23 The Use of Biologic Tissue and Synthetic Mesh in Urogynecology and Reconstructive Pelvic Surgery
Pelvic organ prolapse and urinary incontinence are common phenomena in women. Eleven percent of the female population will undergo surgery for prolapse or stress incontinence in their lifetime (Olsen et al., 1997). Approximately 30% of these women will need a repeat operation for recurrent prolapse. No standard surgical approach is available to patients who suffer from recurrent pelvic organ prolapse and/or incontinence. Multiple surgical techniques for recurrence, some incorporating surgical implants of synthetic or biologic graft material, have evolved. Some investigators have recommended the use of graft material or prosthesis for recurrent prolapse. Currently, the use of implants, both synthetic and biologic, in reconstructive pelvic surgery is expanding rapidly in spite of a paucity of data supporting their use. Indications for the use of graft implantation in reconstructive pelvic surgery include nonexistent or suboptimal autologous tissue; need to augment weak or absent endopelvic tissue; connective tissue disorder; unavoidable stress on the repair (chronic lifting, chronic obstructive pulmonary disease [COPD], chronic straining to defecate, obesity); need to bridge a space; concern about vaginal length or caliber; and denervated pelvic floor. Some surgeons choose augmentation with graft implants routinely in reconstructive pelvic surgery.
Good evidence supports the use of implantation of synthetic material for abdominal treatment of pelvic organ prolapse, as noted in Chapter 21. Suburethral sling procedures using synthetic materials have been shown to have similar cure rates compared with autologous rectus fascia (ARF) slings. The tension-free vaginal tape (TVT) procedure, a polypropylene mesh midurethral sling, has gained widespread popularity and has proven effectiveness comparable to Burch colposuspension. However, the use of synthetic or biologic implants in transvaginal reconstructive procedures is less clear. Although multiple studies have examined the use of implants for vaginal repair of anterior or posterior compartment prolapse, most are case series or cohort studies, with very few randomized surgical trials. Presently, several manufacturers promote various types of synthetic and biologic materials. No adequate studies comparing implants are known. In this chapter, we will review properties of the ideal graft material, host tissue, and available implants. The surgical procedures that involve mesh implantation, associated clinical results, and complications will also be summarized.
PROPERTIES OF THE IDEAL GRAFT MATERIAL
Authors have proclaimed a need for ideal graft materials since the mid-twentieth century, and the search continues today. The ideal graft would be chemically and physically inert, noncarcinogenic, mechanically strong, sterilizable, not physically modified by body tissue, readily available, inexpensive, and have minimal risk of infection and rejection (Box 23-1). In prolapse and incontinence surgery, the optimal implant, once healed, would restore normal anatomy and function to the vagina and surrounding pelvic organs. It would be biocompatible and, if biodegradable, persist long enough to allow a durable and adequate repair and incorporation of the surrounding tissue. It would be resistant to mechanical stress or shrinkage and easy to work with and be pliable. Other desired criteria include availability in the desired shapes for various operations, prevention of adhesions at visceral surfaces, and a better, or at least equal, response to implantation compared with autologous tissue. Unfortunately, at present, none of the synthetic or biologic tissue implants meet all of these criteria.
To date, no published human articles are known that directly compare synthetic and biologic implants in human subjects, except for two randomized trials: one that compares porcine dermal sling to the TVT procedure; (Arunkalaivanan and Barrington, 2003) and another that compares polypropylene mesh to solvent dehydrated cadaveric fascia lata (Culligan et al., 2005). Literature regarding the physical properties of these materials is confined to animal studies and is rarely comparative, mostly analyzing abdominal wall implantation. Furthermore, few studies are known of the biomechanical properties of graft materials or vaginal wall after implantation. Walter et al. (2003) were successful in using a rabbit vagina model to study graft material tensile strength after implantation.
BIOLOGIC PROPERTIES OF HOST TISSUE
Before discussing properties of various implants, the role of connective tissue in the pelvic floor must be described. Cervigni and Natale (2001) eloquently summarized the biologic properties of host connective tissue. This supportive structure contains fibrous elements (collagen and elastin) and a viscoelastic matrix containing proteoglycans (large polysaccharides attached to proteins). Connective tissue cells determine the biomechanical properties of soft tissue and are embedded in the extracellular matrix, which comprises 20% of the tissue volume. Collagen, a protein produced by fibroblasts, is composed of glycine, proline, and hydroxyproline. Glycine allows collagen to form a tight helix while proline and hydroxyproline form cross-links to stabilize the collagen chains. Tensile strength of tissues is attributed to collagen fibers. Two fibers found in tissue that require strength and flexibility are type I (the most plentiful and strongest) and type III (less common and randomly organized with type I). Elastin and laminin are glycoproteins that are thought to play a role in a tissue’s ability to stretch. Several authors have shown that the metabolism of collagen and/or elastin is disrupted in various pelvic floor disorders.
For the scope of this chapter it is important to summarize the reaction of host tissue to implanted materials. Biocompatibility is defined as the capability of a material to cause a favorable reaction in a living system, thus performing, augmenting, or replacing a natural function in the host. Williams (1973) described four types of soft tissue response: (1) minimal response with a thin layer of fibrosis around the implant, (2) chemical response with severe and chronic inflammatory reaction around the implant, (3) physical response with an inflammatory reaction to certain materials and the presence of giant cells, and (4) necrosis resulting from in situ exothermic polymerization.
Four stages of histologic reaction to graft implantation have been described by Kaupp et al. (1979). They are:
PROPERTIES OF SYNTHETIC MATERIAL
The available absorbable synthetic mesh implants are polyglycolic acid (Dexon, Davis & Geck, American Cyanamid, Danbury, CT) and polyglactin 910 (Vicryl, Ethicon Inc., Somerville, NJ). Absorbable implants may be desirable because they promote postoperative fibroblast activity, are not threatened by infection, do not undergo rejection, and are not known to be harmful to viscera. Lamb et al., (1983) showed that fibrous deposition into polyglactin mesh cannot take place before its absorption. Polyglactin 910 starts to hydrolyze during the third week after implantation and loses the majority of its mechanical value after 30 days. Polyglycolic acid requires 90 days for absorption. Macrophage activation results in mesh absorption and subsequent recycling of byproducts into new collagen fibers (Levasseur et al., 1979). The resultant scar tissue is not as strong as the reinforced tissue, as evidenced in animal studies (Klinge et al., 2001).
Nonabsorbable (permanent) synthetic mesh implants are either monofilament or multifilament (Fig. 23-1 and Table 23-1). The most important physical properties of synthetic implants are pore size and porosity. Some authors have noted small intrafiber pores (interstices of less than 10 μm) as a theoretic disadvantage of multifilament mesh in comparison to monofilament mesh (Brun et al., 1992; Neel, 1983). Most bacteria are less than 1 μm in diameter in comparison to granulocytes and macrophages, which are greater than 10 μm in diameter. The pore size plays an important role in mesh infection prevention and fibrous ingrowth of surrounding tissues. When describing the characteristics of synthetic mesh, Bobyn et al. (1982) listed type of polymer, weave, type of filament, weight, and pore size as important. The authors emphasized that the pore size is the key factor in determining inflammatory response, fibrocollagenous tissue ingrowth, angiogenesis, flexibility (or stiffness), and strength. Best mechanical anchorage with collagen infiltration was noted with pore size between 50 and 200 μm. Pourdeyhimi (1989) noted that exact pore sizes of various meshes cannot be quoted because measurement is technique-dependent.
Marlex reportedly has the highest flexural rigidity when compared with Mersilene, Teflon, and Prolene. Both Marlex and Prolene are monofilament; however, Prolene is more flexible due to its larger pore size. Magnified views of six types of synthetic mesh are displayed in Figure 23-2.
Synthetic mesh has been reclassified as types I to IV, with respect to pore size, as described by Amid (1997). Refer to Table 23-1 for various grafts and associated properties.
All types of synthetic mesh have high tensile strength (more than 50 newtons [N]). Type I mesh acts as a scaffold for tissue ingrowth (fibroblastic cell infiltration). Pore size of greater than 90 μm reduces inflammation, and type I mesh has been associated with reduced rates of infection. Lower inflammatory responses should decrease erosion. Marlex mesh has a smaller pore size and thus has poor tissue ingrowth; therefore, all polypropylene mesh is not type I. Type II and III meshes result in greater foreign body reaction when compared to type I mesh. Klinge et al. (1999) noted that microporous mesh had ongoing perimesh inflammatory reaction at 18 months. Microporous and macroporous multifilament meshes appear to be associated with greater infection rates and poor tissue ingrowth that is attributed to fiber makeup or less surface tension. Microporous multifilament mesh, such as Gore-Tex, is associated with a high infection rate and foreign body reaction.
Implant structure has been eloquently outlined by Cosson et al. (2003). The mechanical properties depend on the structure of the fabric and the thread. These materials can be woven, knitted, or unwoven. Plain, twill, and satin are the three types of woven materials, and their advantages are strength and good memory (Fig. 23-3, A). The disadvantages of woven structures are fraying and poor conformity. Knitted fabrics consist of warp knit, interlock, and circular knit (Fig. 23-3, B). The advantages of knitted materials are flexibility, versatility, and high conformity. The unwoven materials are well-absorbed but have the disadvantages of no conformity and poor visibility. Composite structures have two surfaces, one of which prevents adhesions. The disadvantages of these fabrics are that they are rigid and poorly visible. Implants can have perforations and be molded into various shapes: kidney, umbrella, or a plug. Materials can be structured from monofilament or multifilament fibers, which can be twisted, coated, braided, or double-braided (Fig. 23-4). Cosson et al. (2003) reported mechanical properties of eight types of synthetic implants available in Europe from a study performed in collaboration with the engineering department of Institut Catholique des Arts et Métiers (ICAM); however, they acknowledge that no current recommendations are known regarding desired resistance or elasticity of various implants. Certain materials had differing values based on orientation (lengthwise or widthwise); however, too little is known about how various mechanical properties of mesh contribute to the function and longevity of a reparative procedure.
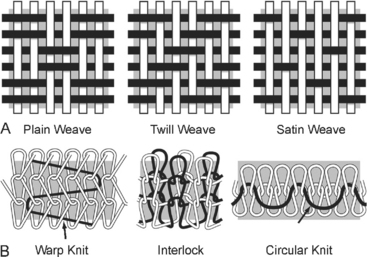
Figure 23-3 A. Woven mesh. B. Knitted fabric.
(Adapted with permission from Cosson M, Debodinance P, Boukerrou M, et al. Mechanical properties of synthetic implants used in the repair of prolapse and urinary incontinence in women: which is the ideal material? Int Urogynecol J 2003;14:169.)
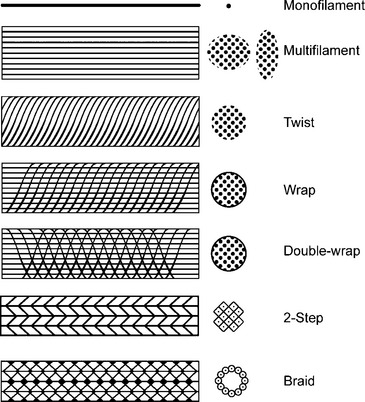
Figure 23-4 Fiber structure.
(Adapted with permission from Cosson M, Debodinance P, Boukerrou M, et al. Mechanical properties of synthetic implants used in the repair of prolapse and urinary incontinence in women: which is the ideal material? Int Urogynecol J 2003;14:169–178.)
A composite mesh has recently been introduced in the United States for use in pelvic reconstructive surgery. This mesh is comprised of a monofilament polypropylene mesh coated with a hydrophilic porcine collagen (Pelvitex, C.R. Bard Inc., Covington, GA). The hydrophilic, absorbable coating protects the viscera from risk of adhesion formation during the first 10 to 14 days after surgery when inflammatory processes peak. Although no data are available regarding the use of this mesh in pelvic floor procedures, adhesion formation has been minimized in abdominal hernia repair (Mutter et al., 2000; Balique et al., 2000). This mesh is also a lightweight monofilament polypropylene thought to maintain strength, increase flexibility, and decrease mesh load on the tissues. A lighter weight and load of mesh may decrease erosion.
PROPERTIES OF BIOLOGIC TISSUE
Autologous grafts can be harvested, but allograft, xenograft, and synthetic materials are widely available, and the morbidity associated with autologous tissue procurement can be undesirable in some patients. Although synthetic materials eliminate the morbidity associated with the donor site, they may have a higher risk of erosion compared to allografts (biologic tissue procured from a source other than the recipient) and xenografts (biologic tissue procured from a source or species foreign to the recipient). Some surgeons routinely use biologic tissue because they consider the host tissue impaired or insufficient for successful reconstruction (although for slings, host tissue is well proven to be effective). The biologic grafts include cadaveric dermis, cadaveric fascia lata (CFL), and collagen matrix laminates—usually from porcine dermis, porcine small intestine submucosa, or bovine pericardium (Fig. 23-5; Table 23-2). Each product differs in the type of processing and sterilization techniques, giving biologic graft materials varying biomechanical and physiologic properties. Various abbreviations for biologic tissue used in this chapter are listed in Box 23-2.
BOX 23-2 BIOLOGIC TISSUE ABBREVIATIONS
ARF | Autologous rectus fascia |
AFL | Autologous fascia lata |
CFL | Cadaveric fascia lata |
DA | Dermal allograft |
SD | Solvent dehydrated |
FD | Freeze-dried |
SIS | Small intestinal submucosa |
BCM | Bioengineered collagen matrix |
Implantation of xenografts has gained popularity in reconstructive pelvic surgery because of concerns regarding availability of human allograft tissue and risk of viral transmission. Porcine dermal allografts (DAs) have been used in cardiothoracic and general surgery for many years. The intended goal of human allograft and xenograft tissue is to provide a scaffold of acellular biocompatible material to allow infiltration and subsequent replacement of graft tissue by the regenerated functional host cells. This acellular material consists of a bioactive and absorbable extracellular tissue matrix consisting of proteins, collagen, elastin, and various growth factors. Acellularity is a desired quality rendering the tissue incapable of eliciting an inflammatory response by its implantation (nonimmunogenic), thus decreasing risk of infection and erosion after graft implantation. However, published data refute that various biomaterials are cell-free. Zheng et al. (2005) recently showed the presence of porcine DNA in small intestinal submucosa (SIS) implants for tendon repair. This cellular characteristic may increase risk of rejection and infection after implantation. Fitzgerald and Brubaker (2000) reported that freeze-dried (FD) and Tutoplast CFL retain donor HLA class I and II antigens. Choe and Bell (2001) proved that FD gamma-irradiated CFL and acellular cadaveric DA tissue contained intact DNA. Hathaway and Choe (2002) used extraction techniques and DNA amplification to show that all four commercially processed human allografts (SD-CFL Tutoplast, Mentor Corp., Santa Barbara, CA; DA, Musculoskeletal Transplant Foundation, Edison, MJ; DA, Regeneration Technologies Inc., Alachua, FL; and cryopreserved CFL Repliform, Life Cell Corp., Woodlands, TX) contained intact genetic material. This is of particular concern because of the theoretical transmission of prions
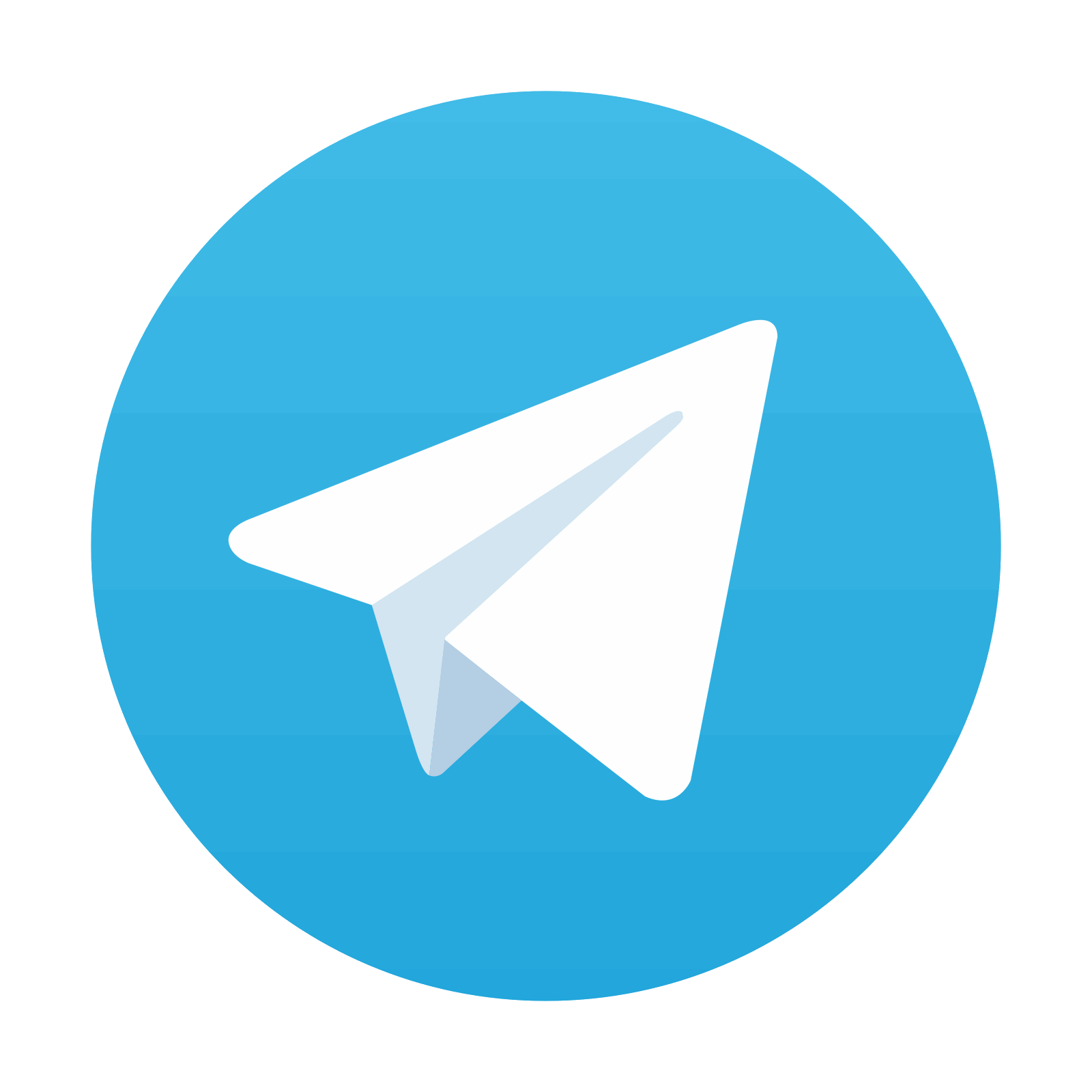
Stay updated, free articles. Join our Telegram channel

Full access? Get Clinical Tree
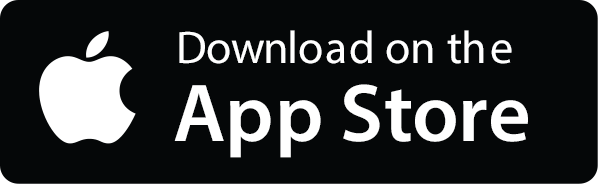
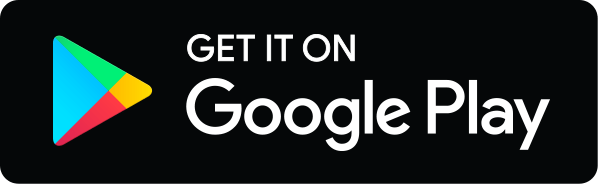