34 Transplantation in plastic surgery
Synopsis
The most important antigens contributing to allograft rejection are the major histocompatibility complex (MHC) antigens.
The immune system has two main arms that mediate both rejection and tolerance to foreign antigens: the humoral response (B cells and antibodies) and the cell-mediated response (T cells).
T lymphocytes have a central role in coordinating the immune response, forming the cell-mediated arm of the immune response.
Acute rejection takes place days to weeks after transplantation and occurs with rapid onset. This T-cell mediated response is characterized by fever, graft tenderness, and edema, and loss of function. Interstitial lymphocytic infiltration is seen on microscopic examination.
Chronic rejection is characterized by fibrosis and severe organ dysfunction. This process usually occurs over years.
Immunosuppressive medications must inhibit the body’s ability to reject a transplanted organ, but not at the expense of the defense network against pathogens.
Despite the use of powerful immunosuppressive medications most transplant patients experience episodes of acute rejection.
Two major issues in hand transplantation are the need for maintenance immunosuppression and the evaluation of the functional outcomes of the transplants.
Currently, clinical hand transplantation remains an experimental procedure, and the long-term outcomes of this innovative procedure are still being determined.
The fields of transplantation and plastic surgery have always been closely linked. In fact, the age of organ allotransplantation in the US began when Joseph E Murray, a plastic surgeon, transplanted a kidney between identical twin brothers in 1955.1 Such “reconstructive surgery” using organ allografts was one of the great achievements in 20th-century medicine. Plastic surgeons often reconstruct tissue defects via transplantation of autologous tissue from other regions of the body. Nonvascularized skin, bone, and cartilage grafts alone or in combination with axial and random flaps are common everyday procedures used to reconstruct tissue defects. However, these surgical techniques all have significant limitations, often require revisions, and leave the patient with a donor site. Advances in the development of skin substitutes (prepared from allogeneic or xenogeneic sources) and the application of frozen bone allografts have shown the possibility of reconstruction without a donor site but their current application is limited. Despite advances in plastic and reconstructive surgery, including the refinement of microvascular techniques and delineation of flap vascular anatomy, many complex wounds, especially those on the central face, still remain outside the realm of possibility for restoring both form and function.
The inability to reconstruct missing tissues accurately occurs when the surgeon must deviate from the principle of replacing “like with like” due to a lack of appropriate autologous donor sources. Thus, the function of a severely injured extremity cannot be adequately restored, the appearance of a severely disfigured face cannot be satisfactorily improved, and both the function and appearance of an amputated extremity cannot be reconstructed. Understandably, the inclination toward tissue transplantation has led plastic surgery researchers to look to nonautogenous sources for reconstructive material.2–4 However, the ability to engineer tissue from single cell sources has yet to yield a technique that can provide the complex tissue constructs needed. One technique with the potential for providing access to complex vascularized tissue constructs without the need for a donor site is through the process of allotransplantation.
The clinical feasibility of composite tissue allotransplantation (CTA) has been demonstrated by the successful transplantation of over 50 hands and nine faces.5–9 This emerging field of reconstructive transplantation could represent a paradigm shift in the arena of reconstructive surgery. The application of these transplants to patients with complex wounds would provide the reconstructive surgeon with the opportunity to reconstruct with the exact tissues lost. However, unlike traditional solid-organ transplants (kidney, liver, and heart) that consist of relatively homogeneous parenchymal tissue, the composite tissue allograft is comprised of multiple heterogeneous tissue types (skin, bone, and muscle). Each of these distinct tissue types has been shown to exhibit varying degrees of antigenicity, with skin and mucosa the most antigenic.10 While solid-organ transplantation is the gold standard for the treatment for end-stage organ failure, there has yet to be true consensus on the use and application of composite tissue allografts.11,12 The survival of the hand and partial face transplants is dependent on the use of chronic immunosuppression and their application is currently limited to experimental institutionally approved protocols. In order for the field of reconstructive transplantation to expand its indications beyond the experimental arena, techniques need to be designed either to significantly reduce or eliminate the need for chronic immunosuppression. The future direction of this field is heavily dependent on the development of innovative approaches to the use of immunosuppressive agents and tolerance induction protocols.
Transplantation immunology
Major histocompatibility complex
There are two major classes of MHC genes. Class I MHC genes encode a transmembrane glycoprotein complex with a polymorphic 44-kDa heavy chain consisting of three extracellular domains (α1, α2, and α3). The α1 domain is highly variable and contains sites for antigen binding. The heavy chain is stabilized by noncovalent binding to a lighter chain, referred to as β2-microglobulin. There are three distinct genetic loci for the class I antigens in the human: HLA-A, HLA-B, and HLA-C. Class I antigens are expressed on nearly all nucleated cells and serve as the primary target for cytotoxic (CD8+) T lymphocytes. Class II MHC genes encode two noncovalently bound transmembrane proteins, a 34-kDa α chain and a 29-kDa β chain. There are three class II loci in humans: HLA-DR, HLA-DP, and HLA-DQ.13 Class II antigens are expressed primarily on vascular endothelium and cells of hematopoietic stem cell origin such as lymphocytes and macrophages.
Both class I and class II molecules have a specific site at which foreign peptide antigens can be presented after they have been processed by the cell.14–17 Tissue distribution is not the same in all species: humans, dogs, pigs, and monkeys express class II antigens on endothelial cells, whereas rodents and many species do not.18 Matching of HLA-A, HLA-B, and HLA-DR has been found to be an important factor in determining long-term renal allograft survival.19
Other transplant antigens
The blood group antigens are important in clinical transplantation because they are expressed on vascular endothelial cells. Patients with type A or type B blood develop natural antibodies to the other protein, whereas patients with type O blood develop natural antibodies to both type A and type B proteins. Although ABO antigens will not stimulate cell-mediated rejection, a brisk antibody-mediated attack can rapidly lead to graft failure.20
Minor histocompatibility antigens are peptides of self origin that are not presented by the MHC complexes. Siblings (other than identical twins) with a completely matched MHC profile will still differ with respect to minor antigens because of allelic variation of the genes encoding those proteins. Although minor antigens will stimulate a cell-mediated response, they will not do so in a primary in vitro test. Rejection of a graft due to minor antigens alone, therefore, often proceeds at a slower rate.21
Skin-specific antigens (Sk antigen) are tissue-specific proteins that can cause graft rejection by a cell-mediated response. Consequently, skin is one of the most difficult tissues to which transplantation tolerance can be induced.22
Immunologic rejection cascade
Cells of immune response
Macrophages
Beyond simple destruction of cells, the main purpose of the macrophage is to reprocess the breakdown products of ingested cells. These fragments of foreign protein may then be bundled with a new class II antigen molecule, and during the bundling process, fragments of the foreign protein come to reside in the peptide-binding groove of the class II molecule. When the fragment is exteriorized, it faces outward and is easily recognized by the immune system as foreign. This process is known as antigen presentation. Macrophages also secrete important cytokines,23,24 such as interleukin-1 (IL-1). This polypeptide can, in a hormonal fashion, stimulate the immunologic function of responding cells. These cells are critical to the presentation of foreign antigen.
Natural killer cells
Another primitive cell derived from the bone marrow stem cell is the lymphocyte (non-T, non-B) called the natural killer (NK) cell. NK cells are thought to be active in the antitumor response.25 They are able to demonstrate spontaneous tumoricidal properties on exposure to tumor cells. This cell does not require recognition of MHC molecules or antigen processing (as do T cells and B cells).26 However, the exact method this cell uses to recognize foreign cells remains unclear. They are able to kill cells by incorporating a lipophilic protein into the target cell membrane, which leads to increased permeability and cell lysis. NK cells also secrete several cytokines, including interferon-γ, interferon-α, and B-cell growth factor. They may also serve to eliminate cells that fail to express normal self-MHC proteins and thereby be self-reactive. Finally, they appear to serve as a barrier to the engraftment of donor bone marrow after non-MHC-matched bone marrow transplantation.27
T lymphocytes
Three broad classes of T cells are helper T (TH) cells, cytotoxic T cells, and suppressor T cells. All T cells express CD3 on the cell surface. Cytotoxic T cells (cells that effect target cell killing) express CD8. Suppressor T cells that can buffer and down-regulate the immune response also express CD8.28,29 TH cell, however, expresses CD4 and serves to amplify the immune response through its interaction with other cells and secretion of critical cytokines. Each T cell expresses a T-cell receptor (TCR) capable of binding antigen. The TCR, a 90-kDa heterodimer composed of an α chain encoded on chromosome 14 and a β chain encoded on chromosome 7, is located close to the CD3 antigen and the CD28 antigen. The TCR is relatively flat and possesses an outward-facing surface. This “antigen recognition platform” is the critical interface for foreign peptide in the binding groove. As with B-cell development, T cells undergo rearrangement of genes coding for a hypervariable region on the receptor proteins. This allows a body’s population of T cells to respond to a nearly limitless array of foreign antigens, with each individual T cell capable of binding one specific antigen.
T-cell binding and activation
Although the TCR is capable of binding antigen, it will not recognize the target molecule by itself.30 The TCR can bind antigen only if it has been processed and presented by an antigen-presenting cell together with an MHC molecule; thus, it recognizes the MHC together with the target antigen. This limitation of binding is referred to as MHC restriction. CD4 (helper) T cells can only bind antigen presented with MHC class II molecules; CD8 (cytotoxic) T cells recognize antigen along with MHC class I proteins. The TH cell is most critical to the immune response because its activation results in the production of cytokines that are necessary for the function of many other immune cells. Binding of a CD4 cell to an antigen-presenting cell that expresses target antigen together with MHC class II molecules initiates a predictable cycle of intercellular communication. The antigen-presenting cell is stimulated to produce the cytokine IL-1, a powerful chemoattractant, a primary mediator in the acute-phase reaction, and a potent activator of lymphoid cells. The T cell, in turn, secretes IL-2, which is a required stimulant for differentiation and proliferation of T cells. The IL-2 produced by the bound T cell has autocrine function by binding to newly expressed self IL-2 receptors. Secreted IL-2 also has paracrine function that affects other T cells in the region, such as CD8 cells, which require IL-2 for activation but do not produce it themselves. As CD4 cells become further activated, they secrete IL-4 and IL-5, which stimulate the maturation and proliferation of B lymphocytes. Furthermore, there is evidence that two subsets of CD4 cells, TH1 and TH2, function to enhance alloreactivity and stimulate antibody production by B cells, respectively. With such a central role by CD4 cells in cell signaling, it is easy to understand the severely compromised immune response from the loss of host CD4 cell function secondary to human immunodeficiency virus infection.31–34
Antigen recognition and graft rejection
In the case of allograft tissue, foreign antigens would be recognized by host T cells after being processed by host antigen-presenting cells and presented in the context of self MHC. This is termed indirect presentation. In addition, host T cells can directly recognize donor MHC on donor antigen-presenting cells, termed direct presentation. This mechanism helps explain the more vigorous response to allograft tissue than to foreign peptides alone.35
Hyperacute rejection occurs almost immediately after perfusion of the allograft with host blood. It is the result of preformed antibodies to either ABO blood group proteins or donor MHC molecules enacting a rapid attack on the donor tissue. Complement activation results in destruction of vascular endothelial cells and induces rapid thrombosis of vessels as well as an amplification of the inflammatory signal. Standard screening before transplantation should detect preformed antibodies, making hyperacute rejection a rare clinical entity. Many mammals possess preformed antibodies to other species that stand as a major obstacle to xenotransplantation.36
Acute rejection takes place days to weeks after transplantation and occurs with rapid onset. This T-cell-mediated response is characterized by fever, graft tenderness, and edema, and loss of function. Interstitial lymphocytic infiltration is seen on microscopic examination. In addition, severe forms of acute rejection may include a humoral attack on the graft, resulting in a vasculitis.37
Inflammatory mediators in transplantation
In addition to the direct cellular interaction observed during an immune response there are also multiple inflammatory mediators involved in immune activation and regulation. These include cell adhesion molecules (CAM), cytokines, and chemokines that are all critical to a functioning immune system. Cytokines are transient regulatory proteins that a wide variety of cells can produce in response to stimulation. These proteins can act locally by binding to a cell from the same cell line (autocrine) or to the target cell in the vicinity (paracrine).38 The cytokines are divided into proinflammatory cytokines (IL-1α, IL-1β, IL-6; tumor necrosis factors: TNF-α, TNF-β), cytokines involved in T-cell differentiation (IL-2, IL-4, IL-5, IL-10, IL-12, IL-13, and interferon-γ), and cytokines of immunoregulatory function belonging to the transforming growth factor-β family, which primarily promotes wound healing and fibrosis.38
Chemokines are a subset of cytokines that are currently defined as small chemotactic cytokines. Chemokines, which are constitutively expressed, are involved in homeostatic lymphocyte trafficking to the lymphoid organs and bind to the cellular component by a specific chemokine receptor. The main role of proinflammatory chemokines (macrophage inflammatory protein-1α (MIP-1α), MIP-1β, monocyte chemoattractant protein-1 (MCP-1), and RANTES) is to attract neutrophils to an inflammatory site and trigger T lymphocytes to elicit an additional inflammatory response.39 In humans, CCR1-positive cells increase in the peripheral blood of renal allograft recipients before acute rejection.40 In addition, CCR1 mRNA was found to be expressed in cells derived from biopsies from renal allografts.41 Ruster et al. described an increased number of CCR1-positive cells in glomeruli during rejection.42 Mayer et al. reported that the mRNA expression of CCR1 was associated with the decrease of renal function in allografts with an acute rejection episode.43 Thus, it is clear that chemokines play an important role in allograft rejection and interruption of these interactions could have an impact on allograft survival.
CAMs play a role in leukocyte migration from the circulation to tissues. Three types of CAM are involved in the transmigration process: selectins (L-, E-, and P-selectins) mediating the rolling of leukocytes along the vascular endothelium, integrins (intercellular adhesion molecule-1 (ICAM-1), vascular CAM-1 (VCAM-1), mucosal addressin cellular adhesion molecule-1 (MAd CAM-1)) leading to leukocyte adhesion to the endothelium, and finally immunoglobulin superfamily platelet endothelial CAM-1 (PECAM-1), responsible for transmigration of leukocytes.44 The complex specific migration of leukocytes to the target tissue requires a coordinated process of proinflammatory mediators. Proinflammatory cytokines, IL-1α and TNF-α, may induce expression of proinflammatory chemokines. Chemokines play a major role in the activation of integrins needed for adhesion of rolling leukocytes to the vessel endothelium, and this process leads to leukocyte transmigration to the surrounding tissue, initiating the inflammatory process.
Immunologic screening
Blood group typing is an important first step in determining transplant compatibility. An ABO mismatch will result in certain failure because of preformed antibodies.45 Although it is possible to transplant type O donor tissue into type A or type B recipients, the limited supply of donor organs in the US makes this practice uncommon.
HLA typing is used to match organs with potential recipients. Serologic methods are employed to type HLA-A, HLA-B, and HLA-DR. A heterozygous individual with a complete match at all these loci is referred to as a six-antigen match. For renal allografts, HLA matching has been shown to affect graft survival. Kidneys transplanted from HLA-identical siblings have a 3-year success rate that well exceeds 90%, whereas parent-to-child grafts have an 82% survival. Cadaveric kidney grafts have a 70% 3-year survival rate.46 MHC class II matching has been found to be more important than class I matching for renal transplantation; the reverse appears to be true for liver transplantation. This would suggest differences in mechanisms of graft rejection for different tissues.47 Serologic tissue typing has certain limitations. An HLA antigen can be identified only if it is being searched for with a specific antibody. For example, if only a single HLA-DR phenotype is identified in donor tissue, the individual could be either homozygous for that allele or heterozygous with an unrecognized HLA-DR antigen. This method of testing fails to type the other class II antigens, HLA-DP and HLA-DQ.
Current immunosuppression
The multiple pathways and mechanisms employed by the immune system to defend the body against both extracellular and intracellular pathogens have presented a significant barrier to the survival of transplanted allografts. Immunosuppressive medications must inhibit the body’s ability to reject a transplanted organ, but not at the expense of the defense network against pathogens. The use of several immunosuppressive agents has allowed the inhibition of the immune system that maximizes the protection of the allograft with the least cost to the body’s overall ability to fight infection and tumors (Table 34.1).
In all of the transplanted organs including the hand and face transplants, it appears that it is central to prevent allograft recognition during the peritransplantation period. This is currently achieved through so-called induction protocols. Several agents are currently used to protect the transplant during that period of cytokine excess observed after surgery (Table 34.2). After the induction agents are used, “maintenance” medications are used to maintain the transplant. Finally, when ongoing rejection occurs, it is often necessary to use “rescue” agents to stop ongoing rejection and salvage a transplant that would otherwise be lost.
Corticosteroids
Glucocorticosteroids bind to an intracellular receptor after nonspecific uptake in the cytoplasm. The receptor–ligand complex then enters the nucleus, where it acts as a DNA-binding protein and increases the transcription of several genes.48 The most important gene is thought to be IκBα, which binds to and prevents the function of NF-κB (a key proinflammatory cytokine that is an important transcription factor for T-cell activation). Steroids block the production of IL-1 and TNF-α by antigen-presenting cells. They also block interferon-γ production by T cells and migration and lysosomal enzyme release by neutrophils. Steroids also mute the up-regulation of the MHC and, through their diminution of the inflammatory responses, decrease the degree of costimulation in the environment. Steroids do not have an impact on the production of antibody.
Antiproliferative agents
Mycophenolate mofetil
Mycophenolate mofetil (MMF), which was approved for use after allograft transplantation in 1995, acts through noncompetitive, reversible inhibition of IMP dehydrogenase.49 This modification improves the bioavailability of mycophenolic acid. Physiologic purine metabolism requires that GMP be synthesized for the subsequent production of guanosine triphosphate (GTP) and deoxyguanosine triphosphate (dGTP). GTP is required for RNA synthesis and dGTP for DNA synthesis. GMP is formed from IMP by IMP dehydrogenase. MMF prevents a critical step in both RNA and DNA synthesis. However, MMF does not affect the “salvage pathway” for GMP production that is present in most cells. This pathway is not present in lymphocytes, and MMF exploits this difference and spares most other cells in the body, including neutrophils. MMF blocks the proliferative response in both T and B cells, inhibits antibody formation, and prevents clonal expansion of cytotoxic T cells.
MMF decreases biopsy-proven rejection and the need for antilymphocyte agents in rescue therapy compared with azathioprine.50–52 MMF has replaced azathioprine in clinical transplantation. However, MMF cannot be used as a sole immunosuppressive agent and must be paired with either steroids or, more commonly, calcineurin inhibitors (tacrolimus and cyclosporine).
Calcineurin inhibitors
Cyclosporine
Cyclosporine is a cyclic endecapeptide that was isolated from the fungus Tolypocladium inflatum gams in 1972.53,54 This drug acts as a T-cell-specific immunosuppressant, and its mechanism of action is primarily through its ability to bind to the cytoplasmic protein cyclophilin.53 The cyclosporine–cyclophilin complex forms a high-affinity bond with calcineurin–almodulin complex and blocks the calcium-dependent phosphorylation and activation of NF-AT. The interference with NF-AT prevents the subsequent transcription of the gene encoding IL-2. This process also interrupts other genes critical for T-cell activation. In addition, cyclosporine increases transforming growth factor-β transcription, which appears to down-regulate T-cell activation further, decrease blood flow to the area, and activate pathways critical to wound healing.54,55
The effect of cyclosporine is reversible because it blocks TCR signal transduction but does not inhibit costimulatory signals.56 If the drug is withdrawn, the T cell is not anergic but is again capable of mounting an attack on its target. The effects of cyclosporine can be overcome with the exogenous administration of IL-2. This may explain why cyclosporine is not effective once rejection is ongoing; it is only useful as a maintenance agent and is ineffective as a rescue agent.
Tacrolimus
Tacrolimus (FK506), a macrolide produced by Streptomyces tsukubaensis, was discovered in 1986. Tacrolimus, like cyclosporine, blocks the effects of NF-AT, prevents cytokine transcription, and arrests T-cell activation. The intracellular target is an immunophilin protein distinct from cyclophilin known as FK-binding protein.56,57 The effect is additive to that of cyclosporine, and these drugs cannot be given together because of the prohibitive toxicity. Tacrolimus also increases the transcription of transforming growth factor-β and thus shares both the beneficial and toxic effects seen in the administration of cyclosporine. It is, however, 100 times more potent in its inhibition of the production of IL-2 and interferon-γ. The renal side-effects are similar to those with cyclosporine. It has more pronounced neurologic side-effects and a diabetogenic effect. The cosmetic side-effects are less than those with cyclosporine. This drug has been proved to be effective as a maintenance drug for both liver and kidney transplantation. It has only minimal use as a rescue agent.58
A topical preparation of tacrolimus has recently been developed and approved for use in atopic dermatitis. Its mechanism of action and local route of administration render it an attractive therapeutic alternative for the treatment of various autoimmune dermatologic conditions and could be of potential use in CTA. It has also been widely used in clinical hand and face transplant immunosuppressive protocols for both maintenance and treatment of rejection.5 There has also been some evidence that it can be used in graft-versus-host disease (GvHD).59 The mechanism by which topical tacrolimus may be effective in GvHD is the suppression of local cytokine secretion such as IL-2, interferon-gamma, and TNF-α in the skin.60 The only adverse events reported in its dermatologic applications have been local irritation, pruritius, erythema, and burning.61,62 The majority of these symptoms are reported to occur when initiating treatment and systemic effects have not been observed.
Rapamycin
Rapamycin is a macrolide antibiotic derived from Streptomyces hygroscopicus and is structurally similar to tacrolimus.63,64 However, they antagonize each other’s biologic activity. Both of the drugs bind to the same FK-binding protein, but rapamycin does not affect the calcineurin activity.65,66 Instead, the interaction of rapamycin and FK-binding protein complex impairs signal transduction by the IL-2 receptor through its interaction with a cytoplasmic protein (RAFT-1). In doing so, the p70 S6 kinase cascade is interrupted and T cells are prevented from entering into the S phase of cell division.67 Thus, rapamycin is able to interrupt T-cell activation and proliferation, even in the presence of IL-2.68 Other receptors that are affected are IL-4, IL-6, and platelet-derived growth factor.
Rapamycin has been shown to prolong allograft survival in multiple animal models and is being used in several drug clinical regimens.69 The drug is most commonly used after the peritransplant period to replace tacrolimus.70 It has also been applied to the experimental human hand transplantation patients who have experienced renal toxicity secondary to tacrolimus.71 This drug has little to no nephrotoxicity. It does, however, demonstrate some bone marrow toxicity and has been observed to cause hypertriglyceridemia. Finally, it appears to interrupt the process of wound healing and caution should be applied before using it immediately after surgery.
Antilymphocyte preparations
Antilymphocyte/antithymocyte globulin
The mechanism behind the effectiveness of these drugs is through the coating of the T cells by the antibodies.72,73 These coated T cells are then eliminated by complement-mediated lysis and opsonin-induced phagocytosis. The mere presence of the antibodies on the surface of the T cell reduces its ability to express an effective TCR signal. The overall impact of the antibodies is functionally to remove the primary effector cells required for acute rejection after transplantation.
The extent of peripheral lymphocyte depletion in the blood appears to be dose-dependent. Although these agents preferentially bind to T cells, they may also bind to B cells, dendritic cells, and other nonlymphoid cell lines, especially at high doses. In fact, two pilot studies have demonstrated that high-dose ATG induction can facilitate monotherapy maintenance immunosuppression in selected patients, with graft and patient survival comparable to the current standard.74,75 Treatment with ATG has been shown to be associated with both short- and long-term changes in T-cell populations, generating altered homeostasis characterized by expansion of specific T-cell subsets that have been shown to exhibit regulatory suppressor functions. The use of ATG induction has been demonstrated to result in a lower incidence of acute rejection and improved graft survival during the first year after transplantation. However, as has been observed with most induction agents, patient and graft survival after 20-year follow-up were not affected.76
OKT3
This is a murine monoclonal antibody that is directed against the signal transduction subunit on human T cells (CD3). OKT3 is thought to bind to the CD3 subunit found on all mature T cells and results in the internalization of the receptor, thus preventing antigen recognition and TCR signal transduction.77,78 In addition, T-cell opsonization and clearance by the reticuloendothelial system occur. After the administration of OKT3, there is a rapid decrease in the circulating CD3+ T cells. There is little or no effect on those cells in the spleen and lymph nodes or thymus. After several days, there is a return to T cells that are CD4+ and CD8+ but that do not express CD3. These “blind” T cells remain incapable of binding to antigen and interfere with the process of antigen recognition and generation of cytotoxic T cells. Finally, OKT3 blocks the cytotoxic activity of already activated T cells by an inappropriate degranulation when the CD3 is bound by OKT3. This mechanism is central to its effectiveness but also to one of its most significant side-effects.
Anti-IL-2
Two monoclonal antibodies have become available for use in renal transplantation and have also been employed in some hand transplantations. Both of these agents (daclizumab and basiliximab) are directed against CD25, the high-affinity chain of IL-2 receptor.79,80 These agents were designed to have the same indications for treatment as ATG and OKT3, without the significant side-effects of those agents.
The high-affinity chain on the IL-2 receptor is required for T-cell expansion and targeting. This receptor offers the advantage that the CD25 receptor is present only on those active T cells. Theoretically, this agent should affect only those cells that have been activated against a new allograft. This agent is also useful in that it does not lead to the activation of the T cell and therefore potential cytokine release, as is seen with OKT3. These agents have also had several of the murine portions of the molecule replaced with human IgG, thus eliminating much of the nonspecific reactions observed in the heterogeneous antibodies. These agents can be used in the induction phase, but because IL-2 is needed only in the initial activation of T cells, it does not appear to be useful to stop ongoing rejection. Early studies of use as induction agents demonstrated a lower incidence of acute rejection but no long-term graft prolongation in both cardiac and renal allografts.81
Currently it is used as induction therapy with two doses (day 0 and day 4) as part of double- or triple-immunotherapy regimens in adult renal transplant recipients and appears to reduce acute rejection episodes without increasing the incidence of biopsy-proven acute rejection than alemtuzumab induction. Basiliximab is generally associated with a tolerability profile that is similar to that reported with placebo, and better than that reported with ATG.82 The drug does appear to allow for reduced dosage of corticosteroids or calcineurin inhibitors, while maintaining adequate immunosuppression, thereby reducing the potential for adverse effects associated with these co-administered agents. However, its use as an induction agent is usualy limited to those patients who cannot tolerate either alemtuzumab or ATG.
Alemtuzumab
Alemtuzumab is an anti-CD52 antibody that has enjoyed increased use in solid-organ transplantation as a lympho-depleting induction agent. CD52 is expressed on most T and B lymphocytes, NK cells, and monocytes. Alemtuzumab profoundly depletes T cells from peripheral blood for several months with a somewhat reduced effect on B cells, NK cells, and monocytes (in descending order).83–87 It has very minimal effect on CD34+ hematopoietic stem cells. The initial enthusiasm for its application in solid-organ transplantation was based on research by Calne et al., where 33 kidney recipients were treated with alemtuzumab in combination with low-dose cyclosporine. These patients were then compared to the unit’s historical control of patient on standard triple therapy.88,89 The 5-year survival of these two groups was similar and this finding led to the initial suggestion that the use of this agent could lead to “prope” tolerance (graft acceptance with reduced immunosuppression).89 However, later attempts to use the drug alone or in combination with deoxyspergualin led to 100% acute rejection, demonstrating that the drug itself was not tolerogenic. This may be due to the lack of CD52 expression on plasma cells and poor effect on memory T cells. Alemtuzumab combined with a single agent for maintenance (low-dose calcineurin inhibitors) demonstrated safety and efficacy, but has an unacceptably high rate (28%) of early cellular and humoral acute rejection. The drug is currently used as an induction agent with tacrolimus and MMF. Several groups have used it as their induction agent of choice in clinical hand transplants.
Clonal deletion
Clonal deletion is the process in which T cells that express a TCR specific for a certain antigen are eliminated. The deletion of these cells can occur in the thymus (central deletion) or extrathymically in the peripheral tissues (peripheral deletion). The thymus is the major site for the generation of immunocompetent T lymphocytes. T-cell progenitors migrate from the bone marrow to the thymus, where they undergo a well-defined pathway of maturation. Once the T cells express their respective TCRs, they then undergo a process of selection. During this process, cells with low-affinity TCRs are not stimulated to progress; this is called positive selection. T cells in the thymus with a high affinity for self antigen are eliminated by a process called negative selection. When this process is complete, the remaining T cells should be able to recognize self and mount a response only when they encounter foreign antigen. Extrathymic clonal deletion has been described in several experimental models using exogenous antigens90–92 as well as self antigens,93–95 demonstrating that elimination of self-reactive antigens can occur after maturation in the thymus. This mechanism may ensure tolerance to self antigens not expressed in the thymus. Several strategies attempt to influence this process.
The acceptance or tolerance of one’s own tissues first develops in utero along with an immunologic ability to recognize foreign tissue. This phenomenon was successfully exploited by Medawar95a in his original experiments in which strain-specific neonatal rodents were injected with donor cells and went on to accept skin allografts. The production of the tolerant state in an adult can be achieved experimentally by various methods. A combination of total body irradiation to remove mature recipient T cells followed by donor bone marrow infusion before transplantation induces a state of chimerism. (The term chimera is derived from the Greek mythological figure composed of parts from different animals.) The chimeric host then develops an immune system that is tolerant of both donor and self antigens. A further refinement is the use of total lymphoid irradiation; the marrow cavities of long bones are protected during irradiation, thus producing a state of mixed chimerism.96 These animals have gone on to accept donor hearts and kidney allografts. Another method of achieving transplant tolerance involves intrathymic injection of donor cells. These cells survive in the immunologically “privileged” thymus and cause production of maturing T cells that are tolerant of the donor alloantigen.97,98 All of these methods take advantage of the central mechanism of tolerance induction and rely on the phenomenon of clonal deletion.
Anergy
For a T cell to become optimally activated, it requires a second, independent costimulatory signal in addition to the primary signal that is generated through contact between the TCR and the MHC. When T cells are stimulated in the absence of these signals, they can become functionally nonresponsive to repeated stimulation with antigen and are termed anergic.99 Two major costimulatory interactions that take place between a T cell and antigen-presenting cell involve CD28/B7 and CD40L/CD40 pathways.100,101 There has been considerable interest recently in trying to block these pathways. Anergy is not automatically maintained once it is induced, and the continual presence of antigen has been shown to be required to maintain tolerance.102,103 Tolerance relying on anergy may also be a precarious state. It can be broken by infection and inflammation.104,105
The blockade of these second signals uses antibodies (CD40, CTLA4) to specific receptors (CD40R, B27) to induce a peripheral form of tolerance. The concept that the presentation of antigen in certain situations could down-regulate the immune system is not new. Before the discovery of these receptors, previous investigators had noted that donor-specific blood transfusions appeared to increase graft survival, theoretically by presenting MHC antigens in a limited fashion and inducing a state of T-cell anergy rather than activation.106
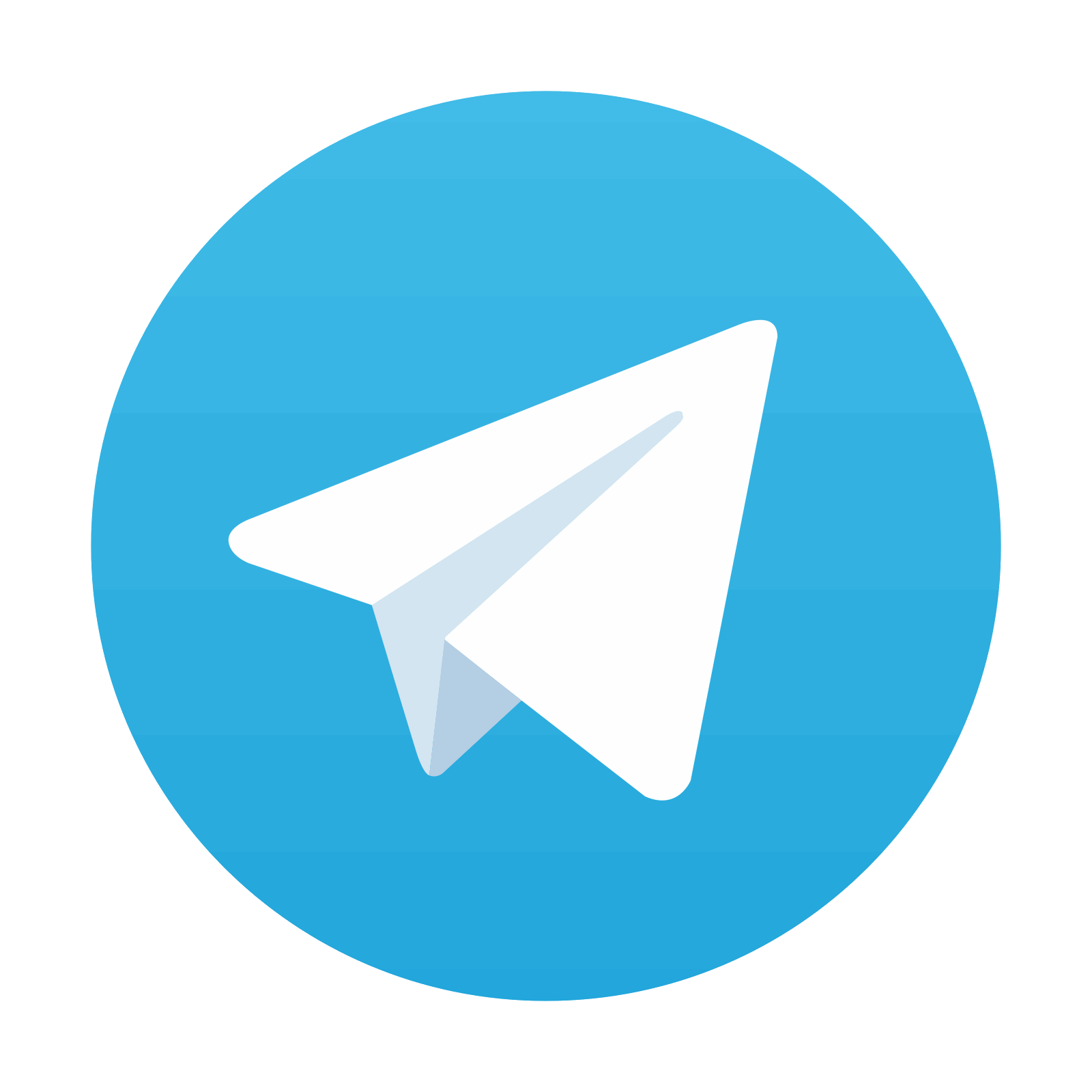
Stay updated, free articles. Join our Telegram channel

Full access? Get Clinical Tree
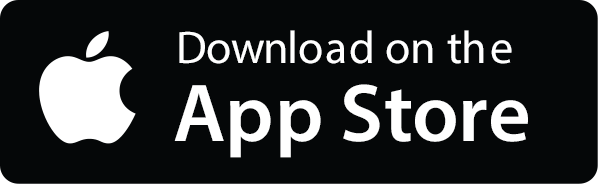
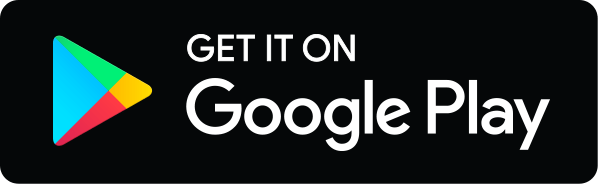