Infrared radiation is made up of longer wavelengths (760–1060 nm) and lower energy. It constitutes 40% of the radiant energy reaching the earth’s surface, contributing to the warmth that keeps life on this planet from freezing. Although not typically associated with cutaneous photodamage, there is significant evidence that infrared radiation contributes to some of the photoageing processes [1,2].
Ultraviolet (wavelength 10–400 nm) and visible (400–760 nm) radiation occupy a central portion of the electromagnetic spectrum, between X-ray and infrared radiation (Fig. 108.1, Table 108.1). These shorter wavelengths are required in many of the important yet complicated life processes such as photosynthesis, vision and photoresponsiveness, vitamin D synthesis and general well-being. Moreover, there are relatively recent beneficial applications using UVR in the killing of pathogens, and in phototherapy and photochemotherapy. Life could not exist without this form of electromagnetic radiation. However, like other forms of radiation, too much exposure can lead to many untoward health consequences.
Table 108.1 Subdivisions and important properties of solar radiation [4]
Spectrum name | Wavelength range (nm) | Properties and health significance |
Ionizing radiation (gamma, X-ray) | <10 | Does not reach the earth’s surface; ionizes molecules; some medical applications |
Vacuum ultraviolet | 10–200 | Does not reach the earth’s surface |
Ultraviolet C (UVC, germicidal light) | 200–290 | Does not reach the earth’s surface; damages DNA, RNA, protein; generated to sterilize laboratory hoods, surgical areas, etc. |
Ultraviolet B (UVB) | 290–320 | Does not penetrate glass; stimulates cutaneous production of vitamin D to regulate calcium metabolism |
Overexposure increases risk of skin cancer, photosensitive diseases and photoageing | ||
Ultraviolet A (UVA, black light) | 320–400 | Overexposure increases risk of skin cancer, photosensitive diseases and photoageing |
Less intense but more penetrating than UVB, e.g. through the atmosphere, glass and skin | ||
Visible light: violet | 400–470 | May improve acne through destruction of Propionibacterium acnes |
Visible light: blue | 470–530 | Reduces neonatal jaundice through isomerization of bilirubin; used to treat actinic keratosis with photodynamic therapy; used to treat seasonal affective disorder |
Visible light: green | 530–600 | Used in lasers to treat vascular birthmarks |
Visible light: yellow | 600–700 | Wavelengths in yellow and red range sometimes used to treat rosacea |
Visible light: red | 700–760 | |
Infrared | 760–106 | Helps maintain the Earth’s temperature; may contribute to photoageing; repeated exposure causes erythema ab igne |
Radar, TV and radio waves | >106 |
Properties of Ultraviolet Radiation [3]
The subdivisions in the solar radiation spectrum were created on the basis of the biological significance of their properties (see Table 108.1). Radiation with wavelengths below 10 nm will ionize molecules and thus is called ionizing radiation (e.g. γ-rays and X-rays).
The wavelengths below 200 nm (10–200 nm) are known as vacuum UV light. This form of radiation does not reach the Earth’s surface and therefore does not contribute to biological processes.
The high-energy and short wavelengths between 200 and 290 nm are referred to as UVC or ‘germicidal radiation’. This type of radiation can be lethal to organisms because the wavelengths are absorbed by DNA, RNA and proteins of cells. Owing to this sterilizing effect, UVC is used in germicidal lamps, which emit 254 nm electromagnetic radiation. These lamps are used in hospital operating rooms, tissue culture hoods and experimental studies.
A division was made at 290 nm between UVC and UVB because solar wavelengths shorter than 290 nm cannot reach the Earth’s surface. The stratospheric ozone layer, a protective gaseous shield enveloping the Earth, effectively absorbs all UV radiation below this wavelength. The significant characteristic of the UVB (or mid-UVR) spectrum (290–320 nm) is that the bulk of harm to human health results from these wavelengths. The shorter the UVB wavelength, the greater is this potential.
The longer wavelengths around 320 nm mark the division between UVB and UVA. UVA (320–400 nm) or long-wave UVR is often referred to as ‘black light’ because it causes certain substances to emit visible fluorescence. It is also invisible to the human eye. Although UVA is approximately 1000 times weaker than UVB, it reaches the Earth’s surface in an amount roughly 100-fold greater than UVB. It was proposed to divide UVA into UVA1 (340–400 nm) and UVA2 (320–340 nm) because the shorter wavelengths of the latter band are much more damaging to unsensitized skin [3]. It is important to remember that there is tremendous variation in the biological effects, even within these arbitrary subdivisions.
References
1 O’Brien JP. A concept of diffuse actinic arteritis. The role of actinic damage to elastin in ‘age change’ and arteritis of the temporal artery and in polymyalgia rheumatica. Br J Dermatol 1978;98:1–13.
2 Kligman LH. Intensification of ultraviolet-induced dermal damage by infrared radiation. Arch Dermatol Res 1982;272:229–38.
3 Regan JD, Parrish JA (eds). The Science of Photomedicine. New York: Plenum Press, 1982.
Sources of ultraviolet radiation
Solar Radiation
Solar radiation is our primary source of UV light, which accounts for less than 9% of the total solar energy output. The amount of radiation that reaches our biosphere varies with time and location. Some of the variation is predictable and due to well-determined factors such as the sun’s position in the sky and the annual change in the Earth–sun distance. Geographical factors also play a role, as measurements in higher altitudes have detected shorter wavelengths up to 285 nm, whereas at sea level nothing shorter than 290 nm penetrates the atmosphere [1].
Other UVR variations are due to changes in atmospheric constituents that affect the transmission of radiation from the top of the atmosphere to the Earth’s surface: ozone, clouds and aerosols are the most important among these. These constituents exhibit seasonal and geographical patterns, as well as day-to-day fluctuations that are mostly unpredictable. A possibility of utmost concern is that these atmospheric constituents may be changing over the years and decades as a result of human activity. In particular, the introduction of man-made chlorofluorocarbons (CFCs) and nitric oxides into the atmosphere has resulted in significant localized and global depletion in atmospheric ozone since the late 1970s [2,3].
The ozone layer absorbs around 90% of UVR under 304 nm, thus attenuating the more biologically active and harmful, shorter wavelengths [4].This process is extremely important for the maintenance of health in plants and animals. Ozone (O3) is formed by photochemical processes in the upper atmosphere, known as the stratosphere, located 15–35 km above the Earth’s surface. The amount of ozone in this atmospheric layer is set by a dynamic balance of generation and degradation. More recently, scientific evidence suggests that there are catalytic losses of ozone through complicated photochemical reactions with pollutants such as the CFCs (e.g. freons in refrigeration devices and aerosol propellants).
As the transmission of solar UVB through the atmosphere varies exponentially with ozone concentration, small depletions in the ozone layer may result in hazardous increases in UV irradiance at the Earth’s surface. Increases in surface sunburning UV radiation relative to the values in the 1970s are estimated to be about 7% at northern hemisphere mid-latitudes in winter/spring and about 4% in summer/autumn; about 6% at southern hemisphere mid-latitudes on a year-round basis; about 130% in the Antarctic in spring; and about 22% in the Arctic in spring [3].
It has been estimated that a 1% decrease in atmospheric ozone is expected to increase the incidence of non-melanoma skin cancer by 2.7% [5]. Depletion of the ozone layer is a major public health problem and should concern all the populations of the world.
Solar irradiance at the Earth’s surface is affected by several other factors besides ozone. Clouds can decrease irradiance by 10–80%, depending upon the cloud density [6]. UV light can also be reflected from surfaces such as snow, sand, concrete, water and metallic surfaces. Up to 80% of incident UV light can be scattered or reflected from these surfaces. Another factor affecting UV doses is the elevation of the Earth’s surface above sea level. There will be a radiation increase of about 5–8% per kilometre increase in altitude. All these factors must be kept in mind when assessing an individual’s needs for photoprotection as they all contribute to the determination of daily UV exposures.
Artificial Ultraviolet Radiation Sources
Although the sun is the main source of environmental UVR, artificial UVR sources are becoming important in other ways. Specifically, fluorescent lamps are used in various capacities such as phototherapy and photochemotherapy for psoriasis, vitiligo and other skin diseases, including atopic dermatitis. The radiation spectrum from a fluorescent lamp depends on the lamp’s fluorescent or phosphorescent coating. These lamps are made of glass tubes that contain mercury, with electrodes at either end. Once the mercury is photoexcited by current passing through the tube, the energy is then absorbed and re-emitted by the fluorescent coating at a longer wavelength [7].
Tanning beds use fluorescent lamps that emit primarily UVA, although exact wavelengths vary across machines, with some emitting up to almost 10% UVB radiation [8]. As suntanned skin has become popular over the last several decades, a concomitant rapid growth in the tanning bed industry has occurred. In the USA, it is estimated that annual revenues from indoor tanning approximate $US 5 billion [9]. With this tanning industry growth comes recent evidence of both the acute and chronic adverse effects of tanning beds. Studies have reported an association between tanning bed use and both melanoma and non-melanoma skin cancers [10–13]. Animal studies also support this skin cancer association, including investigations that were intended to simulate tanning parlour usage [14].
Although there is some evidence supporting the argument that a UVA-induced suntan affords some protection against later UVB and UVA radiation damage [15,16], there is also evidence arguing that tissue damage is significant. One study suggests that DNA damage and/or the repair of this damage is the triggering factor in causing the tanning process [17]. Melanin production was induced in unirradiated pigment cells (in vitro) and in guinea pig skin (in vivo) when DNA fragments were added. This implies that damage must occur in order for a tan to develop. Given the controversy and the more recent evidence of earlier onset photocarcinogenesis, the use of tanning beds for cosmetic reasons should be discouraged by the medical profession.
There are several other artificial UV and visible light sources besides fluorescent lamps. These include Wood’s lamps, arc sources (e.g. mercury and xenon), incandescent sources, halide lamps and laser (Light Amplification by Stimulated Emission of Radiation). However, the need for photoprotection and public health concern regarding these sources do not approach those of fluorescent lamps or solar radiation.
Cutaneous Effects of Ultraviolet Irradiation
Exposure of normal skin to UV and visible radiation can induce many effects, some of which can be deleterious. Altering skin structure and function can lead to susceptibility for photoageing, immune dysfunction and skin malignancies.
Optical Properties
When radiation strikes the surface of the skin, some of it is directly reflected, some is absorbed and the rest is scattered and transmitted further to successive layers of cells, until the total energy has been dissipated (Fig. 108.2). The specificity of the photobiological response depends on two main factors: wavelength penetration and energy absorption. The wavelength of light to which the skin is exposed influences the type of cutaneous response.
Different wavelengths penetrate to different depths of the skin, with longer wavelengths penetrating deeper. Therefore, UVA leads to deeper cutaneous responses than does UVB. Furthermore, skin responses to radiation are initiated when energy is absorbed by endogenous or exogenous molecules, called chromophores. The skin contains multiple chromophores, although not all of them initiate photobiological responses. In the UVB range, DNA is the most important chromophore absorbing radiation. In the UVA range, protein and lipids are important endogenous absorbers, while exogenous chemicals such as certain medications (e.g. tetracyclines, sulphonamides and psoralens) often mediate photosensitizing reactions [18].
Melanin and haemoglobin represent two important chromophores for visible radiation in the skin. In addition to absorbing visible radiation, melanin also absorbs potentially damaging UVB and UVA radiation. The amount and type of melanin in the skin are major factors in reducing UVB-induced responses.
Early Effects
The early or acute effects of UVR exposure on normal human skin include vitamin D synthesis, the sunburn reaction, pigmentation, changes in skin thickness and immunological alterations.
Vitamin D Synthesis
Among all the positive health impacts of solar radiation, by far the most important is the regulation of cutaneous production of ‘vitamin’ D. The term ‘vitamin’ is a misnomer, as D is an endogenous steroid hormone, the key precursor of which is produced in the skin upon stimulation by UVB radiation. There are two forms of vitamin D: D2 (known as ergocalciferol, which is synthesized by plants) and D3 (known as cholecalciferol, which is synthesized by animals). Vitamin D3 is the main source of vitamin D in humans, and it is synthesized in the skin when UVB in the range of 290–320 nm converts 7-dehydrocholesterol into previtamin D3. In this form it is biologically inert and must be converted to 25-hydroxyvitamin D (25-OH) in the liver and then to 1,25-dihydroxyvitamin D in the kidney in order to be physiologically active [19]. This hormone is essential for calcium metabolism. Therefore, like calcium, it is crucial for proper cellular functioning throughout the body. The consequences of inadequate vitamin D are particularly severe in children, who suffer impaired bone mineralization, neurological abnormalities including seizures, muscle weakness, impaired growth and decreased immunity. In children, this constellation of symptoms is called rickets.
Rickets was first noted in large numbers during the Industrial Revolution, when people began to crowd into factories to work long hours without sunlight exposure. Many also lived in dark urban slums. By the early 1800s, rickets was attributed to lack of time outside. By the early 1900s, animal experiments identified lack of sunlight as a cause and found that both UVR and cod liver oil could prevent and treat rickets [20]. Once identified, the active component was named ‘vitamin D’ (as vitamins A, B and C had already been discovered) and it was added to various foods (especially milk) to help prevent rickets. As a result, some reconceptualized vitamin D status as primarily a nutritional issue. However, only a few foods naturally contain vitamin D, such as fish liver oils (cod), fish meat such as salmon and mackerel, egg yolks and beef liver [21].
In recent years a growing body of literature has suggested beneficial health effects of vitamin D beyond just bone health. There are studies suggesting that vitamin D may play a role in decreasing mortality and incidence in colon, breast and prostate cancers, as well as reducing risk of autoimmune diseases such as type 1 diabetes mellitus, rheumatoid arthritis, inflammatory bowel disease and systemic lupus erythematosus [22–25]. However, most of these studies are observational in nature, with concomitant biases, and some studies actually refute the correlations [26]. Specific to the field of dermatology, there are also new studies suggesting that vitamin D improves melanoma outcome [27] as well as being a major factor in the regulation of cathelicidin which plays a significant role in diseases such as atopic dermatitis and psoriasis [28,29]. Over time we will probably witness more research into this area in dermatology.
Controversy continues about the best way to simultaneously prevent vitamin D deficiency and skin cancer. Some health authorities previously recommended a purely nutritional approach, consisting of vitamin D supplementation (200 IU/day) for all infants 2 months and older, and for children and adolescents [30]. A more recent statement from the American Academy of Pediatrics recommends 400 IU/day of vitamin D for all infants (beginning in the first few days of life), and for all children and adolescents, unless they are getting this amount through ingesting at least 1 L of vitamin D-fortified infant formula or milk per day [31]. However, questions remain about the necessity, efficacy and safety of this approach compared with selectively supplementing those at risk.
Not surprisingly, the vast majority of rickets cases occur in individuals with genetically rich pigmentation, often in conjunction with other behavioural risk factors, such as cultural shrouding or minimal time outside due to sedentary lifestyles, lack of safe outdoor play space or time and long periods in childcare. Although these genetic and behavioural influences play a significant role in the risk of rickets, there are also other environmental factors such as pollution, increased cloud cover, wintertime and higher latitudes [32]. These other factors probably play a role in the realization that, while overt vitamin D deficiency such as in the form of rickets is no longer common in children, lesser degrees of vitamin D insufficiency are widespread [33]. Studies have shown prevalence of hypovitaminosis D to be as high as 78%, and breastfed infants in winter who did not receive vitamin D supplementation were the most severely vitamin D deficient [33]. These findings support recommendations for the daily supplementation of vitamin D.
Encouraging supplemental vitamin D in place of solar-triggered cutaneous production leads to some potential concerns, however. As a fat-soluble hormone, vitamin D can accumulate to toxic levels in body fat. This occurs only with exogenous supplementation, because cutaneous production is limited by a negative feedback mechanism. However, there is now a significant amount of data suggesting that concern about dietary vitamin D causing toxicity is more theoretical than real [34,35]. There are other concerns around the fact that some children will still be more at risk for vitamin D deficiency, including those with fat malabsorption problems, increased skin pigmentation or those on chronic anticonvulsant medications. These children may need even higher doses of vitamin D [36,37]. Furthermore, modest sun exposure is consistent with a healthy lifestyle and has other health benefits, which might be undermined if recommendations against sun exposure become too extreme.
Only modest sun exposure is thought necessary to produce sufficient vitamin D, e.g. only 30 min of sun exposure per week for infants dressed only in a nappy compared with 120 min per week if only the head is exposed [38]. As increasing melanization protects against solar damage to skin, it also increases the amount of UVB needed to make vitamin D. Some studies estimate that deep pigmentation increases the minimal erythemal dose 33-fold compared with type 1 skin, and correspondingly requires up to 6–10-fold increases in UVR doses to produce vitamin D [39]. In another study, a suberythemogenic exposure of 5% of the skin surface produced mean serum 25-OH vitamin D levels above the deficiency range in elderly patients [40]. More research is needed to better quantify optimal sun exposure, including how it is affected by environmental, behavioural and pigmentation factors.
Sunburn
The sunburn reaction, an inflammatory erythema, is the major initial clinical manifestation of UVR overexposure and is the result of vasodilation (Fig. 108.3). Heat also occurs because of increased blood flow in the skin. Erythema develops when the volume of blood in the superficial and deep dermal vascular plexuses increases by a mean of 38% over normal levels [41]. Other features of this response include swelling from vasopermeability, and pain and pruritus from the effects of chemical mediators on nerve endings. An individual’s skin type and pigmentation affect the amount of UVR needed to induce the sunburn response, as well as the wavelength of UV light.
Although UVB is much more erythemogenic, the large quantity of UVA in terrestrial sunlight can contribute as much as 15% of the erythemal effectiveness at the solar peak [42]. The erythema that is elicited after UVB overexposure is delayed in onset and usually appears 3–5 h later, reaching a maximum intensity between 12 and 24 h [43]. UVA exposure induces a biphasic erythematous response. The erythema is present just after irradiation, decreases around 4 h, then peaks again at a plateau between 6 and 24 h [44]. When compared with individuals less than 30 years of age, the erythema in people over the age of 60 years is less intense during the first 24 h, but it is more persistent.
There are several histological alterations that occur in the sunburn reaction. The epidermis is the cutaneous target that is most affected by UVB irradiation, whereas dermal responses are most characteristic of UVA irradiation [45]. As early as 30 min after a single sunburning exposure, damaged keratinocytes called sunburn cells are seen. These sunburn cells are considered to represent apoptotic keratinocytes that have sustained a lethal dose of UVR, which is a crucial protective mechanism against the carcinogenic effects of UVB irradiation [46]. They are more numerous in the lower half of the epidermis. Intercellular oedema (spongiosis) is a variable finding, although subepidermal oedema and blistering can commonly occur. These latter changes result from the most prominent dermal alterations involving the superficial venular plexus. Endothelial cell enlargement and perivenular oedema develop most significantly during the first 24 h after sunburn, and by 72 h the venular changes are minimal. These alterations also involve the venules of the deep reticular dermis and even the subcutaneous fat [43]. Along with the blood vessel alterations is an associated perivascular infiltrate in which the mononuclear cells exceed the number of neutrophils at all times.
Although best avoided, if sunburn does occur, several palliative options are available depending upon the severity. Oral fluids should always be encouraged, especially if blistering occurs. Oral non-steroidal anti-inflammatory agents (NSAIDs) such as aspirin or ibuprofen can alleviate some sunburn symptoms, presumably by inhibiting prostaglandin production [47]. Topical corticosteroids can also help by inhibiting leucocyte migration or cytokine production. Synergistic effects of using both oral NSAIDs and topical corticosteroids together have also been demonstrated [48]. Moreover, scientists are examining the role of topical and oral antioxidants such as vitamin E to reduce inflammatory damage following UVR overexposure [49,50]. The rationale behind antioxidant use is to lower the concentration of free radicals in UV-injured skin and presumably decrease the production of chemoattractants for leucocytes. Recent work is also highlighting the ability of green tea polyphenols to inhibit UV-induced erythema and cyclobutane pyrimidine dimer production in human skin [51]. Cyclobutane pyrimidine dimers play an essential role in skin cancer induction and immune suppression, so green tea may ultimately prove to be a photoprotective pharmacological agent.
Pigmentation
Another early effect of UV irradiation is increased melanin pigmentation of the skin. This response occurs in two distinct phases depending upon the action spectra: immediate pigment darkening and delayed tanning.
Immediate pigment darkening occurs within seconds of exposure to UVA or short-wavelength visible irradiation. Depending upon the dose, the reaction can fade within minutes (small exposures) or it can persist for many hours or days and blend into the delayed tanning response (large exposures). The darkening response results from alteration of melanin present in the skin, and is thus more conspicuous in individuals who have higher genetic (constitutive) pigmentation or induced (facultative) pigmentation. The alterations include the photo-oxidization of existing melanin and the redistribution of already formed melanosomes into the peripheral dendrites [52]. A photoprotective effect against UVB-induced sunburning has not been demonstrated for this immediate darkening response.
Delayed tanning induced by UVB irradiation first appears about 72 h after the exposure. The resultant suntan forms after a sequence of complicated biochemical events. In general, melanocyte tyrosinase activity increases, melanocytic dendrites elongate and arborize, the number and size of melanosomes increase, and accelerated melanin transfer to keratinocytes results in a significant increase in epidermal melanin granules [53]. In fair-skinned people, this response may manifest not as uniform pigmentation but as small ephelides (freckles) or even much larger ‘sunburn freckles’ or solar lentigines [54] (Fig. 108.4).
The major action spectrum for the process of delayed tanning is within the UVB range, although UVA and visible light may also cause this effect. Overall, melanogenesis may be a response to damaging UV irradiation that has evolved over time to provide a protective suntan in skin at risk of further UVR injury. Although delayed tanning affords some protection against further sunburn reactions by lowering the erythemal sensitivity, it does not protect against many of the later effects of chronic UV light exposure as seen in photodamaged skin.
Skin Thickening
The epidermis, including the stratum corneum, can thicken twofold to fourfold as a transient protective response to UVB and UVC. There is minimal epidermal thickening in UVA-induced delayed tanning. This thickening increases skin tolerance to UV-induced erythema severalfold and, following the cessation of UVR exposure, these changes gradually fade over several weeks. The thickening is due to hyperplasia and augments the lesser protective effects of tanning in pigmented skin. After a period of cellular inactivity immediately following UVR exposure, the hyperplasia results from marked acceleration of mitotic activity and the synthesis of DNA, RNA and proteins [55]. Although the mechanism of the increased epidermal mitotic activity is not fully known, UV irradiation induces a large increase in epidermal ornithine decarboxylase. This enzymatic activity often precedes tissue proliferation in many other experimental systems [56].
Immunological Alterations
There is accumulating evidence to suggest that UVR significantly affects the cutaneous immune system in both experimental animal models and humans. This fascinating discipline is known as photoimmunology.
Cutaneous immunoreactivity is one of many barrier mechanisms required to protect the skin and the body. The goal of this mechanism is to detect and kill micro-organisms that penetrate the skin and mucous membranes, as well as to detect and destroy cells involved with carcinogenesis. However, many influences of UVB, and to a lesser extent UVA, on the cutaneous immune system are suppressive and thus impair these functions.
Suppression of contact sensitivity to certain allergens such as 2,4-dinitrochlorobenzene (DNCB) has been demonstrated in normal subjects exposed to UVA and UVB radiation in a tanning solarium [57]. Moreover, DNCB contact sensitivity could not be induced on the UVB-exposed skin in 40% of healthy Caucasian volunteers, and in 90% of patients with a history of skin cancer [58]. This UV-induced local immunosuppression is probably initiated by a direct effect of UV radiation on epidermal Langerhans cells, impairing their capacity to present antigen, as well as release of soluble immunosuppressive factors (interleukin 10, tumour necrosis factor α, cis-urocanic acid) [59]. There is also UV-induced systemic immunosuppression, whereby larger doses of UVR applied to the skin lead to failure of contact sensitivity responses in distant, non-irradiated skin, probably through inhibition of Th1- and Th2-mediated immune responses [58,60].
Ultraviolet B light can also impair the resistance against skin- and non-skin-associated infections and tumours. Animal studies have found diminished resistance to infectious agents such as Candida albicans, Mycobacterium bovis, Schistosoma mansoni, Borrelia burgdorferi, Trichinella spiralis, Mycobacterium leprae and herpes simplex virus [61–66]. There is also significant evidence that photoimmunosuppression plays a role in the development of non-melanoma skin cancers [67–69] and this role may even extend to the development of cutaneous melanoma [70,71]. However, given that experimental methodologies are quite diverse and data acquired from humans are incomplete, it is still difficult to assess the clinical relevance of photoimmunosuppression in the general population.
Despite all the suppressive effects on the immune system, UV light exposure can also have beneficial immune effects as well. Reportedly, suberythemal doses of UV light increased the immunological capacity in children with frequent upper respiratory infections [72]. Furthermore, there exists epidemiological evidence to suggest that UVR may play a protective role in some autoimmune diseases, including multiple sclerosis and rheumatoid arthritis [73]. It is quite possible that UVR-induced increases in serum vitamin D levels may play a significant role in the immunomodulation of these diseases.
Late Effects
Although most of the late cutaneous effects of chronic UVR exposure are not considered to be a childhood problem, they certainly should be a childhood (and parental) concern. Given that a significant proportion of our lifetime sun exposure occurs before adulthood, attention to regular photoprotection is of the utmost importance to prevent the late effects of photodamage: photoageing and photocarcinogenesis.
Photoageing
Cutaneous photoageing describes the chronic UVR damage that is later superimposed on the chronological or intrinsic ageing process in the skin. It is typically mistaken for the inevitable ‘natural ageing’ of the skin. However, looking aged is mostly due to the environmental insults of UV light exposure, accounting for about 90% of perceived ageing changes [74]. The clinical features include fine and coarse rhytides (wrinkles), dryness, mottled pigmentation, coarseness, lentigines, telangiectasias, comedones, purpura and laxity [75]. Histologically, there is elastosis and accumulation of amorphous and degenerate elastic tissue in the upper dermis. Figure 108.5 demonstrates over-exposed skin on the dorsal forearm versus less exposed skin on the underside of the forearm.
Fig. 108.5 Over-exposed skin on the dorsal forearm versus less exposed skin on the underside of the forearm.
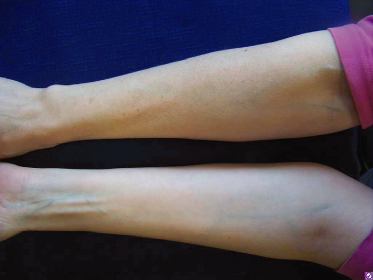
Photocarcinogenesis
The association of sunlight and the development of skin cancer was first reported by Unna [76] in 1894. Subsequently, there have been convincing correlations between the incidence of non-melanoma skin cancer and cumulative sun exposure, especially in fair-skinned individuals [77–79]. Except for lentigo maligna melanoma, the correlations for cutaneous melanoma are not as strong but seem more dependent on intermittent high-dose UVR exposures and sunburns early in life [80–83]. Mouse models have shown that a single dose of erythemal UVR to neonates, not adults, is necessary and sufficient to induce tumours similar to human melanoma [84]. On a molecular level, the tumour suppressor gene p53 is considered a critical regulator of the cell cycle as it allows either DNA repair or apoptosis. When the p53 gene has undergone mutation, p53 protein functions can be disturbed and the ability to manage DNA repair and to eliminate highly damaged cells is lost [85]. Clonal expansion of p53 mutant cells has been found in chronically sun-damaged skin as well as actinic keratoses, squamous cell carcinomas and basal cell carcinomas [85,86]. The role of p53 gene mutations in melanoma is still unclear as tumour results have been extremely variable.
UV light addiction
For years dermatologists have considered the possibility that UV light can potentially be addicting. Now there is much more evidence to support this hypothesis. High risk tanning behaviour shows signs of both dependence (physiological dependence) and addiction (psychological dependence) [87]. In a 2006 study, researchers used naltrexone, an opioid antagonist, to induce symptoms of withdrawal in frequent tanners [88]. Fifty percent of these tanners given naltrexone before UVR exposure demonstrated symptoms of withdrawal such as nausea and jitteriness. Infrequent tanners did not exhibit these symptoms, suggesting the possibility of physiological dependence.
In a different study, frequent tanners were able to distinguish between UV-emitting versus non-UV-emitting (placebo) tanning beds that appeared identical [89]. Tanners in this study (95%) demonstrated a marked preference to tan in the UV-emitting bed, and they also described a more relaxed mood in this bed, suggesting the possibility of endorphin release.
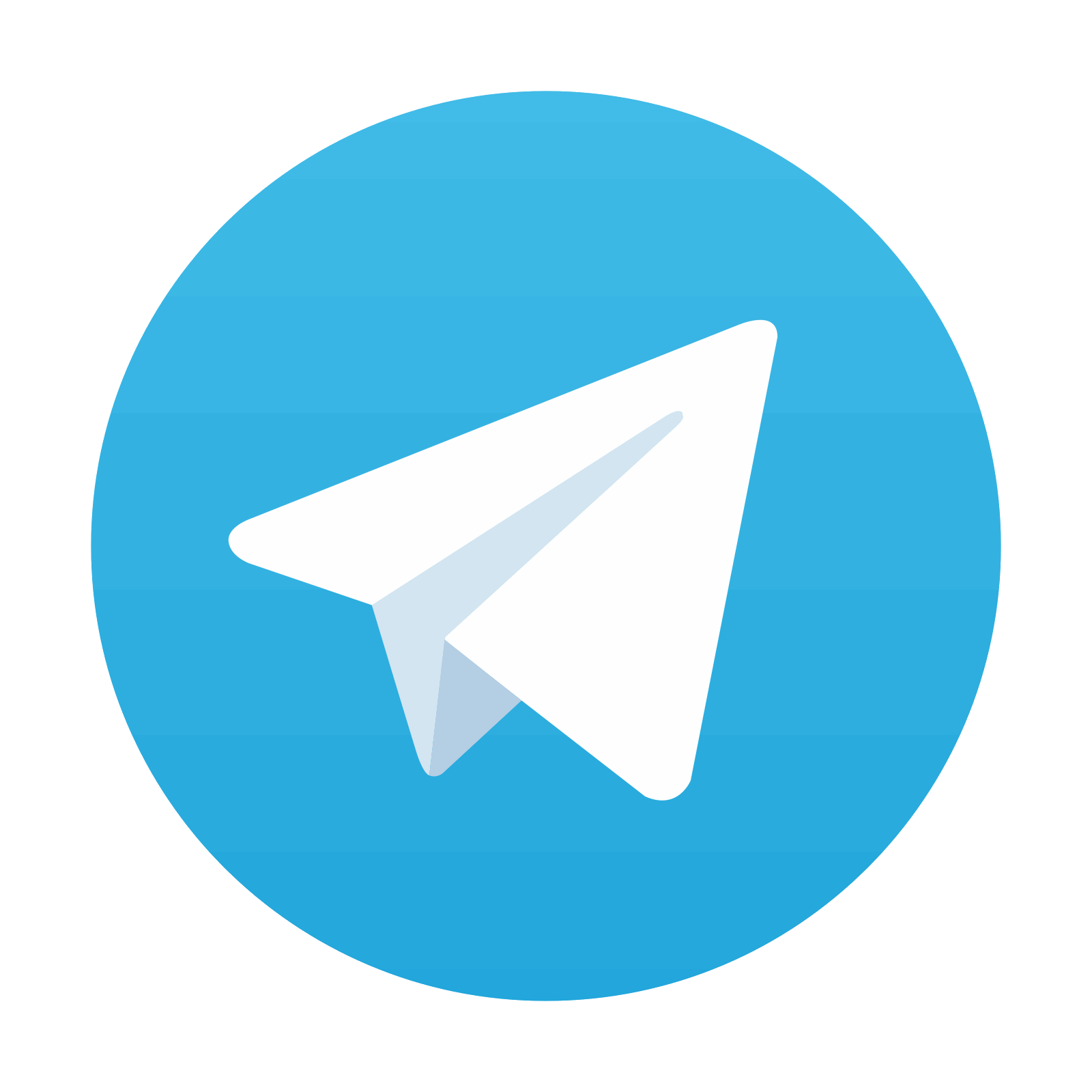
Stay updated, free articles. Join our Telegram channel

Full access? Get Clinical Tree
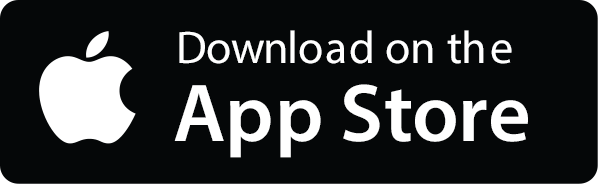
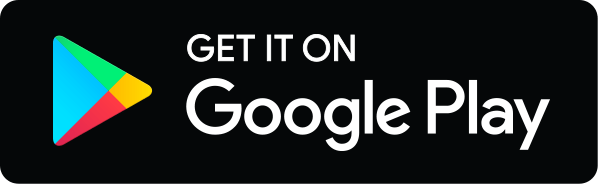