free iron. Alterations in transcriptional activity of various pathways, including nuclear factor erythroid 2-related factor 2, glycogen synthase kinase 3β, mitogen-activated protein kinase, nuclear factor kappa B, and reduced activity of superoxide dismutase; catalase and glutathione are also the result of oxidative damage caused by free radicals. Paraoxonase 1 (PON1) is an anti-atherosclerotic enzyme that mainly prevents accumulation of lipoperoxides and inhibits the lipid oxidation in low-density lipoproteins (10). A declining endogenous defense system during senescence dictates the need for supplementation with exogenous antioxidants.
Reactive oxygen species (ROS) are thought to have deleterious effects on T-cell function and proliferation. Low concentrations of ROS in T-cells are a prerequisite for cell survival, and increased ROS accumulation can lead to apoptosis/necrosis. Antioxidants such as glutathione, thioredoxin, superoxide dismutase, and catalase all serve to balance cellular oxidative stress. T-cell redox states are also regulated by expression of various vitamins and dietary compounds (11).
These are ubiquitous organelles that perform such functions as hydrogen peroxide metabolism and β-oxidation of fatty acids. Reactive oxygen species produced by peroxisomes are a major contributing factor to cellular oxidative stress, which is supposed to significantly accelerate aging and cell death. The role of peroxisomes in aging and cell death has recently been reported (12).
The immune response declines with aging (immunosenescence), which is primarily due to T-cells’ decline. Resveratrol has been found to be capable of counteracting immunosenescence, thereby leading to cellular rejuvenation (13).
d. Advanced Glycation Endproducts (AGEs)
Reducing sugars can react non-enzymatically with the amino groups of proteins to form Amadori products. These early glycation products undergo further complex reaction such as rearrangement, dehydration, and condensation to become irreversibly cross-linked heterogeneous fluorescent derivatives, termed advanced glycation endproducts. The formation and accumulation of AGEs have been known to progress in a normal aging process and at an accelerated rate under diabetes. This is one of the reasons that diabetic skin is more prone to excessive dryness, eczema, and other clinical issues (14). There is evidence that interaction of AGEs with a Receptor for Advanced Glycation Endproducts (RAGE) elicits oxidative stress generation. The interaction between RAGE and its ligands is thought to result in pro-inflammatory gene activation and subsequently evokes inflammatory, thrombogenic, and fibrotic reactions.
e. Proteasomes in Cellular Anti-Senescence
Normal human fibroblasts undergo a limited number of divisions in culture and progressively they reach a state of irreversible growth arrest, a process termed replicative senescence. The proteasome is the major cellular proteolytic machinery, the function of which is impaired during replicative senescence. Recent work suggests that proteasome malfunction may contribute to the aging process in human skin and that the maintenance of normal proteasome activities could delay skin aging. Proteasome activities changed with age in a biphasic manner, decreasing significantly up to 50 years of age and showing no significant change between 50 and 78 years of age. The decreases in proteasome activities were accompanied by reduced levels of proteasomal peptidase activities coupled with increased levels of both oxidized and ubiquitinated proteins in senescent cells. Recent work also suggests that mitochondrial and proteasomal activities are interdependent (15).
The body constantly produces proteins and degrades proteins that are no longer needed or are defective. The production and destruction of proteins, called protein turnover, is a constant, ongoing process that is crucial for tissue renewal. A well-nourished person synthesizes nearly one pound of protein per day. Proteins that are broken down balance this protein gain. The process of protein breakdown, called proteolysis, is essential to cell survival. Numerous proteolytic systems exist in mammalian cells, the most important of which are the lysosomes, the ubiquitin-proteasome pathway, and enzymes called calpains. Lysosomes are small cell components that contain specific enzymes (proteases), which break down proteins. In the ubiquitin-proteasome pathway, proteins that are to be degraded are first marked by the addition of ubiquitin molecules and then broken down by large protein complexes called proteasomes.
Calpains are proteases that are involved in several physiological processes, including the breakdown of proteins that give cells their shape and stability. The ubiquitin-proteasome system is now considered the major system involved in intracellular protein degradation. Two major components of this system are (1) three enzymes that add a small protein called ubiquitin onto substrate proteins destined for degradation, and (2) the proteasome, a rather large cellular particle composed of several smaller protein subunits, which executes the actual proteolysis. By degrading short-lived regulatory proteins, the ubiquitin-proteasome system controls basic cellular processes such as cell division, cell signaling, and gene regulation. The system also removes misfolded, damaged proteins, and in certain immune cells it breaks down foreign proteins into pieces called antigenic peptides, which can then be transported to the cell surface to induce an immune response.
Ubiquitin is a small protein that occurs in most eukaryotic cells. Its main function is to mark other intracellular proteins for destruction, known as proteolysis. Several ubiquitin molecules attach to the condemned protein (polyubiquitination), and polyubiquitinylated protein then moves to a proteasome, a barrel-shaped structure where the proteolysis occurs. Ubiquitin can also mark transmembrane proteins (for example, receptors) for removal from the membrane.
Ubiquitin consists of 76 amino acids with two sequentially linked glycine moieties at the carboxyl terminal and has a molecular mass of about 8500 atomic mass units (amu). It is highly conserved among eukaryotic species: Human and yeast ubiquitin share 96% amino acid sequence identity.
The process of marking a protein with ubiquitin consists of a series of steps; (i) Activation of ubiquitin—the carboxyl group of the terminal glycine of ubiquitin binds to the sulfhydryl group -SH of an ubiquitin-activating enzyme E1. The sulfhydryl group is a cysteine residue on the E1 protein. This step requires an ATP molecule as an energy source and results in the formation of a thioester bond between ubiquitin and E1; (ii) Transfer of ubiquitin from E1 to the ubiquitin-conjugating enzyme E2 via trans (thio) esterification; (iii) Then, the final transfer of ubiquitin to the target protein can occur either directly from E2 (this is primarily used when ubiquitin is transferred to another ubiquitin already in place, creating a branched ubiquitin chain) or via an E3 enzyme, which binds specifically to both E2 and the target protein. The target protein is usually a damaged or nonfunctional protein that is recognized by a destruction-targeting sequence. Ubiquitins then bind to a lysine residue in the target protein via the transformation of thioester bond into an iso-peptide bond, eventually forming a tail of at least four ubiquitin molecules. The resulting ubiquitin-linked protein, called ubiquitin-protein conjugate, then can be recognized and degraded by the proteasome into peptides. This is the typical way to mark specific proteins for proteolysis.
A functional proteasome (also called 26S proteasome) is composed of a smaller barrel-shaped core and two “caps” that are attached to each end of the core. The proteasome core consists of four stacked rings containing two types of subunits, all facing into a central cavity. These subunits together have at least five distinct proteinase activities that cleave proteins at different sites. The “caps” at each end of a proteasome perform a regulatory function. Each cap is composed of multiple subunits with numerous functions. These subunits recognize the ubiquitinylated protein, cut off the ubiquitin chains from this protein, thereby “unfolding” the protein, and open the channel inside the proteasome core so that the protein can enter the channel for degradation; and (iv) Finally, the marked protein is digested in the 26S-proteasome into small peptides, amino acids (usually 6–7 amino acid subunits). Although the ubiquitins also enter the proteasome, they are not degraded (despite their protein structure) and may be used again.
Proteasomes are large multi-subunit protease complexes, localized in the nucleus and cytosol, which selectively degrade intracellular proteins. Proteasomes play a major role in the degradation of many proteins that are involved in cell cycling, proliferation, and apoptosis. The ubiquitin-proteasome pathway is well documented. Intracellular proteolysis is the most recently discovered regulatory system of cellular physiology. Everything from cell division, development, and differentiation to cellular senescence has a proteolytic component. There is no simpler way to stop a physiological process than to destroy one of the components of a pathway in a controlled fashion. The discovery of the role of ubiquitin in the proteolytic pathway earned Aaron Ciechanover, Avram Hershko, and Irwin Rose the 2004 Nobel Prize in Chemistry. Several books have become available that further reveal the importance of ubiquitins in human biology and human disease control, some of which are referenced in (15).
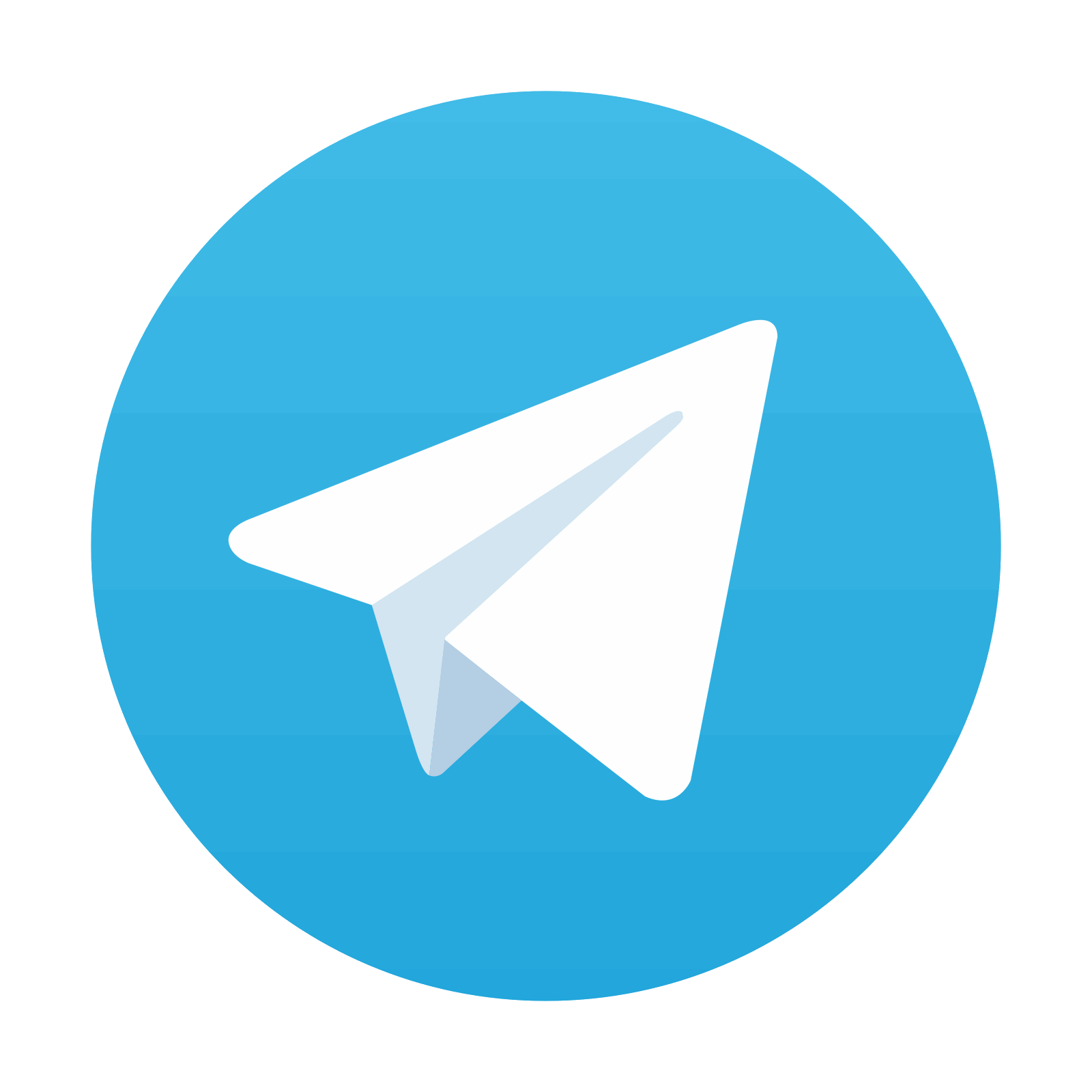
Stay updated, free articles. Join our Telegram channel

Full access? Get Clinical Tree
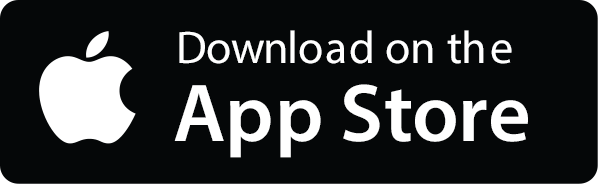
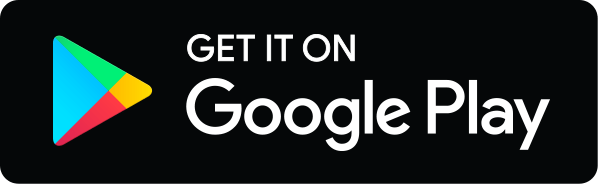