html xmlns=”http://www.w3.org/1999/xhtml”>
CHAPTER 54
Pyodermas and Toxin-mediated Syndromes
Pathophysiology
Epidemiology
Localized cutaneous staphylococcal and streptococcal infections: pyodermas
Toxin-mediated staphylococcal and streptococcal disease
Pathophysiology
Streptococci
Group A streptococcus (GAS) is a common infectious agent in children, having a large number of virulence factors responsible for a broad range of disease. It is a Gram-positive organism that is seen in chains on Gram stain. On blood agar, GAS displays characteristic β-haemolysis due to the haemolysin streptolysin S. The GAS has numerous surface and extracellular factors that confer virulence, of which the cell surface M protein is the main antigenic determinant. It aids in adherence but most importantly enables the bacterium to evade phagocytosis. GAS classification is based on genotyping of the M protein (emm sequence typing). There are currently approximately 180 emm sequence types and 800 emm subtypes described, but new types and subtypes are still being identified [1].
Invasive GAS disease is defined by the isolation of GAS from a normally sterile body site, and includes entities such as streptococcal toxic shock syndrome (TSS) and necrotizing fasciitis [2]. These infections depend in large part on the ability of the bacteria to produce streptococcal exotoxins, which are particularly potent virulence factors because they function as superantigens. Unlike traditional antigens, superantigens have the ability to stimulate immune cells without undergoing antigen processing and presentation by antigen-presenting cells (APCs) [3]. Conventional antigens are processed within the APC, and protein fragments of the antigen are then expressed on the cell surface in the groove of the major histocompatibility type II complex (MHCII). The antigen-MHCII complex then interacts with the T cell receptor in a very specific, antigen-restricted fashion. The segment of T cells bearing the receptor that corresponds to the antigen is then activated, with resultant cytokine production and specific immune activation.
Superantigens, however, are able to bypass many elements of the typical immune response sequence. Superantigens are not processed by APCs but bind directly to the MHCII complex outside the groove and therefore are able to interact with T cells in a relatively non-specific fashion. Whereas conventional antigens require recognition of all five elements of the T cell receptor (Va, Ja, Vb, Db, Jb), the recognition sequence for superantigens is almost entirely dependent on Vb only. Because only a limited amount of Vb genes exists, a given superantigen–T cell interaction may lead to the activation of 5–30% of the entire T cell population, whereas conventional antigens activate approximately 0.01–0.1% of the body’s T cells. This large-scale activation of T cells by superantigens leads to massive cytokine production, especially that of tumour necrosis factor-α (TNF-α), interleukin-1 (IL-1), and IL-6. These cytokines, especially TNF-α and IL-1, have been shown to mediate clinical effects such as fever, erythematous rash, emesis, hypotension, tissue injury and shock. Certain superantigen-mediated illnesses appear to induce a large-scale depletion of particular Vb subsets; this may result from apoptosis of initially activated T cells [4].
Host antibacterial and antitoxic immunity to GAS is an important aspect in the pathogenesis of cutaneous and systemic infections. Antibacterial immunity is related to the type-specific M component of GAS. For example, infection with type 4 Streptococcus is followed by development of specific neutralizing antibody for M type 4-bearing organisms, but not against heterologous types. Antitoxic immunity follows exposure to erythrogenic toxins such as the streptococcal pyrogenic exotoxins (SPEs). Most commonly, these toxins produce the exanthem and constitutional manifestations of scarlet fever; rarely they may cause TSS. A child with antibacterial immunity to a strain with a particular M type will not develop clinical disease. Conversely, in the absence of M-type specific immunity, an infected child will develop scarlet fever if he or she lacks immunity to the specific erythrogenic toxin. Furthermore, failure to produce an adequate humoral immunity to toxins can lead to recurrent toxin-mediated disease.
The immune response to various streptococcal exoproducts has been used in several diagnostic serological tests, most notably the response to streptolysin O. The antistreptolysin O (ASO) titer is generally elevated in patients recovering from a recent streptococcal infection. This test may be useful in certain settings to confirm or support a diagnosis. In addition, there are two important late sequelae of GAS infections that appear 1–3 weeks later: glomerulonephritis and rheumatic fever. The pathogenesis remains poorly understood, and appears to be best explained by an abnormal immune response or hypersensitivity to streptococcal antigens.
Staphylococci
Staphylococci are Gram-positive cocci found in clusters on Gram stain. Staphylococcus aureus is usually a transient pathogenic organism on the skin, and can be found to asymptomatically colonize the nasal mucosa. In certain situations, however, Staph. aureus can cause skin or invasive infections via expression of a wide array of virulence factors, including wall teichoic acid (TA) and surface proteins. These promote adherence to damaged tissue and diminish neutrophil function and immune response. Staph. aureus also secretes exotoxins and enzymes that can contribute to a variety of cutaneous and systemic infections, including four haemolysins (a-, b-, d- and g-toxins). Staphylococcal exfoliative toxins (ETs), including ETA, ETB, and ETD, disrupt desmoglein 1, a desmosomal cadherin expressed in the upper epidermis. This results in staphylococcal scalded skin syndrome (SSSS) and bullous impetigo [5]. TSST-1, among other toxins produced by Staph. aureus, can also cause TSS, an acute life-threatening illness.
Methicillin-Resistant Staphylococcus Aureus (MRSA)
In 1942, the first penicillin-resistant Staph. aureus isolate was observed, and in 1961, Staph. aureus developed methicillin resistance due to the acquisition of the mecA gene. It has been suggested that MRSA originated in vivo through the horizontal transfer of the mecA gene between two staphylococcal species. During the last 45 years, various hospital-associated MRSA (HA-MRSA) clones have disseminated worldwide. In addition, community-associated MRSA (CA-MRSA) clones have also become much more prevalent [6].
Methicillin resistance occurs by the presence of the mecA gene, which encodes penicillin-binding protein 2a. Penicillin-binding protein is a transpeptidase that restores cell wall biosynthesis of MRSA in the presence of β-lactams. The mecA gene is carried on the staphylococcal methicillin resistance gene cassette, or staphylococcal cassette chromosome mec (SCCmec). CA-MRSA and HA-MRSA have different types of cassettes and antibiotic resistance. CA-MRSA typically has SCCmec type IV, and is generally susceptible to most non-β-lactam antibiotics. In contrast, HA-MRSA usually carries SCCmec types I, II or III, and is resistant to aminoglycosides, clindamycin and macrolides.
A recently cloned strain of CA-MRSA called USA300 defies this generalization of antibiotic resistance. USA300 carries the genes that encode resistance to macrolides, lincosamides, streptogranin B, tetracycline, doxycycline and mupirocin [7]. The USA300 strain has predominated in outbreaks throughout the USA, suggesting that it possesses virulence or transmissibility factors that confer unusual pathogenicity. Recent sequencing of the complete genome of this strain identified a mobile genetic element, termed arginine catabolic mobile element (ACME). It has been hypothesized that the products of this gene cluster enhance the capacity of USA300 strains to survive at low pH on human skin and within phagocytic cells [8].
Other toxins produced by MRSA also contribute to the pathogenesis of skin and soft tissue infections. Panton-Valentine leukocidin (PVL) is a cytotoxin of CA-MRSA involved in the development of abscesses, cellulitis and furuncles. PVL lyses leucocytes by facilitating calcium channel opening and pore formation, and increases IL-8 secretion which leads to an inflammatory response and tissue necrosis. The genes that encode PVL have been found in almost all CA-MRSA isolates [7].
Risk factors for acquisition of HA-MRSA include a history of hospitalization, surgery, dialysis or residence in a long-term care facility within 1 year before the MRSA culture date, presence of an indwelling device at the time of culture, or a previous history of MRSA infection or colonization. CA-MRSA has been defined by some as a MRSA infection with onset in the community in a patient lacking established HA-MRSA risk factors. However, it is generally not possible to determine with certainty where MRSA was initially acquired [8]. Staph. aureus can persist as a colonizer for years, leading to misclassification of the source. Indeed, some ‘community-onset’ infections may in fact be caused by hospital-acquired strains, leading to a blurring of the distinction between CA-MRSA and HA-MRSA. Nevertheless, the presence of SCCmec type IV and PVL have been useful molecular markers to identify true CA-MRSA strains.
Infections caused by CA-MRSA predominantly affect children and young adults. Direct contact with infected patients, colonized subjects or a contaminated environment is implicated in the transmission of CA-MRSA infection. Crowding and sharing of personal items appear to be important factors. Transmission has occurred through activities in which direct contact is common, such as among football players, wrestlers and military personnel [9]. Nasal colonization has also been identified as a risk factor for infection [8].
References
1 Steer A, Danchin M, Carapetis J. Group A streptococcal infections in children. J Pediatr Child Health 2007;43:209–13.
2 Martin J, Green M. Group A streptococcus. Semin Pediatr Infect Dis 2006;17:140–8.
3 Burnett A, Domachowske J. Therapeutic considerations for children with invasive group A streptococcal infections: a case series report and review of the literature. Clin Pediatr 2007;46:550–5.
4 Manders S. Toxin-mediated streptococcal and staphylococcal disease. J Am Acad Dermatol 1998;39:383–98.
5 Iwatsuki K, Yamasaki O, Morizane S, Oono T. Staphylococcal cutaneous infections: invasion, evasion, and aggression. J Dermatol Sci 2006;42:203–14.
6 Deurenberg R, Stobberingh E. The evolution of Staphylococcus aureus. Infect Genet Evol 2008;8(6):747–63.
7 Kil E, Heymann W, Weinberg J. Methicillin-resistant Staphylococcus aureus: an update for the dermatologist. Cutis 2008;81:227–32, 247–52.
8 Gorwitz R. A review of community-associated methicillin resistant Staphylococcus aureus skin and soft tissue infections. Pediatr Infect Dis J 2008;27:1–7.
9 Stryjewski M, Chambers H. Skin and soft tissue infections caused by community-acquired methicillin-resistant Staphylococcus aureus. Clin Infect Dis 2008;46:S368–77.
Epidemiology
Streptococci
In the past two decades, there has been a re-emergence of serious streptococcal infections. Surface proteins, host factors and toxin production have all contributed to the renewed virulence of these bacteria. The re-emergence of invasive GAS infections, such as necrotizing fasciitis and streptococcal TSS, has been closely linked with the renewed prevalence of GAS bearing M-1 and M-3 surface proteins. Host factors in the general population appear to be partially responsible for the re-emergence of streptococci as major pathogens, as very young or immunocompromised persons are at high risk for infection with these bacteria. However, a large percentage of patients who have serious disease are otherwise healthy. This is postulated to be due to the absence of previous exposure to these more virulent strains of bacteria, as an absence of protective antibody appears to predispose persons to infection. Bacterial toxin production has also been shown to be a highly important factor in the increased frequency of serious streptococcal and staphylococcal disease, with toxins such as streptococcal pyrogenic exotoxin-A (SPE-A) being linked with the increased prevalence of serious invasive GAS infections in the United States [1].
Around the world, there are an estimated 1.7 million new cases per year and 500,000 deaths per year from serious GAS disease. In addition, there are over 100 million cases of less serious skin infections per year. Since the 1980s, severe GAS diseases have been increasing in incidence and severity. The incidence of invasive GAS disease in most industrialized countries is between 2.5 and 3 per 100,000, and mortality rates vary between 10% and 20%. The burden of severe GAS disease is predominantly in developing countries and impoverished populations. It is estimated that more than 660,000 cases of invasive disease resulting in more than 160,000 deaths occur globally each year, most in developing countries. The peak incidence of these infections occurs in infants and the elderly [2].
Staphylococci
The incidence and prevalence of MRSA infections have also been increasing. Over the last 20 years, there have been reports from many healthcare facilities documenting increasing numbers of CA-MRSA infections treated at the facility, increasing proportions of all MRSA infections that are community associated, and increasing proportions of all community-acquired Staph. aureus infections that are methicillin resistant. To date, reported CA-MRSA infections have disproportionately affected children, young adults, and people from racial or ethnic minorities and low socio-economic groups [3].
Between 1998 and 2004, 44.9% of non-intensive care unit Staph. aureus strains were methicillin resistant. Furthermore, the percentage of methicillin resistance among patients in intensive care units infected with Staph. aureus was 59.5% in 2003, an 11% increase in resistance from 1998–2002. Methicillin resistance has also spread to the outpatient setting and between 1998 and 2004, 25% of Staph. aureus infections were methicillin resistant [4].
References
1 Manders S. Toxin-mediated streptococcal and staphylococcal disease. J Am Acad Dermatol 1998;39:383–98.
2 Steer A, Danchin M, Carapetis J. Group A streptococcal infections in children. J Pediatr Child Health 2007;43:209–13.
3 Gorwitz R. A review of community-associated methicillin resistant Staphylococcus aureus skin and soft tissue infections. Pediatr Infect Dis J 2008;27:1–7.
4 Kil E, Heymann W, Weinberg J. Methicillin-resistant Staphylococcus aureus: an update for the dermatologist. Cutis 2008;81:227–32, 247–52.
Localized Cutaneous Staphylococcal and Streptococcal Infections: Pyodermas
Impetigo
Pyoderma refers to a localized purulent infection of the skin and soft tissues. Impetigo is a common type of superficial pyoderma that is characterized by inflammation and infection localized in the epidermis. Non-bullous impetigo (impetigo contagiosa) is the most common form of pyoderma and is usually due to GAS, whereas bullous impetigo is usually due to Staphylococcus aureus [1]. Non-bullous impetigo represents a host response to the infection, whereas a staphylococcal toxin causes bullous impetigo.
Impetigo most often affects children 2–5 years of age, although it can occur in any age group. Non-bullous impetigo accounts for approximately 70% of cases. Impetigo is the most common bacterial skin infection among children and is more common in those receiving dialysis. The diagnosis usually is made clinically and can be confirmed by Gram stain and culture. The infection usually heals without scarring and can resolve within several weeks even if left untreated [2].
Impetigo usually is transmitted through direct contact. In many cases, antecedent cutaneous trauma, such as insect bites or minor scratch injuries, is necessary for clinical infection. Bacterial colonization of the nares, axilla or perineum may serve as a reservoir for infection. Impetigo is more common in tropical climates and in crowded living conditions, and can be associated with poor hygiene. Certain dermatoses, most notably atopic dermatitis, are associated with Staph. aureus colonization rates as high as 90%, leading to frequent bacterial superinfection. Epidemic impetigo may also occur in the newborn nursery setting.
Non-bullous impetigo begins as an erythematous macule or papule, which rapidly evolves into a vesicle or pustule and then possibly an erosion. Serous and purulent drainage forms a characteristic honey-coloured crust. Individual lesions can enlarge to 1 or 2 cm, but satellite lesions typically appear in the vicinity due to spread by autoinoculation. Coalescence of these lesions may produce wider areas of crusted involvement. The face is the usual location, especially around the mouth and nares, but extremity lesions as well as buttock involvement are not uncommon. Although typically localized, widespread impetigo may occur, especially in the setting of underlying atopic dermatitis. Itching and mild discomfort may occur with impetigo, but systemic complaints are rare.
Bullous impetigo is mainly caused by toxin-producing phage group II Staph. aureus. It is a localized form of SSSS, with subcorneal epidermolysis caused by one of the two staphylococcal exfoliative toxins. These toxins are localized to the area of infection and Staph. aureus can be cultured from the blister contents (unlike the blisters in generalized SSSS). Superficial vesicles progress to rapidly enlarging, flaccid bullae with sharp margins. Ruptured bullae are replaced with yellow crusting. Bullous impetigo is most frequently seen in infants and favours moist, intertriginous areas, such as the nappy area, axillae and neck folds. It appears to be less contagious than non-bullous impetigo, and cases usually are sporadic.
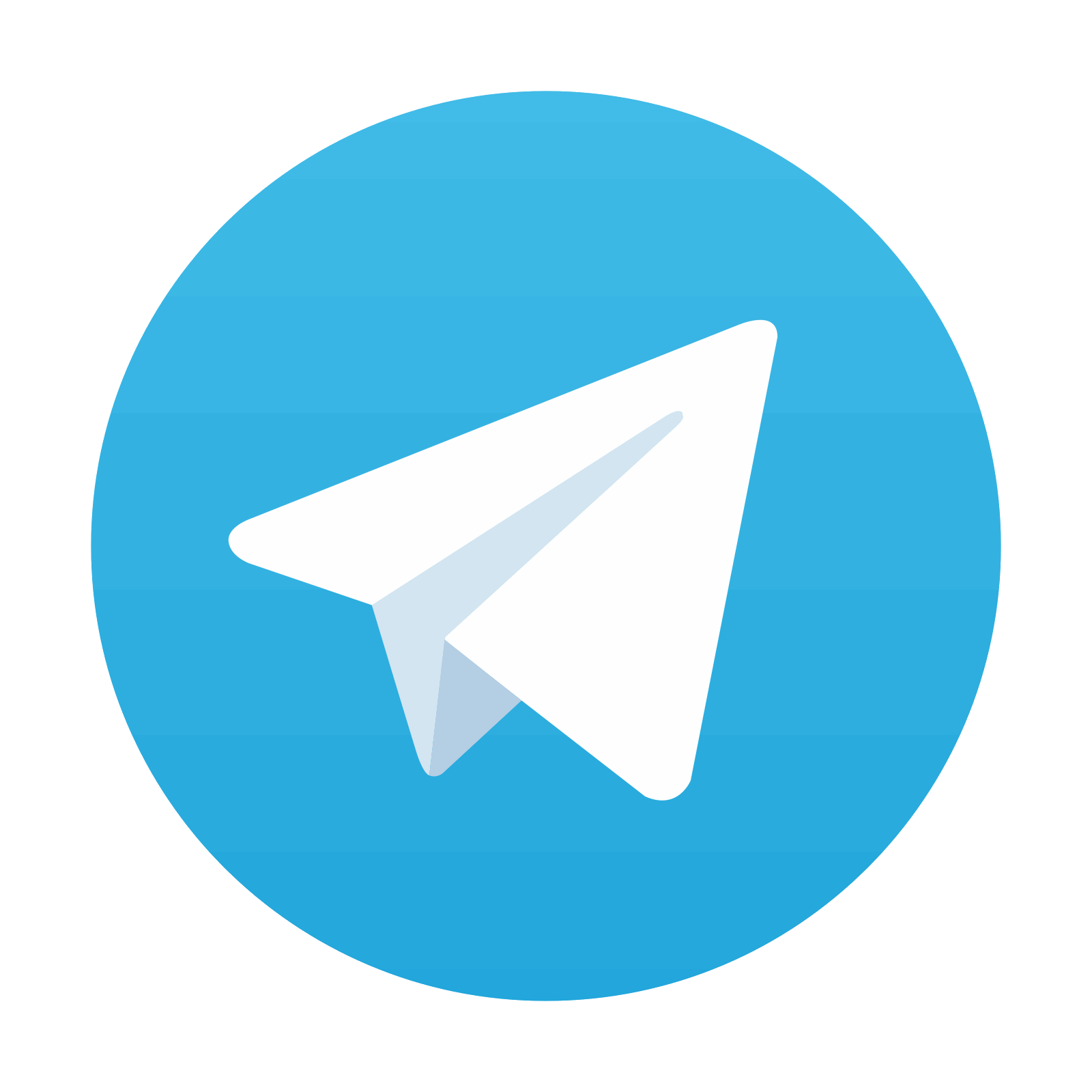
Stay updated, free articles. Join our Telegram channel

Full access? Get Clinical Tree
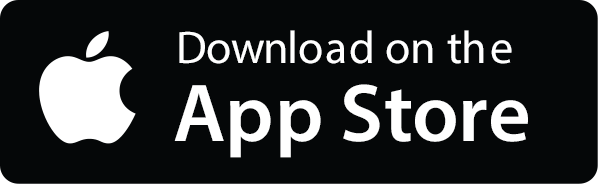
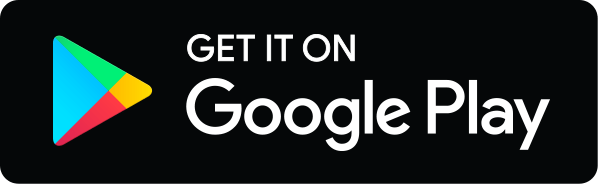