Fig. 21.1
Performance of 16 unilateral upper extremity amputees across three tests of sensory function. In each test, stimuli were applied to the perioral regions located contra- or ipsilateral to the amputation . We failed to detect any significant differences between sides in the SemmesWeinstein test of sensory thresholds, grating orientation test, or static 2-point discrimination
On the basis of these data, one might be tempted to conclude that reorganizational changes in cortical maps due to decreases in activity are functionally irrelevant. Consistent with this perspective, neurophysiological recordings and microstimulation in residual limb peripheral nerves suggest that even chronic amputees retain functional sensory [49, 52] and motor [53–56] representations of their missing hands.
Another perspective on the functional relevance of amputation-related cortical reorganization comes from attempts to determine whether it can be reversed, and to identify associated behavioral consequences.
Can Amputation-Related Cortical Reorganization Be Reversed?
This question will remain unanswerable in humans. Rightfully, we never will have the opportunity to map the brain of healthy individuals prior to their traumatic amputations. Further, there is considerable intra and interindividual variation in brain structural and functional organization . As illustrated below, this places limitations on the precision of comparing cortical representations of the affected versus unaffected hand, and between patients and healthy controls. We can, however, hope to answer a number of other important questions: Is the representation of the transplanted hand located where we would expect it to be based on normative data from carefully matched controls? Do stimulation and/or use of the hand recruit other brain regions more heavily? Is there any persistent evidence for intrusion of representations of other body parts into the normatively defined former hand territory? And, perhaps of greatest clinical importance, do any of the changes that we observe at the central level correlate with current or future recovery of function?
Nerve Repair and Cortical Reorganization
What little we know about the reversibility of reorganizational changes in the cortex comes from classic experiments of sensory nerve injury and repair in monkeys. Following axotomy and surgical repair of the fascicles, there is an initial silencing of S1 cortical neurons whose receptive fields lie below the lesion. As the nerve regenerates at approximately 2–3 mm per day [57], the S1 neurons whose receptive fields were formerly devoted to the deafferented region gradually redevelop a map. However, in adult monkeys, map topology remains disorganized and includes islands of responsive regions amongst areas of unresponsive neurons [45]. Fetal monkeys experiencing the nerve injury and repair recover what appears to be a typically organized S1 map [58], which is likely attributable to the increased capacity for experience-dependent plasticity during the period of rapid cortical development. Importantly, if the nerve is crushed rather than severed, the S1 map in adult monkeys also recovers its normal topography [10]. Similarly, adult whisker amputation alters the receptive field properties of barrel cells of the rodent sensory cortex, but appears to leave the architecture of the cortical map intact and thus recoverable once the whisker regenerates, reinstating afferent signals [59, 60]. This indicates that it is not the period of deafferentation that is problematic for sensory map recovery, but rather the reinnervation errors that occur through nerve regeneration [3]. As noted above, these errors and the resulting changes in cortical maps may account for the persistent sensory difficulties experienced by patients following adulthood nerve repairs [44, 45].
Recipients of hand replants provide an opportunity to investigate the reversibility of reorganizational changes in the human cortex, a complete loss of afferent and efferent traffic between the brain and regions distal to the injury. Although hand replantation is done within 24 h of amputation, the brains of these patients also undergo an extended period of time during which activity between the hand and brain is first absent and then diminished due to the pace of nerve regeneration. Compared with hand transplants, there may be greater potential for reinnervation errors due to the fact that the surgery involves a traumatically amputated limb. Nevertheless, the few fMRI investigations of hand replant recipients conducted indicate increased activity within M1–S1 during hand movement [61–63]. Interactions between these primary areas and higher-level motor regions may evolve along with hand function. Functional connectivity analyses of these fMRI data reveal increased contributions of premotor cortex and increased inhibition from ipsilateral M1 during movements of the affected hand, which may be compensatory and related to the increased difficulty in sensorimotor control [63].
Chronic Deafferentation and Cortical Reorganization
In comparison to replant patients, transplant recipients have typically been without their hand(s) for years, or even decades, before transplanted. Despite substantial variation among cases and the small number of published functional neuroimaging studies, it appears that volitional movement can elicit increased activity in the contralateral primary sensorimotor cortex of allogeneic transplant recipients [64–66]. It is important, however, to recognize that in most (if not all) cases, these tasks involved use of forearm flexors and extensors, which were not transplanted. This fact constrains what should be concluded from these data with regard to the reversal of amputation-related cortical reorganization. It does seem reasonable to expect that forearm muscles would be considerably less active following distal hand loss, and this could certainly contribute to changes in activity-dependent representations. However, it would be a mistake to treat these changes as reflecting the emergence of cortical representations of transplanted muscles.
To address the cortical representation of transplanted muscles one would need a task that isolates movements of intrinsic hand muscles (e.g., finger abduction–adduction). In practice, however, this is challenging because few patients appear to develop volitional control of muscles intrinsic to the transplanted hand. When this does develop, it appears to occur late in the recovery process [67]. An alternative is to determine whether TMS of M1 can elicit motor-evoked potentials (MEPs) from transplanted muscles. This approach was applied successfully to two double-hand transplant recipients and demonstrated substantial interindividual variation, as well as significant differences within individuals between the time to re-establish M1 muscle representations on the left and right sides [68]. Because TMS is a means of artificially stimulating M1, the question remains as to when (or if) these representations will become relevant to hand control.
Whether or not cortical responses arise from transplanted tissue is a persistent issue that plagues motor (really “sensorimotor” due to the afferent feedback that accompanies movement) studies of transplants. This challenge would seem to be overcome in studies employing localized sensory stimuli. In the first such published investigation, brushing of the transplanted insensate palm with a coarse sponge increased activity within the contralateral postcentral (putative S1) and precentral (putative M1) gyri a mere 4 days postsurgery [69]. As appreciated by the authors, there is no known physiological mechanism that can account for such rapid recovery. What could produce these data? My suspicion is that this seemingly impossible result most likely arises from the inadvertent induction of neuromuscular activity in the flexors and extensors of the residual forelimb during application of the tactile stimulus. A similar criticism can be levied against our earlier work on sensory recovery 4 months posttransplant in a right hand recipient. In this case, however, the patient had regained sensitivity in the thenar eminence and wrist [70]. It is clear that more well-controlled systems for the delivery of sensory stimuli during fMRI are required. Along these lines, we have developed a computer-controlled, pneumatic system to deliver cutaneous sensory stimuli to any location of the body including the individual fingers and the face [71]. Some preliminary findings from this technique are presented below.
Recruitment of the Normatively Defined Former Hand Territory
While it may be impossible to ever determine whether amputation-related reorganizational changes can be fully reversed, progress is being made on answering a number of other important, clinically relevant questions. Is the representation of the transplanted hand located in the location we would expect based on normative data from carefully matched controls? Do stimulation and/or use of the hand recruit other brain regions more heavily? Is there any persisting evidence for intrusion of representations of other body parts into the normatively defined former hand territory? And, perhaps of greatest clinical importance, do any of the changes that we observe at the central level correlate with current or future recovery of function?
Along with our collaborators at the Christine M. Kleinert Institute, we have developed a strategy to address what happens to the former hand territory after amputation and after transplantation. A distinguishing feature of our approach is that fMRI data from a control group matched to patients on the basis of age, sex, and hand-dominance is used to functionally define the regions of cortex responsive to sensory and/or motor stimulation of the hands (i.e, the normative hand representations) in a standardized space (Fig. 21.2 [72]). Then, using nonlinear techniques, we can warp the coregistered structural and functional brain images of our patients into this template space and undertake quantitative comparisons of activity within the probabilistically defined former hand territory during various manipulations. By way of illustration, consider DS, who was a unilateral right hand amputee for 35 years before receiving his transplant 4 months prior to our testing. His level of hand functional recovery at this early stage was limited (Carroll score = 57, disabilities of the arm, shoulder, and hand (DASH) score = 12.5). Sensory threshold testing revealed a localized patch of low normal sensitivity on the thenar eminence, while the rest of the hand remained insensitive at this early stage. Because we did not test this individual prior to his transplant, the amputated state was estimated based on data from a cohort of unilateral, below elbow, pretransplant amputees (details can be found in [70]).
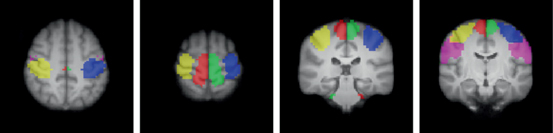
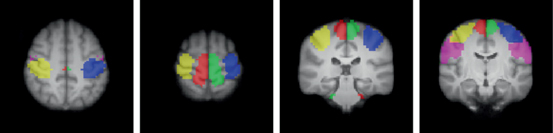
Fig. 21.2
Functionally defined normative sensorimotor representations based on fMRI data from 17 healthy, right-handed adults. Blue = right hand, yellow = left hand, green = right foot, red = left foot, violet = lips
Together with the research introduced earlier, these findings are consistent with two working hypotheses. First, areas of the sensory and motor cortex devoted to representing the hand prior to amputation come to represent the transplanted hand. Volitional finger extension–flexion movements of the transplanted hand increased activity within the contralateral primary sensorimotor areas and specifically within the normatively defined former sensorimotor hand territory (Fig. 21.3).
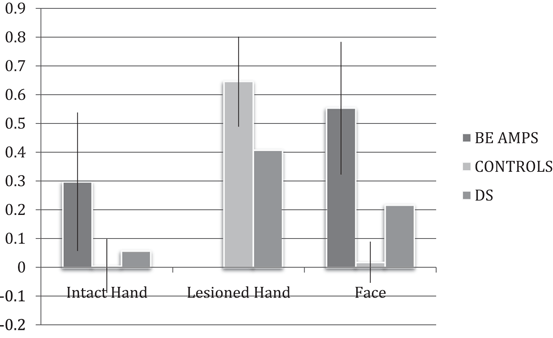
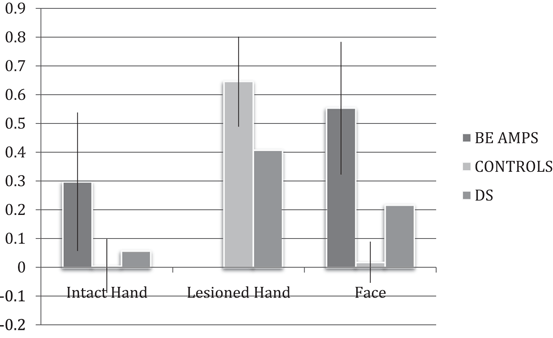
Fig. 21.3
Percent change in the fMRI signal relative to rest within the normatively defined former hand sensorimotor hand territory. Finger flexion–extension of DS’s transplanted right hand 4 months postsurgery are associated with increased activation within the former hand territory, albeit below what is detected on an average in the group of matched controls. Like the below-elbow (BE) amputee group mean, DS shows a tendency for increased activity within the former hand territory during movements of the face, which is not detected in healthy controls. Error bars represent 95 % confidence intervals around group means [70]
Second, changes in cortical organization associated with unilateral hand amputation may not be fully reversed. Like the pretransplant amputees, DS shows some evidence for persistent intrahemispheric reorganization. Movements of the face are associated with increased activity within the former sensorimotor hand territory (Fig. 21.3). This is likely due to an expansion of the somatotopically adjacent face representation into the former hand territory following limb loss. The critical question is whether these effects relate to the recovery of hand function, and if so how. One possibility is that complete dissipation of responses within the hand territory to facial movements will require experience-dependent changes driven by extended increased use of the hand; an idea to which I return in the final section.
As alluded to earlier, we have also been developing techniques that use high-resolution fMRI and a novel, computer-controlled, sensory stimulation system to assess details of the S1 hand representation in healthy adults, as well as hand replant and transplant recipients (Fig. 21.4; see details in [71]). This system makes use of a bank of computer-controlled switches to deliver trains of pulsed, compressed air to specific targets on the body. I have illustrated one system for delivery of cutaneous stimulation to the digit tips (Panel A). A short fMRI localizer is first acquired to identify regions that show increased activity during simple movements of the hands and face. This data is then used to strategically position 15 (1.5 mm in-plane resolution) slices for optimal coverage of the primary and secondary somatosensory cortices (Panel B). This allows us to identify peak responses to individual digit stimulation located within the putative area 3b along the rostral bank of the central sulcus, defined based on probabilistic atlas derived from postmortem analyses of human brain micro-architecture [73]. At this level of resolution, there is considerable individual variation in the structural and functional organizational details of the cortex. For this reason, while it is feasible to determine whether responses fall within the likely former hand territory of area 3b, defining the precise locations of normative digit representations through current noninvasive imaging techniques is impossible. Details of findings from patients will appear in an upcoming publication.
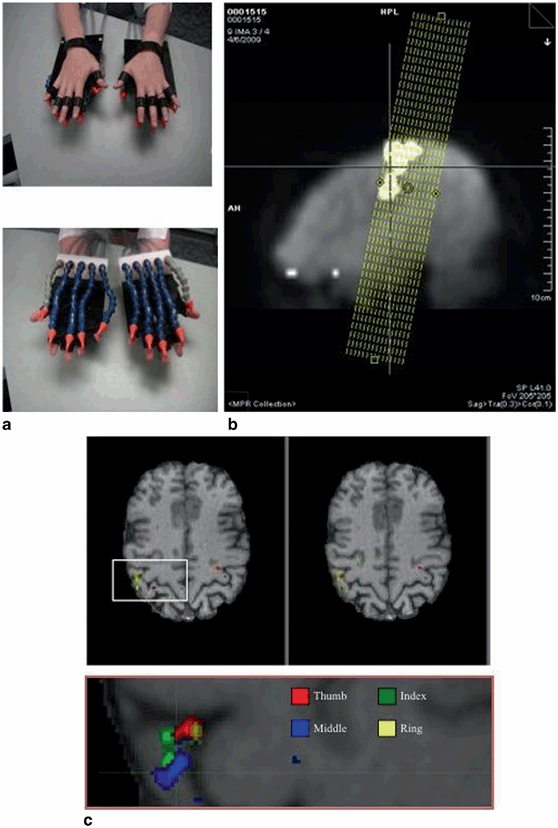
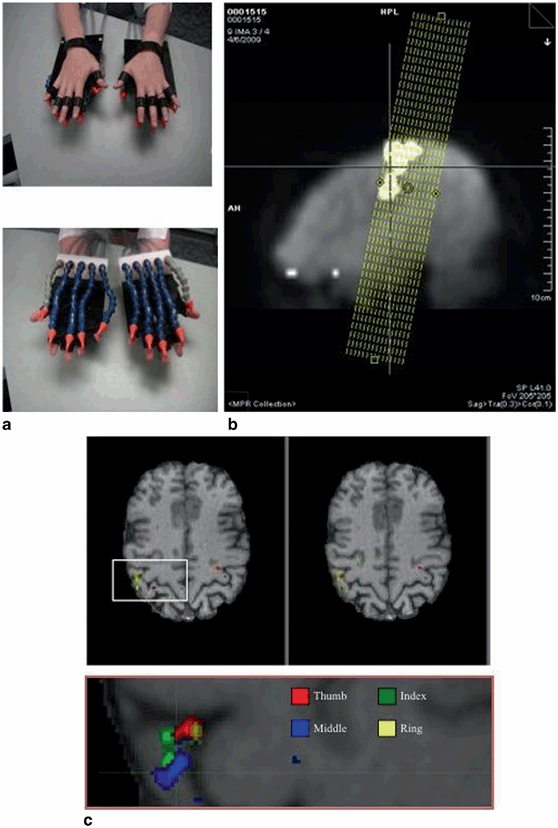
Fig. 21.4
High-resolution mapping of digit representations in S1 area 3b. a MRI-compatible pneumatic stimulators that deliver air pulses to the individual digit tips. b Positioning of MRI slices based on use of a sensorimotor localizer. Fifteen slices are positioned to allow coverage of primary and secondary somatosensory cortices defined functionally for each individual [71]. MRI magnetic resonance imaging
To date, these neuroimaging investigations of hand transplant recipients have focused exclusively on simple repetitive movements or passive sensory stimulation. If the goal is to understand clinical relevance, however, then we need to move toward evaluations that address mechanisms underlying functional use of the hand. Doing so during fMRI is challenging for many reasons including the small space, limtations of magnetic resonance (MR)-compatible hardware for stimulus delivery, and the considerable potential to induce artifacts through movements of the head. Nevertheless, it is possible to study mechanisms involved in goal-directed actions such as manual reaching and grasping that involve not only primary sensorimotor regions but also parietal, premotor, and a number of subcortical structures [74–76]. This provides an opportunity to understand how these structures respond to changes in afferent and efferent activity, and determine any compensatory roles that may play in recovery of function. Our team is presently engaged in a project designed to address these very issues in both hand transplant and replant recipients.
Reconciling Reorganization, and Recovery: A Working Model
On the one hand, I have reviewed some of the large body of evidence that loss of a limb perpetuates experience-dependent changes in the organization of the central nervous system, and that these effects are especially pronounced in the activity-dependent maps of the cerebral cortex. These changes appear to involve multiple mechanisms operating over time scales ranging from hours to years. On the other hand, I drew on the available, albeit limited, data that showing that a hand transplanted years after amputation comes to be represented within the former S1 and M1 hand territories. Do these findings suggest that the changes seen postamputation are functionally irrelevant and epiphenomenal? I believe that the answer is no, and that these results instead suggest the existence of two stages of recovery with distinct mechanisms and time courses.
A key mechanism underlying the changes in cortical maps that occur following amputation is the dynamic functional rebalancing of inhibitory and excitatory synapses after deafferentation [14]. It has been suggested that these functional changes occur while leaving the original pattern of cortical connectivity structurally intact [52, 77]. If this is correct, then one might expect that re-establishing afferent and efferent activity between the brain and hand may reverse these changes, returning the cortex to a state of functional organization similar to what was in place prior to the injury [70]. A parallel might be drawn here with the evidence mentioned earlier showing that the S1 hand map returns to its original state of organization as monkeys recover from a crushed nerve [10]. Put differently, the cortex may maintain a coarse-level, latent somatotopy even years after limb loss that may account for gross recovery of the cortical somatotopy (e.g., responses within the former hand territory during sensory stimulation or active use of the transplanted hand). These changes should emerge in synchrony with peripheral nerve regeneration and be fully completed within the same time frame.
This initial stage may explain the recovery of gross sensory and motor functions in a transplanted hand. However, recovery of more refined sensory and motor functions requires experience-dependent, cortical-level adaptations to compensate for the inevitable peripheral nerve reinnervation errors, as well as reorganization at the spinal and brain stem levels. Recovery of intrinsic S1 hand territory (critical for sensory localization), and the development of representations within the M1 (necessary for nonsynergistic control of intrinsic hand musculature), depend on experience-driven changes, i.e., learning. This is a more long-term process that accounts for the fact that these functions appear to continue to improve many years after hand transplantation [67]. It is in this latter stage of recovery that rehabilitation guided by advances in modern neuroscience has the greatest potential for impact.
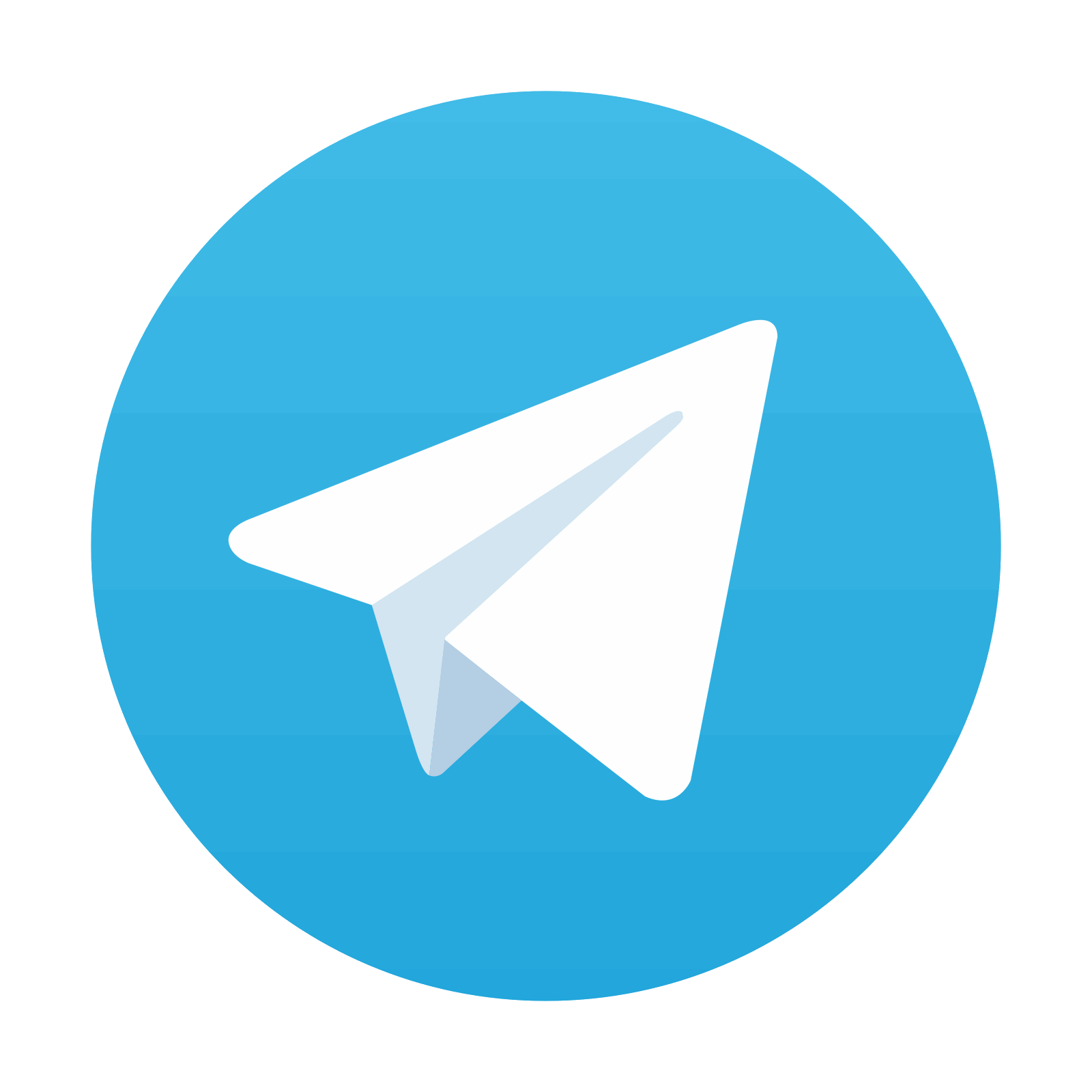
Stay updated, free articles. Join our Telegram channel

Full access? Get Clinical Tree
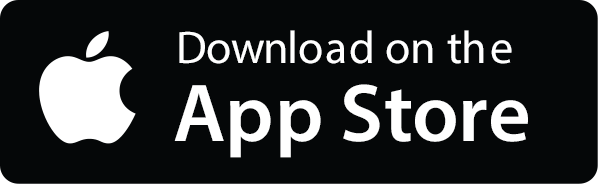
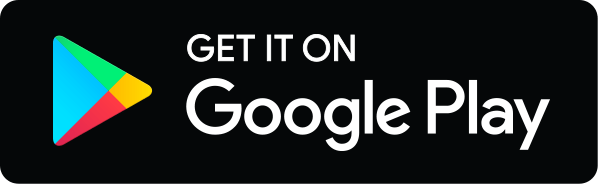