Microvascular free tissue transfer in head and neck surgery has evolved through technological advancements, focusing on enhancing surgical outcomes and reducing complications. The strategic process involves preoperative planning for perforator mapping, intraoperative devices for microvascular anastomoses and patient positioning, and innovative postoperative monitoring techniques ensuring flap viability. This workflow has led to a high flap success rate, although revision surgery can still be necessary. This article aims to explore recent advances in microvascular free tissue transfer technology utilized across the preoperative, intraoperative, and postoperative domains for enhanced surgical efficacy.
Key points
- •
New developments in photoacoustic tomography, including the “PreFlap” algorithm, demonstrate innovative ways to translate 3 dimensional imaging of perforator vessels to 2 dimensional mapping during an operation.
- •
Exoscope is a new technology that has several potential benefits compared to traditional operative microscopy; however, associated costs and provider experience with microscopy may limit adoption in head and neck surgery departments.
- •
Patient limb positioners can decrease the need for additional operative members and improve the exposure of flap sites compared to manual arm support.
- •
While there are a variety of adjunct options available for monitoring microvascular free tissue transfers postoperatively, they should continue to be used in addition to the gold standard of clinical monitoring.
Introduction
Microvascular free tissue transfer (MFTT) has become an essential surgical reconstructive procedure that has special utility in head and neck surgery given the myriad of functional, esthetic, and 3 dimensional (3D) anatomic considerations of the craniofacial region. As MFTT reconstruction has become more commonplace, research has been focused on improving surgical outcomes such as surgical time, patient length of stay, and flap success rate. Preoperative planning has been an area of interest to assist in reducing operative time and improving patient safety for the preparation of MFTTs with more variable anatomy, namely perforator-based flaps. Technology utilized intraoperatively in microvascular surgery has also been advanced overtime to improve surgeon efficiency, operative visualization, and assessment of microvascular anastomoses. Lastly, there have been several innovations in the monitoring of the viability of MFTTs postoperatively to ensure high flap success rates. With a strategic process for preparation and surgical implementation of MFTT with these recent advances, the success rate of MFTT exceeds 95%. However, more than 10% of flaps require revision indicating the need for further technological advancement. The aim of this article is to discuss recent innovations in head and neck MFTT surgery within the preoperative, intraoperative, and postoperative settings.
Preoperative
Computer Tomography Angiography
Head and neck surgeons may utilize computer tomography angiography (CTA) for surgical planning to optimize flap design and donor site. One common situation is in patients requiring a fibula-free flap (FFF) for reconstructive mandibular or maxillary surgery. The lower limb relies on vascular supply from the peroneal artery, posterior tibial artery, and anterior tibial artery. Peripheral arterial occlusive disease, anatomic variants, or stenosis of any of these 3 arteries may lead to critical limb ischemia from the harvesting of an FFF. Furthermore, CTA for FFF planning has been shown to identify nearly 95% of cutaneous perforators found intraoperatively on average within 1 cm from the predicted location.
Beyond FFF, previous research has also shown the benefit of CTA for other perforator-based flaps such as anterolateral (ALT) and thoracodorsal artery perforator (TDAP) flaps. Chen and colleagues demonstrated that the use of CTA significantly reduced the time to harvest ALT flaps; however, there was no difference in the number of perforators identified. Furthermore, a study by Kim and colleagues reported the detection rate of perforators by CTA for an ALT flap was 92%. When considering TDAP flaps, Mun and colleagues found that preoperative localization of TDAPs by CTA minimized incision length, reduced harvest time, and allowed for the planned use of multiple perforators for better tissue perfusion.
As with many imaging modalities, there are also downsides to utilizing CTA for preoperative planning, including radiation exposure, overall cost burden, and the contrast load necessary.
Infrared Thermography
Infrared thermography (IT) is another tool that can be utilized for guidance in finding perforators for MFTT. IT is a noninvasive tool that uses infrared technology to assess tissue perfusion by measuring skin temperature. In clinical practice, skin is cooled, and then thermal images can be generated by the recovery rate and thermal pattern. Hotspots on the thermogram are due to areas of rapid rewarming and correlate with perforating arteries. A study by Chava and colleagues demonstrated that the hotspot on IT correlated with the intraoperative location of perforators in 93% of cases. Two prior studies have shown that IT with arterial Doppler sounds is as accurate as CTA in identifying the dominant perforator. , A study by Xiao and colleagues reported that IT had a sensitivity of 94% and specificity of 86% compared to color Doppler ultrasound. Additionally, Pereira and colleagues found a sensitivity and specificity of 100% and 98%, respectively, when detecting perforators in the ALT thigh flap territory. The primary limitation of this mapping method is the inability to provide information on the anatomic path of the perforator or its caliber.
Photoacoustic Tomography
Photoacoustic tomography (PAT) is a noninvasive imaging method that leverages near-infrared light to induce thermoelastic expansion, thereby generating ultrasound waves. The generated ultrasound waves provide good 3D spatial resolution of deep tissue. This technique is particularly promising for the planning of thin ALT flaps with a branching pattern of perforating subcutaneous vessels. It is a newer technology that has had limited use in the operating room to date. Tsuge and colleagues demonstrated PAT’s capability in identifying branching patterns of vessels within subcutaneous ALT flaps, with superior visualization of subcutaneous microvessels compared to ultrasound alone. However, implementation challenges included limited visualization of subfascial vessels and difficulty in distinguishing between arteries and veins. Tsuge and colleagues attempted to address the lack of intraoperative usability of PAT by creating 2 dimensional (2D) body-attachable sheets from a 3D image for surgeon use. They demonstrated that PAT imaging matched the intraoperative findings within 10 mm. Yet, the absence of a direct navigation method for applying vascular maps to patients and time-intensive sheet preparation remained as obstacles. In a recent development by Munisso and colleagues, an initial “PreFlap” algorithm was introduced to semiautomatically convert 3D PAT images into 2D vascular mapping images. Despite this advancement, challenges persist, including skin shrinkage after flap elevation, which underscores the ongoing need for refinement before the widespread adoption of PAT in preoperative planning becomes feasible.
Color Duplex Ultrasonography
Color Doppler ultrasound (CDU) builds upon traditional Doppler ultrasound by overlaying color onto the grayscale image. CDU provides valuable data regarding direction, internal vessel diameter, and velocity of blood flow rendering it a promising preoperative planning tool in MFTTs, particularly in ALT flaps. , In a systematic review conducted by Cheng and colleagues, CDU exhibited a pooled sensitivity of 95.7% and a pooled positive predictive value of 94.3% when compared to intraoperative findings. Additionally, a prospective cohort study by Debelmas and colleagues, involving 22 thin ALT perforator flaps, illustrated only a 5 mm disparity between preoperative CDU-planned perforator locations and their actual intraoperative positions. Beyond merely pinpointing perforator locations, CDU offers insight into perforator hemodynamics, including blood flow velocity and pulsatility index, thereby contributing to a more comprehensive understanding of optimal flap design. A review by Khoong and colleagues highlighted the integration of CDU with contrast-enhanced ultrasound (CEUS). This CEUS technology utilized sulfur hexafluoride microbubbles for reducing noise and enhancing echogenicity of blood, revealing perforators as small as 0.5 mm. Limitations of CDU include cost, time, and the need for a well-developed knowledge base of ultrasound technology. However, by utilizing the step-by-step guide published by Kehrer and colleagues, microsurgeons can overcome the learning curve to enhance perforator mapping.
Intraoperative
Microvascular Couplers
The microvascular anastomosis is one of the most critical portions of MFTT, and problems that arise can lead to poor perfusion and flap loss. Microvascular coupler devices (MCDs) were introduced to reduce ischemia time and increase patency rates compared to the more traditional hand-suturing method. The MCDs are typically utilized for end-to-end (ETE) anastomosis of arteries and veins. In contrast to hand-sewn (HS) anastomoses, utilizing the coupler enables the eversion of vessel walls by 90°, which allows for both vessel lumens to be visualized. This technique reduces the likelihood of suboptimal suture placement, uneven positioning of vessel lumens, and accidental back-walling of stitches. Although MCDs can be utilized for both arteries and veins, venous couplers have been more widely accepted for microvascular anastomoses.
A large systematic review and meta-analysis of all free flap sites were completed by Maruccia and colleagues comparing MCDs and the HS technique for venous anastomoses. The study consisted of over 13,000 free flaps with an overall thrombosis rate for the MCD group of 1.47%. Their results indicated that the use of venous couplers significantly decreased anastomotic time and postoperative flap failure risk without a significant change in the risk of postoperative venous thrombosis compared to the HS group. There have also been several studies performed within head and neck surgery that have shown venous couplers to be beneficial in decreasing operative time and improving vessel patency.
As previously stated, ETE is the most common technique used for venous anastomosis in head and neck surgery, but recent research has aimed to provide more insight into end-to-side (ETS) venous anastomoses. A retrospective study by Stewart and colleagues found ETS cases to have a significantly higher flap failure rate compared to ETE cases at 11.2% and 3.8%, respectively. Furthermore, their study showed that ETS was more likely to be needed in patients with a history of prior neck dissection. Flap survival for the ETS cohort was improved with a second anastomosis as well as the utilization of an MCD. These results indicate that ETS using an MCD is a feasible option when ETE is not possible.
The use of MCDs for arterial anastomosis is less commonly utilized compared to veins. Arterial vessels have thicker walls compared to veins and may be affected by atherosclerosis or radiation-induced thrombosis or scarring from previous radiation for head and neck cancer, limiting widespread acceptance of MCD. Initial literature on this topic reported concerns about the potential increase in arterial thrombosis rates when using MCDs. However, more recent studies have not supported these findings. A large meta-analysis of 622 arterial anastomoses performed by MCDs reported a 2.1% arterial thrombosis rate. The overall flap fail rate with the use of an arterial MCD was also low (0.96%). These results are aligned with general MFTT success rates and demonstrate that arterial MCD is a safe alternative to HS anastomoses; however, further research and information are needed to confirm the findings within this systematic review and identify patients for whom arterial MCD would not be recommended. The primary criticism of the use of microvascular couplers is the associated costs of the devices. Yet, this needs to be considered in the context of potential reduction in operative time and comparable or improved patency rates (thereby additional potential reduction in operative costs with revision procedures and resultant increased length of stay).
Patient Limb Positioners
The utilization of the patient limb positioners has greatly aided intraoperative surgical techniques for subscapular flaps. The historic conventional approach for a subscapular system flap harvest calls for moving patients into a prone or lateral decubitus position, with the need for an additional team member for arm retraction to ensure adequate exposure during flap elevation. This system allows for minimal patient repositioning, potential simultaneous harvest during the ablative portion of the procedure, optimal exposure to the head and neck region, and eliminates the need for manual assistance. They are already widely used in orthopedic surgery and can be easily transferable to the head and neck setting.
Bender-Heine and colleagues studied 105 patients undergoing free flap procedures with 53 using the Spider Limb Positioner. Compared to manual arm support, the Spider Limb group had significantly shorter surgical times (486 vs 568 minutes).
Stevens and colleagues also found faster surgeries (466 minutes) with no nerve compression or positioning issues in 73 patients. This same research team, led by Smith and colleagues, expanded the application of the limb positioner to fibular flaps. Traditionally, FFF harvest via a lateral approach requires hip flexion and internal rotation, knee flexion, and an additional team member to stabilize the ankle and foot. The use of the Spider Limb allowed the elevation of the foot above the table without manual assistance, improving operator ergonomics and simplifying the dissection and closure process.
While the Spider Limb Positioner presents certain limitations, such as a finite range of articulation, length, and loading capacity (50 lbs), its cost-effectiveness merits consideration. Set-up of the positioner requires an extra 15 minutes although this is marginal when looking at the reduction in total surgical time. Limb positioners additionally cut down on surgical time as the reconstructive and ablative team can work together.
Three Dimensional Exoscope
Magnification is needed for MFTT anastomoses and has traditionally been achieved using surgical loupes/operating microscopes. However, emerging technology in the form of 3D stereoscopic camera-based viewing systems offers a novel approach to magnification while also addressing surgeon ergonomics and educational benefits for the operating room (OR) team. This technology known as the exoscope can be utilized for dissection and microvascular anastomosis procedures, presenting a promising advancement in surgical visualization techniques.
An observational study of 10 consecutive patients undergoing free flap reconstruction by Molteni and colleagues utilized the VITOM 3D system for microsurgical anastomoses, flap harvesting, and inset. Their study showed by using the exoscope that the average time for microvascular anastomoses including 1 artery and 1 vein was 34 minutes with a range of 32 to 38 minutes. There were no intraoperative complications or postoperative flap failures. Another study by McMaine and colleagues had a flap success rate of 93.4% (43 out of 46), which is similar to the expected success rate of 95%. An additional study comparing operative microscopy and the exoscope showed no difference in operative time, ischemia time, or microvascular complications.
Additionally, Molteni and colleagues also performed a subjective assessment of the surgeon’s experience that showed that surgeons experienced no neck or back pain throughout 10 surgeries. Additional benefits of the exoscope were reported by a study assessing surgeons’ and nurses’ feedback for microvascular anastomoses for free flap reconstructions. In their assessment, the highest rated items were ergonomics, ease of use, and the possibility of sharing the view among all members of the surgical team. Conversely, the main criticisms of the exoscope were the luminance of the surgical field and the eye strain from the 3D glasses. This study also noted a 100% flap success rate after approximately 5 month follow-up.
Furthermore, an observational study comparing the exoscope with traditional operating microscopy after 46 cases of head and neck free flap reconstructions using the exoscope noted that the benefits of the exoscope were ergonomic position and improved visualization and orientation by the assisting surgeon. When utilizing the operative microscope, the primary surgeon places the machine head and eyepieces in an area that provides the best surgical view. This can result in the assistant surgeon adjusting their body to allow their eyes to utilize the secondary eyepieces, which may lead to straining, awkward posture/balance, and increase difficulty assisting in the microvascular surgery. This is in comparison to the exoscope, where the same 3D high-definition image that the primary surgeon visualizes can be viewed by the entire surgery team in an optimal location. Finally, the exoscope provides better angulation and is easier to drape than the operative microscope.
A major limitation of adopting the exoscope is the starting cost, which is approximately $250,000 to over 500,000 with additional add-ons such as tracking instruments or monitors. The costs of adopting the exoscope must be considered with the associated benefits of the novel instrument. This cost may be viewed as a benefit, however, when taken in context of the cost of a traditional operating microscope that is significantly more expensive. Additionally, the exoscope may be a lower cost alternative for institutions where operating microscopes may be in limited supply when used by multiple service lines.
Fluorescent Angiography
Fluorescent angiography, also known as indocyanine green (ICG) angiography, involves the intravenous injection of a fluorescent water-soluble dye, which binds to plasma proteins and fluoresces under near-infrared light while traveling through the microcirculation. In MFTT surgery, fluorescent angiography aids surgeons in assessing flap perfusion and identifying vascular compromise or tissue hypoperfusion in real time. By visualizing the fluorescence of the dye within the blood vessels, surgeons can evaluate the patency and adequacy of arterial inflow and venous outflow of the flap. This information helps in ensuring proper microvascular anastomosis and flap viability during surgery. A recent retrospective study looked at the total flap failure rate, reexploration rate, and flap salvage rate between surgeries utilizing fluorescent angiography and those without. Researchers concluded that utilization of ICG angiography significantly decreased total flap failure rate, significantly increased salvage rates, but did not affect the reexploration rate.
Fluorescent angiography offers several advantages in MFTT surgery. It provides immediate feedback on flap perfusion, allowing surgeons to make intraoperative decisions regarding flap design, repositioning, or revision to optimize blood flow and prevent flap failure. Additionally, it helps identify any areas of inadequate perfusion or vascular compromise early on, enabling prompt intervention to salvage the flap or modify the design or shape of the cutaneous component of the flap. Furthermore, fluorescent angiography is noninvasive and does not require radiation exposure. The dye has been demonstrated to have a high level of safety. Hope-Ross and colleagues’ sizable study looked at the use of fluorescent angiography in 1226 patients. There were a total of 3 mild, 4 moderate, and 1 severe adverse reactions. The severe reaction involved the cardiac, respiratory, and neurologic systems, but there were no patient deaths. However, providers should be wary of the use of ICG in patients with a history of iodine allergy, uremia, pregnancy, and liver disease.
Postoperative
Implantable Dopplers
Postoperative flap viability monitoring remains crucial for early intervention in cases of compromised blood supply. Traditional methods, such as clinical staff monitoring, are still the gold standard; however, they may lack real-time vascular flow data. Implantable Dopplers offer direct and immediate measurement of flow in pedicle vessels. The Cook-Swartz Doppler system introduced by Swartz and colleagues has become one of the most popular methods for free flap monitoring in head and neck surgery. It employs a 20 MHz pulsed ultrasonic Doppler probe fixed via silicone to a cuff of expanded polytetrafluoroethylene, directly secured to the pedicle vessels for continuous blood flow monitoring. The probe wire exits the wound and is connected to a monitor. These probes can facilitate both arterial and venous monitoring, depending on the vessel they are secured to. They are also particularly useful with buried flaps without a cutaneous paddle. A systematic review revealed that the use of implantable Doppler probes significantly reduced flap failure rates (2.11% vs 4.21%) and increased successful salvage rates (83% vs 59%) compared to clinical assessment alone. However, concerns have been raised regarding the high false-positive rate with venous monitoring due to probe dislodgement and potential pedicle disruption upon wire removal.
Recently, wireless implantable Dopplers have garnered attention. However, these systems are limited by bulk and battery life. Rothfuss and colleagues developed a 1.70 cm 3 (without battery) implant, demonstrating a relative error rate of less than 5% above a flow rate of 8 mL/min, calibrated with in vitro models. Yet, implementation in porcine in vivo models proved challenging due to noise obscuring the flow signal. While wireless Dopplers may mitigate dislodgement and pedicle disruption risks, further development is required for accurate blood flow assessment.
Color Duplex Doppler Ultrasonography
Like its use in preoperative planning, color Doppler ultrasound (CDU) is a beneficial tool for separately monitoring arterial and venous flow of a pedicle in the postoperative period. It serves as a noninvasive, repeatable, and objective diagnostic tool, utilizing a compact linear probe. Seres and colleagues published a case series demonstrating the use of CDU in aiding decisions for reexploring flaps, highlighting 3 cases in which surgical reexploration was avoided with detection of intact arterial and venous circulation. Vakharia and colleagues investigated the utilization of CDU in buried flaps with gracilis muscle transfer for facial reanimation. Buried flaps present monitoring challenges posed by the absence of a cutaneous paddle and the potential for muscle edema obscuring signs of vascular insufficiency. CDU can not only evaluate flap perfusion but can also assess muscle quality and help detect seroma or hematoma that may necessitate intervention. CDU can also be used as an adjunct in scenarios where implantable Doppler probes demonstrate a loss of signal, distinguishing false-positive results from true vascular compromise.
Despite its clinical benefits, CDU is not without limitations. The cost of the device, ranging from $30,000 to $225,000, poses a financial barrier, and comprehensive education of the surgical team is essential for proficient utilization.
Near-Infrared Spectrophotoscopy
Near-infrared spectrophotoscopy (NIRS) is a tool that can be used to monitor MFTTs postoperatively. NIRS employs near-infrared light to assess tissue characteristics such as oxygenated and deoxygenated hemoglobin concentrations. By measuring the absorption and reflection of near-infrared light, NIRS provides insights into tissue oxygenation levels and perfusion dynamics by presenting findings as an alteration in the percentage of saturated hemoglobin (StO 2 ). Surgical teams can monitor this number to assess transplanted tissue viability. Unfortunately, there is no established cutoff to alert the team to assess the free flap. A study by Repez and colleagues used a StO 2 level of 50% below the initial postoperative measurement. In this study, NIRS monitoring successfully identified all cases of vascular compromise of the flap before manifesting observable clinical signs recognizable by skilled personnel. A study by Starr and colleagues defined an optimal cutoff of postoperative StO 2 as 68% by a recurrent operating character curve for classifying flap success at discharge. However, their analysis showed that there was no difference in flap success rate when monitored postoperatively by Doppler or NIRS. Conversely, a systematic review by Chen and colleagues reported significantly higher salvage rates and lower flap loss rates in the NIRS cohort compared to the non-NIRS cohort. However, there was no difference in free flap reexploration between groups.
The primary advantages of NIRS include the accuracy, the noninvasive aspects of the tool, and the ability of the method to differentiate between venous versus arterial vascular crises. However, the disadvantages include a lack of established StO 2 cutoff, the price of the device, the potential need for re-arrangement of the sensors, and the inability of the application to be utilized for buried flaps.
Laser Doppler Flowmetry
Laser Doppler flowmetry (LDF) is another tool that may be used to monitor MFTTs for possible vascular impairments. The noninvasive device uses a photodetector within the cutaneous surface of a free tissue transfer to assess for changes in microvasculature perfusion. The device can be used for continuous monitoring beginning within the operating room. A previous study began recording values at shorter intervals for the first 2 hours postoperatively and then switched to every hour for 3 to 5 days. In this study, the attending or resident would be notified if the flow decreased by 50% or greater for at least 20 minutes. Research also has demonstrated that the flowmeter can detect alterations in values that signify flap compromise up to 1 to 3 hours before any clinical signs become apparent. Additionally, a study by Salvatori and colleagues identified LDF to have a positive predictive value of 100% with a negative predictive value of 0% for the detection of vascular compromise in free flaps. There are several advantages of LDF in the postoperative monitoring of MFTTs including real-time assessment, noninvasiveness, high sensitivity, and early detection of complications. Conversely, the disadvantages of LDF are the inability to differentiate between arterial and venous thromboses, the lack of utility in buried flaps, complexities in data interpretation, and associated costs.
Digital Infrared Surface Thermometer Monitoring
Surface thermometer monitoring is one of the oldest and simplest methods for postoperative monitoring of free flaps. This approach provides a cost-effective means of assessing flap viability by measuring skin surface temperature using no-contact infrared thermometers. Previous studies have shown a high sensitivity of 98% and a positive predictive value of 75% for surface temperature recordings in detecting flap compromise.
Kraemer and colleagues demonstrated the close relationship between free flap skin temperature and microcirculatory capillary blood flow. An acute temperature drop of 3°C at the center of the skin flap may indicate arterial thrombosis, while a uniform temperature drop of 1°C to 2°C across the flap may suggest venous compromise.
Advancements in technology have enabled continuous monitoring using digital infrared thermal imaging. Perng and colleagues demonstrated the use of infrared cameras on swine pedicles to detect the entire flap surface temperature rather than a singular point. A temperature difference of less than 0.86°C was identified as a criterion for vascular occlusion, with a sensitivity of 90% and specificity of 81%. However, there were minimal differences in temperature between arterial and venous occlusion with considerable variability.
Despite its advantages, digital infrared surface thermometer monitoring has limitations. Its limited spatial resolution and dependence on factors such as room temperature, airflow, and flap location or coverage with dressings or blankets can impact accuracy. Nonetheless, the development of thermal models such as that created by Perng could account for room and body temperature in calculating flap surface temperature. Furthermore, while effective in detecting flap failure, this monitoring technique may not always detect impending compromise before irreversible damage occurs.
Summary
There have been significant advancements within head and neck surgery for preoperative perforator mapping, intraoperative-assisted devices, and postoperative monitors of MFTTs allowing for potential improved flap success rates. Surgeons should assess both the benefits and the limitations of the technology available and adapt practices that complement their current clinical practice. These technological advances have been shown to improve preoperative planning, decrease surgery length, and improve flap success that may outweigh the upfront cost/learning curve of adopting these devices. The field remains open for continued innovation with the goal of improving patient outcomes.
Clinics care points
- •
The application of various technological advances in the preoperative, inraoperative, and postoperative setting have improved efficiency and sucess in microvascular free tissue transfer of the head and neck.
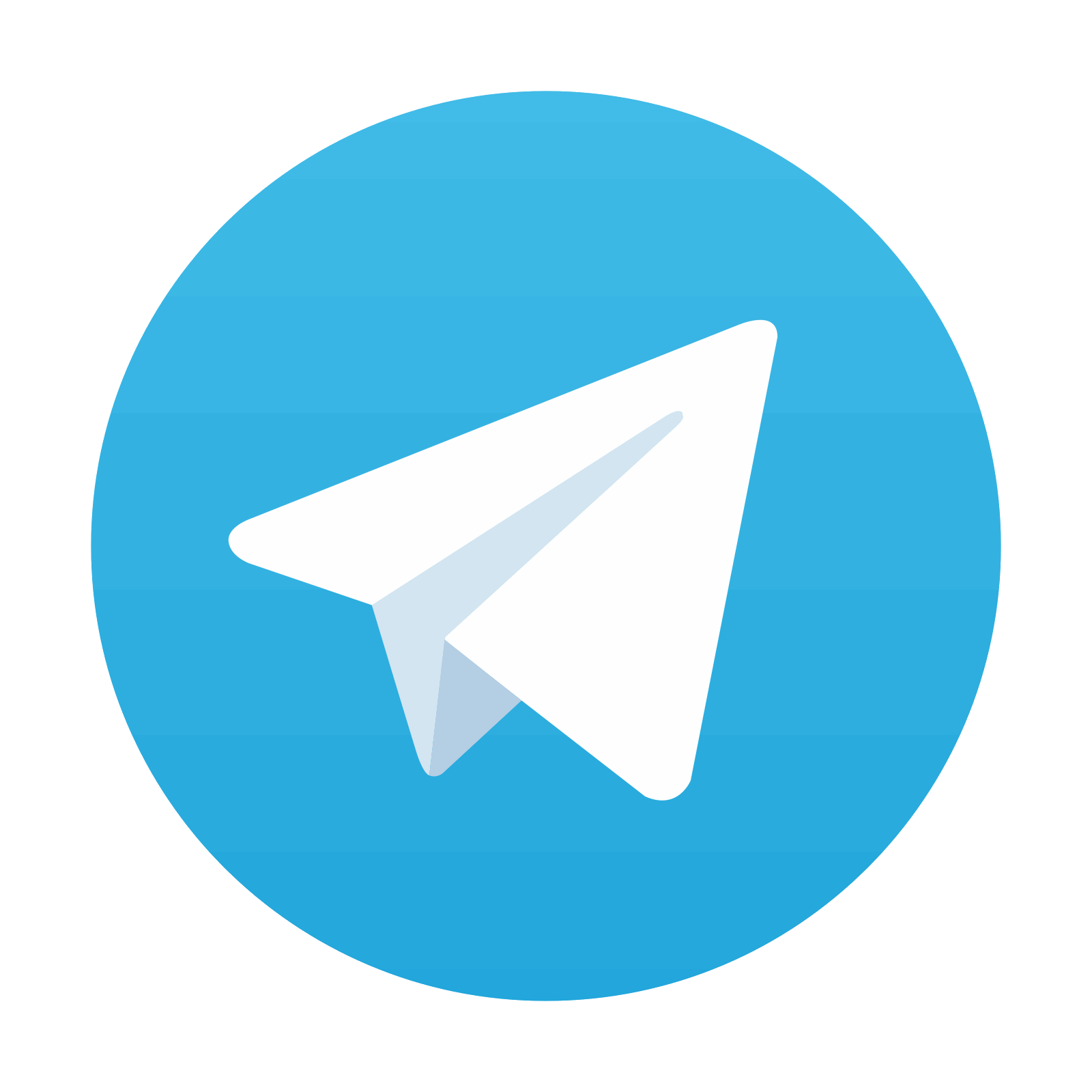
Stay updated, free articles. Join our Telegram channel

Full access? Get Clinical Tree
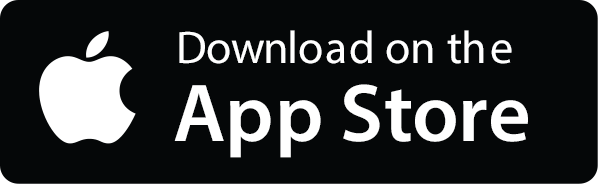
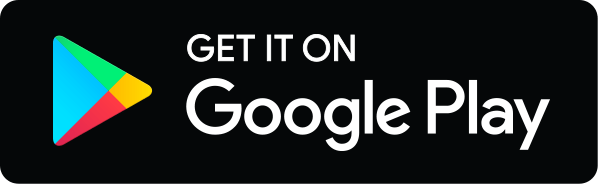
