4 Synthetic and Biologic Implants
Introduction
Functional and aesthetic reconstruction of the craniofacial regions requires the manipulation of tissue to restore or enhance form and function. In the presence of tissue paucity, autologous grafts and flaps are key in the reconstructive ladder. In a broad range of cases where grafts and flaps alone are inadequate, implants are needed. In contrast to transplants, implants are manufactured devices surgically placed to replace, support, or enhance the form or function of a biologic tissue. Implants can be synthetic, biologic, or a combination of the two forms and occur either naturally or are synthesized for their intended use. The use of implants in medicine and dentistry can be traced 2,000 years back to the Romans, Chinese, and Aztecs. Over the past three decades, significant advances in nanotechnology, tissue engineering, material science, and microsurgery has broadened the clinical use and potential application of synthetic and biologic implants. Advanced manufacturing processes including rapid prototyping and additive manufacturing technology (threedimensional [3D] printing) now facilitate the inclusion of complex implants and flaps in the comprehensive reconstruction of extensive craniofacial defects. The convergence of synthetic implants, biologic implants, and 3D printing has opened the door for possible replacement of damaged tissues with manufactured whole organs. This chapter focuses on the present and evolving application of synthetic and biologic implants in facial plastic and reconstructive surgery.
Evolution of Synthetic Implants
The turn of the century marked the increased use of synthetic materials in reconstructive surgery. The earliest implants were made from readily available materials, most of which proved to be either pathogenic or toxic. With improved understanding of the immune system and tissue interaction with synthetic materials, three main generations of implants have evolved. The first generation synthetic implants were designed from materials with physical properties that matched those of the deficient tissue. These were bioinert implants that mainly replaced tissue while eliciting little or no immunologic response. The majority of these implants were made from pure metals and their alloys. Vanadium steel was the first metal alloy developed specifically for use in humans as plates for mandibular reconstruction. Vanadium steel was abandoned because of mechanical failure, corrosion, and poor biocompatibility. Currently, pure metals and alloys of iron, chromium, and titanium are the most commonly used metallic implants.
The second generation synthetic implants are bioactive implants. Bioactive implants include nonresorbable and resorbable polymers that promote implant tissue interaction and integration. Early bioactive implants manufactured in the mid-1980s such as bioactive glass, ceramic-glass composite implants, and synthetic hydroxyapatite were commonly used in head and neck reconstruction. However, problems from stress shielding and particulate wear limited their widespread acceptance. Biodegradable synthetic implants are attractive in craniofacial surgery, particularly in children. Since they dissolve over time, stress shielding and growth restriction is not an issue. The inability of second generation implants to adapt to changing physiologic demands in their microenvironment, such as weight bearing forces. remains a challenge.
Third generation implants include absorbable scaffolds such as hydrogel, designed to allow seeding with stem cells that are able to generate new bone, chondroid, vascular, or soft tissue matrix. Incorporation of nanoparticles and biologically active proteins into these synthetic implants enhance their tissue integrations, regeneration, wound healing, and overall biocompatibility.
Biocompatibility of Synthetic Implants
The biocompatibility of a synthetic implant refers to the ability of the implant to perform its intended function, with the desired degree of incorporation in the host, without eliciting any undesirable local or systemic effects. The requirements for biocompatibility are regulated by the International Organization for Standardization (ISO), which outlines guidelines for required testing for new implants. 1 Required testing includes evaluation for acute and chronic toxicity, carcinogenicity, genotoxicity, immunotoxicity, corrosion, neurotoxicity, and sensitization. Testing strategies that comply with the ISO 10993–1 are acceptable in Europe and Asia. In 1995, the U.S. Food and Drug Administration (FDA) adopted the ISO guidelines, although in some areas FDA’s testing requirements exceed those of the ISO. There are many factors that influence the biocompatibility of an implant, such as implant size, shape, material composition, and surface characteristics; host reaction to the physical characteristics of the implant material; the tissue site of implantation; and the surgical technique of placement. 2
The surface characteristics of an implant are pivotal to its biocompatibility. 3 Within seconds of implant insertion, a biolayer consisting of water, proteins, and other biomolecules from the physiological liquid is formed on the surface of the implant. Further interaction between the implant and the native tissue is mediated through this biolayer. Several methods have been used to modify the surface of synthetic implants to enhance their biocompatibility. One approach exploits the immobilization of bioactive molecules (cell adhesion peptides, albumin, fibrinogen, heparin, glycosaminoglycans) onto the implant surface by adsorption, covalent coupling, and tethering via an intermediate linker molecule. Once modified, the bulk of the implant interacts with the surrounding tissue through these bioactive molecules, thereby enhancing their compatibility. Beyond the biocompatibility of an implant, the host tissue characteristics are important in ensuring longterm success of the implant. Important tissue characteristics include vascularity, proximity to contaminated cavities, mobility of surrounding tissue, cyclic loading stress, and adequate soft tissue coverage.
Preventing Infection and Implant Failure
Sterile intraoperative handling of implants is important in minimizing synthetic implant failure. Extensive handling or exposure of the implant before insertion should be avoided. Once removed from its sterile package, an implant should be handled only by clean instruments with minimal contact with contaminated gloved hands. Implant contact with the surrounding skin or oral cavity should be minimized to decrease bacterial inoculation. Under normal circumstances, 100,000 bacteria are necessary to cause clinically significant infection; however, in the presence of synthetic material, this number is significantly reduced. 4 Antibiotic prophylaxis is prudent but should not replace the use of sterile technique. The rationale for antibiotic coverage is to prevent or eliminate any bacterial inoculation that may have occurred on the implant surface. No large clinical trials have been conducted to confirm the efficacy of this approach.
Additional antibiotic coverage is often sought by washing or soaking implants before intraoperative insertion. This practice may be more valuable with implants with hydrophilic rather than hydrophobic surfaces. Whether this antibiotic impregnation actually lowers the postoperative infection rate is unknown. Some implant surfaces favor the development and maintenance of biofilm that is resistant to antibiotic treatment. 5 , 6 , 7 , 8 Such implants ultimately require removal when infected. Surface characteristics thought to promote biofilm adherence include chemical composition of the material (e.g., Staphylococcus epidermidis often causes polymer implant infection, Staphylococcus aureus is usually found in metal implant infections), surface roughness (irregular surfaces typically promote bacterial adhesion), surface configuration (bacteria colonize porous material surfaces preferentially), and surface hydrophobicity (hydrophilic materials are more resistant to bacterial adhesion than hydrophobic materials). 5 , 6 , 7 , 8
Selected Implants and Implant Materials
Metals
Despite the great numbers of metals and alloys known to humans, remarkably few possess the minimum properties for use as implant materials. The relatively corrosive environment combined with the poor tolerance of the body to even minute concentrations of most metallic corrosion products eliminates most metallic materials from discussion. The main considerations in selecting metals and alloys for biomedical applications are biocompatibility, appropriate mechanical properties, corrosion resistance, and reasonable cost.
Gold
Gold is chemically inert and evokes minimal tissue reaction. It has poor mechanical properties in its purest form and usually functions best as an alloy when some structural integrity is needed, such as in dental implants. However, when used as a weight in upper eyelid reanimation procedures, the 24-carat, highly polished weight provides less reactivity, greater malleability, and greater success than simple tarsorrhaphy. 9 , 10
Platinum
Platinum has long been recognized for excellent biocompatibility and is the preferred implant material for gold-sensitive patients undergoing eyelid-loading surgery for lagophthalmos. 11 Platinum is a lustrous, silvery-white, malleable, and ductile metal. Compared with gold, platinum eyelid implants are denser, allowing for lower profile implants that are less noticeable ( Fig. 4.1 ). 11

Titanium
The superior strength-to-weight ratio of titanium compared with stainless steel and its outstanding corrosion resistance properties have made it the metal of choice in several implants. Light, strong, and totally biocompatible, titanium is one of few materials that naturally match the requirements for implantation in the human body. Additionally, the capacity for osseointegration makes titanium an attractive choice for bone replacement implants. The lower modulus of titanium alloys compared with steel is a positive factor in reducing bone resorption. Titanium is neither magnetic nor paramagnetic. Patients with these implants may safely undergo magnetic resonance imaging without concern about dislodgment of the graft or interference with the study. The use of customized titanium implants has seen a widespread indication for facial reconstruction following excision of orofacial tumors, in cases of severe maxillofacial trauma, and for congenital facial anomalies. Using rapid prototyping methodologies such as stereoscopic lithography or 3D printing, customized titanium implants pretested for form and fit can be produced using computer-controlled milling before surgical implantation. 12 This cuts out guess-work, minimizes operative time, and improves aesthetic outcomes. In dental restoration surgery, osseointegrated titanium roots have greatly revolutionized dental rehabilitation in post cancer patients. Osseointegrated titanium screws also form the basis of more stable fixation of prosthetic devices used for nasal, auricular, and ocular reconstruction.
Polymers
Silicone
Silicone is a polymer consisting of alternating elements of silicon and oxygen with organic side groups. It is the only form of non–carbon chain polymer in clinical use today. One form of medical grade silicone is composed of dimethylsiloxane monomers, which contain a methyl side group. Linear chains of polymethylsiloxane with lower molecular weight and viscosity form liquid silicone, which is injectable. With an intermediate degree of cross-linking and viscosity, silicone gel is obtained. When the viscosity is extremely high (centistokes = 10,000), a solid state elastomer composed of a highly cross-linked gel filled with silica particles is formed. Silastic (Dow Corning) is an example of a high-viscosity cross-linked methylsiloxane polymer, forged from silica powder to modify its mechanical properties.
Injectable medical grade silicone was first introduced by Dow Corning in 1960. By 1990, over 100,000 patients had received silicone gel injections in the face. The gel has desirable plasticity and is widely nontoxic and nonimmunogenic. Medical grade liquid silicone has been associated with numerous clinical abuses. Most abuses are related to an excessive number of injections and the use of impure silicone. Adverse reactions presenting as inflammatory nodules, or “siliconoma,” have been reported up to several years following injection. 13 Migration along the reticuloendothelial system into the regional lymph nodes, liver, and spleen has been reported. The origin of these reactions is not well understood—they have been difficult to treat and often result in disfiguring defects. However, when applied in limited quantities (0.1 mL per area) using the microdroplet technique with a 30-gauge needle, the silicone appears to be stabilized by a fibrous capsule. Interval of injection should not be less than 4 weeks. In 1991, the FDA banned the use of injectable silicone in the United States, but it is still used in a limited fashion elsewhere.
A hybrid suspension of polyvinylpyrrolidone and silicone (Bioplastique, Uroplasty BV) was introduced in 1991. It consists of particles of polymerized silicone, 100 to 600 µm in size, surrounded by a resorbable carrier gel (polyvinylpyrrolidone). The gel surrounding the silicone is engulfed within a week and replaced by collagen within 6 weeks. The silicone particles, which are too large to be phagocytized, remain in the tissue and elicit a local foreign body reaction, ending in fibrosis, which contributes to the filling effect. As with medical grade silicone, successful use of Bioplastique depends on injection technique. Placement below the dermis is crucial for obtaining a lasting result. More superficial placement may result in extrusion. Persistent induration and swelling have been described, often requiring excision because antibiotics and steroid injections have yielded a mixed and inconsistent response. Some authors have reported a curative response to the immune modulator imiquimod. 14
The solid silicone elastomer Silastic has been extensively used in head and neck reconstruction. Silastic blocks are easy to carve. It has been used in thyroplasties, cheek and chin implants, and nasal surgery. Histological studies of implants by several authors reveal that Silastic becomes enveloped by a thin organized capsule with a mild, chronic inflammatory reaction devoid of histiocytes and giant cells ( Fig. 4.2 ). Because it is highly hydrophobic, there is no bonding between Silastic and its capsule. Hence, it is more prone to becoming dislodged if not fixated and extruding when placed in a pocket with thin overlying coverage. It can also be associated with most persistent seromas. This is corroborated by the high extrusion rate of Silastic in nasal and auricular applications. 15

Polymethylmethacrylate
Polymethylmethacrylate (PMMA), an acrylic polymer of high molecular weight, was one of the first polymers used as a biomaterial. Polymerization of the methylmethacrylate monomers yields a polymer of high strength and rigidity. Because of its biocompatibility, reliability, relative ease of manipulation, and low toxicity, PMMA has been used extensively in craniofacial reconstruction. PMMA is packaged as two components: liquid and powder. The liquid contains the PMMA monomer, stabilizer, and activator. The powder contains the polymer, a radiopaque substance, and polymerization initiators. When added together and mixed, polymerization occurs. At first the cement is a relatively low viscosity glistening paste. The viscosity of the preparation steadily increases and the surface becomes dull. The rapid final phase of polymerization is associated with an exothermic reaction that can cause tissue injury. The material then becomes a solid resin.
One distinct advantage is that it can be molded in situ. The ability to mold the implant in the tissues and remove it during the exothermic phase of polymerization enables one to obtain an accurate implant in size and shape without causing thermal damage. In cranioplasty, PMMA can be reinforced with a mesh for moderate-sized defects. For very large defects, computed tomographic (CT)-guided porous custom implants can be fabricated to facilitate a more accurate reconstruction. The best indication for use of PMMA in head and neck reconstruction is in patients with good-quality soft tissue, without previous infection, and with no connection to the sinuses. It is important to ensure immobilization of this material by onlay rigid fixation with screws because it can become loose with time. 16
Polyethylene
Polyethylenes are polymers consisting of a large number of repeating monomeric units of ethylenes linked together to form highly branched macromolecules. Porous polyethylene implants are synthetic polymers that are biologically inert and nonbiodegradable in the body. MEDPOR (Porex Surgical, Inc.) is a brand of high-density polyethylene (HDPE) solid implants that have been used since 1985 in facial augmentation for reconstructive or cosmetic purposes. Its porosity allows for soft tissue and vascular ingrowth, which helps to keep the implant in place. Mesh forms of HDPE include Prolene (Ethicon, Inc.) and Marlex (Marlex Pharmaceuticals, Inc.). Porous HDPEs (MEDPOR) with pore size 100 to 150 µm, encourage osseous tissue ingrowth with mature bony ingrowth into the surface pores at 1 year ( Fig. 4.3 ). Histological analysis in an HDPE specimen revealed mature fibrous connective tissue ingrowth into pores with minimal foreign body reaction.

Cenzi et al showed that the site of implantation (i.e., nose, maxilla, and ear) and diagnosis at admission (i.e., syndromic patients previously operated) is related to a higher risk of implant failure. 17 The author’s personal experience with implants in midfacial reconstruction has shown good integration, vascular ingrowth, and good midfacial support and projection when the MEDPOR implant is completely covered on all sides with a thick and well vascularized flap. The most common site of MEDPOR exposure was around the lateral canthal region where skin coverage is thin. Recently, a porous polyethylene implant made from ultra-high molecular weight polyethylene (UHMWPE) (SynPOR, Synthes, Inc.) or a combination of UHMWPE and titanium has become available for use in anatomical reconstruction of the craniofacial skeleton. With a pore size of 150 to 250 µm, it allows tissue ingrowth instead of encapsulation ( Fig. 4.4 ).

Polyether Ether Ketone
Polyether ether ketone (PEEK) is a high-performance biomaterial that belongs to the polyaryletherketone family. The PEEKs have repeating monomers of two ether and ketone groups. PEEK is one of the highest rated thermoplastic materials in terms of heat resistance, chemical and hydrolysis resistance, resistance to the effects of ionizing radiation, high strength, and extensive biocompatibility. Implants based on the PEEK polymer have been developed in the last decade as an alternative to conventional metallic devices. PEEK has found extensive use as an alternative to titanium in expandable vertebral replacement cages in spine surgery. PEEK devices may provide several advantages over the use of conventional materials, including the lack of metal allergies, radiolucency, low artifact on magnetic resonance imaging scans, and the possibility to tailor mechanical properties. In head and neck reconstruction, it has been used as customized healing caps for dental implants and as prefabricated implants for craniomaxillofacial defects ( Fig. 4.5 ).

Expanded Polytetrafluoroethylene
Expanded polytetrafluoroethylene (ePTFE; Gore-Tex, W. L. Gore and Associates) was first introduced as a vascular graft and subsequently for hernia repair before it became popular for facial augmentation. It is composed of nodules of solid PTFE interconnected by thin, flexible PTFE fibrils. Fibrillar length determines the interspacing of the molecules and hence actual pore size and accessibility of tissue ingrowth. A pore size ranging from 5 to 30 µm allows for adequate tissue ingrowth combined with ease of removal when necessary ( Fig. 4.6 ). Current applications of ePTFE include lip enhancement; facial contouring; malar, nasal, and chin augmentation; filling soft tissue defects; and facial reanimation. 18 Complications associated with ePTFE include infection, extrusion, migration, shrinkage, and scarring. 19 To circumvent its shortcomings, various modifications of ePTFE continue to be developed. SoftForm (Collagen Corporation), a tubeshaped form of ePTFE, was developed for lip augmentation and treatment of deep facial furrows such as the nasolabial folds. Advanta (Atrium Medical Corporation) ePTFE is dualporosity architecture consisting of a soft, open porosity central core of 100 µm integrated with a smooth, medium porosity outer layer of 40 µm. Advanta’s dualporosity structure provides a softer, less palpable facial implant. 20

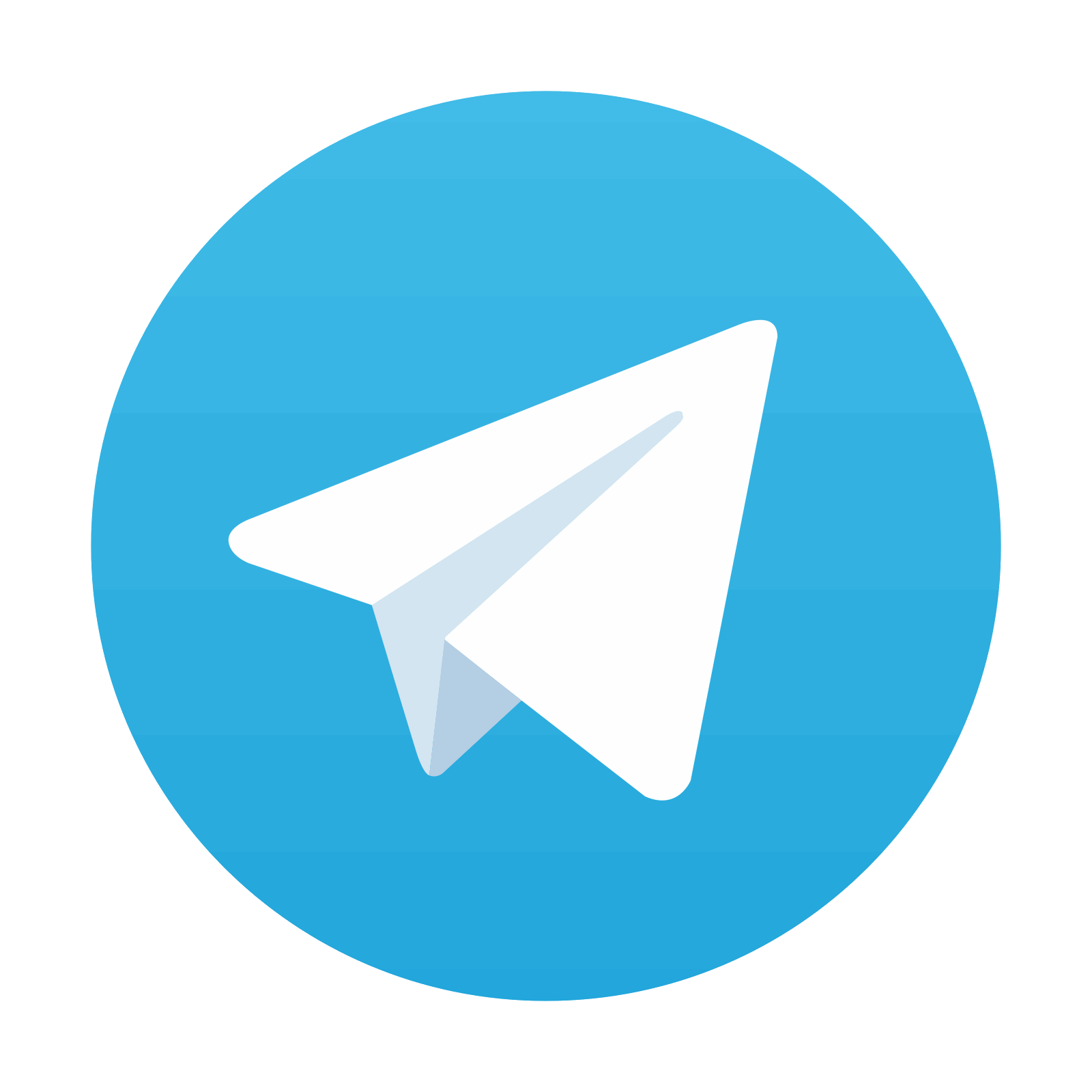
Stay updated, free articles. Join our Telegram channel

Full access? Get Clinical Tree
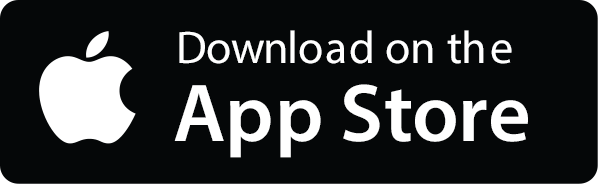
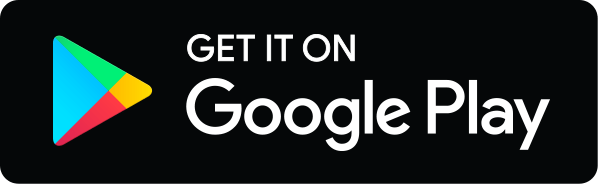
