AND FUNCTION
Editor of The Substrates:
Bozena “Bo” B. Michniak-Kohn, Ph.D., M.R.Pharm.S.
Professor of Pharmaceutics
Ernest Mario School of Pharmacy
Director & Founder
Center for Dermal Research (CDR)
NJ Center for Biomaterials, Life Sciences Building
Rutgers—The State University of New Jersey
145, Bevier Road,
Piscataway, NJ 08854
www.michniaklab.org
CONTRIBUTORS:
1. Amy S. Pappert, M.D.
Assistant Professor, Department of Dermatology
UMDNJ—Robert Wood Johnson Medical School
1 World’s Fair Drive, Suite 2400 Somerset, NJ 08873
2. Philip Wertz, Ph.D.
Professor Department of Oral Pathology, Radiology, and Medicine
N450 DSB, Dows Institute
University of Iowa
Iowa City IA 52242
3. Nripen Sharma, Ph.D.
Clinical Scientist/Project Manager/Technical Support
Salvona Technologies LLC
65 Stults Rd., Dayton, NJ 08810
www.salvona.com
4. Anna Langerveld, Ph.D.
President and CEO
Genemarkers
4717 Campus Drive, Suite 1800
Kalamazoo, MI 49008
www.genemarkersllc.com
5. Ada Polla
Alchimie Forever, The Polla Beauty Group
1010 Wisconsin Avenue NW, Suite 201
Washington, DC 20007
www.alchimie-forever.com
6. Barbara Polla, M.D.
Medical Doctor
Alchimie Forever, The Polla Beauty Group
1010 Wisconsin Avenue NW, Suite 201
Washington, DC 20007
7. Anne Pouillot, MS
Director of Science of Alchimie Forever
Alchimie Forever, The Polla Beauty Group
1010 Wisconsin Avenue NW, Suite 201
Washington, DC 20007
8. Karen E. Burke, M.D., Ph.D.
Dermatologist
429 East 52nd Street
New York, New York 10022
9. Gopi Menon, Ph.D.
Senior Science Fellow
Ashland Specialty Ingredients
1361 Alps Road
Wayne, NJ
10. Nava Dayan Ph.D.
President
Dr. Nava Dayan LLC
ABSTRACT
The skin is the organ that forms the border between the organism and the environment. Skin prevents dehydration, stops the penetration of noxious foreign materials and microorganisms, cushions against mechanical shock, helps to maintain a constant body temperature, and transduces incoming stimuli. In order to perform these functions, skin must be maintained in good condition, an important objective for cosmetic formulators. For cosmetic and personal care scientists, whether they are concerned with the improvement of the skin by pharmacology or cosmeceuticals, with the prevention or repair of damage, an understanding of skin structure and function is essential. As such, this section has a primary focus upon these issues.
Further, the impact of light on skin and on skin aging has become so important in cosmetics that it too requires a complete discussion. This chapter is carefully and thoroughly designed to provide a basic knowledge of skin structure and function, thereby forming a sound foundation for the reader to understand key issues and provide stimulation towards the generation of new ideas and novel approaches for new ingredients, addressing the global quest for advanced cosmetic and personal care products.
Part 3.1 Substrate:
The Skin: Structure, Biochemistry and Function
3.1.2 Epidermis and the Keratinizing System
3.1.3 Terminal Differentiation
3.1.4 Pigmentary System of the Skin
3.1.5 Langerhans Cells and Dendritic Cells
3.1.10 Barrier Function and Permeability
a. The Barrier Property of Skin: A Historical Perspective
d. Penetration of Cosmetic Actives Through the
Stratum corneum
e. Penetration Versus Protection
3.1.14 Ultraviolet Radiation-induced Photodamage and Skin Cancer
3.1.15 Gene expression in Skin Care
c. Gene Expression Analysis: A Breakthrough for
Cosmetic Science
The skin is divided into three layers: the epidermis, the dermis, and the subcutaneous tissue. The epidermis is the outermost layer of the skin and consists of a stratified squamous epithelium. Its thickness varies, depending on location, and varies from 0.05 mm to 1.5 mm. The epidermis is made up primarily of keratinocytes, whose basic function is to produce a filamentous protein, keratin, to serve as a protective barrier in combination with various lipid components. These cells also produce several other proteins, for example, cytokines, which play a role in the cutaneous inflammatory response. Separated from the epidermis by the basement membrane, the dermis is composed primarily of the so-called ground substance, which includes glycosaminoglycans (GAGs) and the structural protein collagen. Its thickness also varies with location from 0.3 mm to 3.0 mm. The dermis is divided into two layers: the papillary layer, which interdigitates with the epidermal rete ridges, and the reticular layer, which extends to the subcutaneous tissue. This deepest layer of the skin, also known as the subcutis or hypodermis, is composed primarily of lipocytes with fibrous septae providing structure and support (Fig. 1.1).
Figure 1.1 Diagram of normal human skin
(Reprinted from Harry’s Cosmeticology 8th Ed.2000)
PART 3.1.2 EPIDERMIS AND THE KERATINIZING SYSTEM
The epidermis measures approximately 100 micrometers in thickness and consists of a number of layers: the innermost basal layer (stratum basale or stratum germinativum) which attaches to the basement membrane at the dermoepidermal junction, the prickle layer (stratum spinosum), the granular layer (stratum granulosum), the clear layer (stratum lucidum) and the horny layer (stratum corneum). Together the basal layer and the prickle layer comprise the Malpighian layer. The viable epidermis (the basal, prickle, and granular layers) consists of about 10 biologically active keratinocytes. The stratum corneum is located on top of the viable epidermis. The stratification into the layers is the result of changes in the keratinocytes as they mature and move outward from the basal layer, in which they are continuously formed by the mitosis of self-renewing progenitor cells, which lose their nuclei and are shed from the skin surface (Fig. 1.2).
Figure 1.2. Conversion of individual basal keratinocytes into the flat cells of the stratum corneum (Reprinted from Harry’s Cosmeticology 8th Ed.2000)
The replacement time for the whole epidermis is about 42 days and it is about 14 days for the stratum corneum. While the process of keratinization remains incompletely understood, in normal skin the desquamation of keratinocytes is in equilibrium with the generation of keratinocytes by mitosis of the proliferating cells. The importance of this equilibrium is best understood by studying examples of those skin diseases with abnormal keratinization. Abnormally rapid transformation of basal cells into horny cells of the stratum corneum occurs in psoriasis. Ichthyosis vulgaris, on the other hand, is a genetic disorder that results from abnormal retention of keratinocytes.
Three other cell types are present in the epidermis: melanocytes, the dendritic pigment-synthesizing cells; Langerhans cells, which are colorless and dendritic in form and perform functions related to immunology; and Merkel cells, which are concerned with sensation. The melanocytes and the Merkel cells are confined mainly to the basal layer, while the Langerhans cells are distributed in the basal, spinous, and granular layers.
The basement membrane zone forms the junction between the epidermis and the dermis. Under the electron microscope, it is seen to be composed of four components, listed from the outermost layer: the plasma membrane of the basal keratinocytes, the clear lamina lucida, the electron-dense basal lamina, and the dermal fibrils and bundles of fine filaments [1]. Some details of the components of this junction are provided in a later segment of this section.
The basal layer is a continuous layer of cells that gives rise to all the keratinocytes through mitotic activity. It is usually described as being one cell thick, but in thick normal or in pathological epidermis it appears that mitosis may not only be confined to cells in contact with the basement membrane. A portion of the basal cells is proliferative; these are the cells that differentiate and move up through the epidermis, eventually to become the components of the stratum corneum (essentially “dead” cells that are anuclear) and later desquamate at the top of the skin.
Cells of the stratum basale are cuboidal and have a basophilic cytoplasm and dark-staining, elongated nuclei; under the electron microscope their cytoplasm reveals many ribosomes, mitochondria, and in some cases smooth membranes. In addition, they contain numerous fine tonofilaments, about five nm in diameter, which form the developing cytoskeleton. The basal cells also often contain melanin, transferred from adjacent melanocytes. Intercellular bridges, or desmosomes, connect basal cells with one another and with the overlying squamous cells. Modified desmosomes, or hemidesmosomes, connect the basal cells to the underlying basement membrane zone.
The stratum spinosum is so called because the cells are given a spiny appearance by the numerous desmosomes. These desmosomes, or specialized attachment plates for the cellular tonofilaments, correlate with the intercellular bridges between keratinocytes. The glycocalyx is the intercellular cement between keratinocytes and is composed of glycoproteins. In the upper region of the stratum spinosum, lamellar granules, also known as keratinosomes or Ödland bodies, make their appearance. These are ovoid bodies about 100–500 nm long. In the stratum granulosum they ultimately migrate toward the periphery of the cell and are discharged into the intercellular spaces. Their appearance there correlates with the degradation of keratinocytes. Their lipid contents act to establish a barrier to water loss and may participate in stratum corneum cellular cohesion. Water loss, as is well known, is a critical phenomenon that is measured in the development of new skin care products and relates to some aspects of skin aging.
The thickness of the granular cell layer is usually proportional to the thickness of the stratum corneum. It may be only one cell layer thick in thin skin and up to ten layers in the thicker skin found on the palms and soles of the feet. The cells contain basophilic granules of a material called keratohyalin, a material thought to be responsible for keratin filament aggregation. The “hard” keratins of hair and nail lack these keratohyalin granules.
The stratum lucidum, not seen in most formalin-fixed sections, is located at the deepest portion of the stratum corneum. It can be recognized only in palmar and plantar skin.
In the stratum corneum the keratinocytes have lost their nuclei and virtually all of their cytoplasmic organelles and contents, including the keratohyalin granules. This layer of cells is about 10–15 cells thick (approximately 10 micrometers) and is located on top of the viable epidermis. The corneal cell layer stains eosinophilic with the hematoxylin and eosin stain due to the absence of basophilic nuclei. The cells are flattened and completely filled with keratin. The keratin is in the form of bundles of filaments embedded in an opaque interfilamentous material. They align into disulfide cross-linked macrofibers under the influence of filaggrin, the protein component of the keratohyalin granule responsible for keratin filament aggregation [2].
The structure of the stratum corneum has been compared to that of a brick wall, with the corneocytes as bricks and the intercellular lipids as mortar [3]. Horny cells are continuously shed from the skin surface. Stratum corneum lipids have been characterized and consist of phospholipids, glucosylceramides, cholesterol, cholesterol sulfate, cholesterol esters, ceramides, and fatty acids [4]. The makeup of lipids suggests that hydrophilic lipids are excluded from the stratum corneum to provide a hydrophobic surface on the skin.
PART 3.1.3 TERMINAL DIFFERENTIATION
Terminal differentiation describes the change of the cuboidal keratinocytes (on the basement membrane) to the flat cellular remnants that are shed from the skin surface. The progressive changes of keratinocytes as illustrated in Figure 1.2 are accompanied by biochemical changes, formation of keratins, formation and hydrolytic changes in lipids, loss of water, and cross-linking of cell envelopes. The formation of keratins proceeds from the intermediate filaments present in keratinocytes. Intermediate filaments of more than 50 types are synthesized in human tissues. In skin, two types (I and II) are specifically expressed in epithelial cells. In this classification, acidic keratins (cytokeratins K9–K20) are identified as type I, while the basic keratins (cytokeratins K1–K8) are classified as type II. In skin, the keratins are customarily dimers of one type I and one type II. The fundamental structure of the intermediate filaments includes coil-coil alpha-helical segments bonded to each other by nonhelical linker segments. Both ends of the rod-shaped filament are terminated by peptides. The exact modus of attachment of these filaments to each other to form the keratins within the keratinocytes is still under investigation. As noted, epithelial cytoskeletal filaments generally belong to one of two keratin (acidic or neutral-basic) groups ranging in molecular weight from about 40 to 70 kDa. Fillaggrin has been identified as one of the keratohyalin proteins forms in differentiating keratinocytes. Filaggrin is involved in the aggregation of keratin filaments to form the keratins found in mature keratinocytes or corneal cells. After filaggrin has served its function as a matrix between intermediate filaments, it is hydrolyzed enzymatically to create various free amino acids that form part of the natural moisturizing factor (NMF).
The hydrolytic changes of the epidermal lipids are also controlled by keratinocytes, which discharge lipids into the intercellular space after forming the lamellar granules. These lipids are distinctly different from the sebaceous lipids secreted by the sebaceous glands. In the process of terminal differentiation, which requires about three to four weeks, the basal keratinocyte generates a remarkable set of complex lipids (e.g., ceramides). During the cells’ passage outward, these lipids are modified (become more hydrophobic) to contribute to the biphasic structure commonly called stratum corneum.
During their ascent to the skin surface, the keratinocytes shrink, primarily through loss of water. The fate of this water is not known, but one may safely assume that it becomes part of the evaporating water generally described as trans-epidermal water loss. The loss of water during the maturation of keratinocytes is an important phenomenon that must be considered in studies of skin moisture levels (Fig. 1.3) and the impact of ingredients used in cosmetic and personal care products to improve the skin’s ability to retain its moisture. The level of water in the basal layer is about that found in internal tissues, that is, about 80–85%. The water level drops stepwise to about 35% at the border between the stratum granulosum and the stratum corneum. The water level in the topmost layers of the skin is variable and is under the control of the environment and the evaporative flux from lower skin layers.
Figure 1.3. Water concentration profile in epidermal layers [SC—stratum corneum; GR—stratum granulosum; SP—stratum spinosum; B—basal layer (stratum germinativum. (Reprinted from Harry’s Cosmeticology 8th Ed.2000)
Finally, the proteins in the cell membranes of the maturing keratinocytes undergo drastic changes due to cross-linking. This provides the terminally differentiated corneal cell with a rigid cell envelope that is chemically resistant and acts as a protective coating. The most important enzymes that play a role in this process are transglutaminases that catalyze epsilon- (gamma-glutamyl) lysine crosslinking. Involucrin is the primary cytoplasmic precursor to the protein making up the cell envelope. Other cross-linked proteins are present and have been identified—for example, loricrin.
Biologists have further identified some layers within the stratum corneum. The desquamating layer at the surface is frequently called the stratum corneum disjunction, while the layer below it is known as stratum corneum conjunction. To complete this highly simplified discussion of the skin, it is important to note that the latter is frequently identified as viable epidermis. Cells from this layer can be cultured and are commonly used to study the release of cytokines and the like as well as the impact of drugs and/or cosmetic ingredients. In contrast, the nonviable epidermis includes only the dead cells of the stratum corneum.
Before leaving the life history of the epidermis, the process of differentiation should be considered as proceeding from the inside to the exterior. The outward movement of biological debris, water, and lipids directly opposes human efforts to drive ingredients/drugs into and through the skin unless special efforts are made to create molecules that are shaped and manipulated to permeate.
PART 3.1.4 PIGMENTARY SYSTEM OF THE SKIN
Melanocytes are dendritic cells that produce and secrete melanosomes, which contain melanin. Melanin is the major determinant of skin color. The number of melanocytes in the epidermis is the same—regardless of skin color; it is rather the number and size of melanosomes produced that determine the color of one’s skin. Melanosomes in dark skin are nonaggregated, whereas they are smaller and form membrane-bound complexes in light skin. Melanocytes are derived from the neural crest in the embryo and are seen in the basal layer of the epidermis by the eighth week of gestation. They differ from the other cells of the stratum basale by the possession of dendritic processes, by which they transfer pigment to a group of keratinocytes; the whole forming the “epidermal melanin unit” [5]. Typically each melanocyte is associated with about 36–40 keratinocytes in the human epidermis. Melanocytes have no desmosomes and thus, when stained with hematoxylin and eosin, appear to have a halo due to the separation from adjacent keratinocytes. The concentration of melanocytes, though, does vary in different areas of the skin, with the highest concentration on the face and the male genitalia and the lowest concentration on the trunk.
The characteristic feature of melanocytes is a special cytoplasmic organelle known as a melanosome in which the melanin is formed by the action of the enzyme tyrosinase. The melanosomes arise as spherical, membrane-bound vesicles in the zone of the Golgi apparatus and eventually appear as densely pigmented granules [6].
Melanins consist of two kinds of quinoid polymers: pheomelanins and eumelanins. Pheomelanins are yellow or red in color, and eumelanins produce the brown or black color. Both are formed by the same initial steps, which involve oxidation of tyrosine to 3,4-dihydroxyphenylalanine (DOPA) and its dehydrogenation to DOPAquinone. The formation of eumelanins then involves several further steps to produce indole-5,6-quinone, which polymerizes and becomes linked to protein. It is now believed that eumelanin is not a homopolymer composed solely of indole-5,6-quinone units but rather a poikilopolymer that includes several intermediates. Pheomelanins are formed by a different route. The DOPA-quinone interacts with cysteine to form 5-S- and 2-S-cysteinyldopa, and these isomers are further oxidized to a series of intermediates that then polymerize [7].
The formation of melanin depends on the generation of free radical species. The biochemical pathways leading to the formation of melanin pigments in vivo were described by Raper [8] and more recently by Prota [9, 10]. This information can be found in most textbooks of biochemistry and is not repeated here. Once formed, melanin has been identified as a (stable?) free radical that can react with superoxide.
The significance of melanin as a purported photoprotectant and the response of skin to ultraviolet (UV) irradiation are critically important to skin appearance and health, especially with regard to sun exposure.
PART 3.1.5 LANGERHANS CELLS AND DENDRITIC CELLS
The skin provides the first line of immune surveillance against biologic pathogens and chemical irritants through a network of sophisticated and specialized antigen-presenting cells. These include Langerhans cells (LCs) in the epidermis and several distinct types of interstitial dendritic cells (DCs) in the dermis. LCs and DCs are the sentinels that recognize certain antigens as well as recognizing tumors. For example, they recognize foreign environmental antigens such as irritating chemicals (thereby inducing contact hypersensitivity), microorganisms (thus protecting through antimicrobial immunity). They also recognize tumors (providing antitumor immunity) and allogeneic markers (causing skin graft rejection).
The extended dendrites of these unique cells form a network within the epidermis and in the dermis they create an immunologic barrier primed to recognize, capture, and process pathogens and foreign antigens for presentation to naïve and memory T-lymphocytes, thereby initiating and then modulating the subsequent immune response. Furthermore, the LCs and DCs play a significant role in inducing peripheral immunologic tolerance to innocuous environmental proteins and to self-antigens, thus helping to prevent autoimmune disease.
LCs are the epidermal resident dendritic cell population. They make up about 3–5% of the suprabasal, stratum spinosum layer of the epidermis [11] including the mucosal epithelia lining the ocular oral and vaginal surfaces [12]. LCs are scattered without desmosomal connections between keratinocytes. In light microscopic sections, LCs cannot be detected by routine histologic staining, but only by staining with gold chloride (for human LCs).
LCs are characteristically stellate with extensive dendritic fibers stretching in all directions, forming a network within the stratum spinosum layer. When viewed by phase contrast microscopy, the DCs extend delicate flower-like projections that bend, retract, and reextend. This shape and motility enable them to accomplish their function of capturing antigens and of selecting and processing the antigens for specific T-cells [13]. By means of high-powered electron microscopy, LCs have been shown to be a folded nucleus and are identified by their distinctive, intracytoplasmic Birbeck granules that (when mature) are rod shaped with a vacuole at one end, resembling a tennis racquet. Antigen capture seems to be a consequence of binding to langerin, a calcium-dependent lectin that specifically binds mannose as well as other sugars and is found only in LCs and some dermal DCs [14]. This binding actually induces formation of Birbeck granules and then routes the antigen into the Birbeck granules for further processing.
Langerin is a recognition receptor for carbohydrates on the surfaces of pathogens that routes antigens to different cellular compartments involved in antigen presentation in order to promote recognition by different specific T-cells [15].
In addition to LCs, the skin has at least four other subclasses of DCs in the dermis. The characterization of these DCs is quite complex because DCs can arise from several types of progenitor cells, and different functional phenotypes of DCs can be generated from the same precursor cell (as determined by function required, generating a sequential encounter with specific cytokines). Furthermore, as the LCs and DCs encounter a foreign antigen and migrate, they mature, and their surface markers as well as their functional interactions change. Thus, the steady-state population is different from the inflammatory response populations.
Langerhans cell function is impaired by UVB radiation, resulting in a decrease in the antigen-presenting capacity and in the production of cytokines. This UV suppression leads not only to adverse consequences by impairing endogenous antimicrobial activity and tumor recognition and suppression, but also to possible advantageous therapeutic treatments for skin allergy and inflammatory disease.
The dermis is a tough and resilient tissue that cushions the body against mechanical injury and provides nutrients to the epidermis and cutaneous appendages [16]. It consists of an association of protein fibers within an amorphous ground substance containing glycosaminoglycans (GAGs), previously known as mucopolysaccharides. There are few cells in this matrix; most of them are fibroblasts, which secrete the dermal constituents. Fibroblasts are derived from the mesenchyme. The mast cell, also of mesenchymal origin, houses granules that contain heparin, histamine, and other active substances. The mast cell is an active participant in skin inflammation and irritation, as well as in several other skin disorders. The dermis also houses blood, contains lymphatic and nervous systems, and surrounds the invaginated epidermal appendages. The GAGs in the dermis can hold copious amounts of water and tend to surround the other constitutents of the matrix. Together with the fibrous portion of the matrix, these substances account for the skin’s flexibility and resistance to deformation.
The major fibrous constituent of the dermis, accounting for 75% of the dry weight and 18–30% of the volume, is collagen [17]. Under the light microscope, collagen fibers appear as eosinophilic branching wavy bands. Collagen fibers are loosely arranged in the papillary dermis and are tightly bundled in the reticular dermis. Pilosebaceous units, eccrine glands, and apocrine and dermal blood vessels are surrounded by a thin meshwork of collagen. Collagen fibers display characteristic cross-striations with a periodicity of 60–70 nm. Collagen is rich in the amino acids hydroxyproline, hydroxylysine, and glycine. The fibroblasts produce a precursor known as procollagen, which includes 300–400 additional amino acids in each of its chains; these extensions are removed after secretion, which results in the conversion to the collagen molecule. Collagen fibrils form by the association of collagen molecules. Vitamin C and copper are two of several cofactors required in the biosynthesis of collagen. Collagen production is a dynamic process that involves continual synthesis by fibroblasts and degradation by collagenases.
Elastic fibers make up only 4% of the dry weight and 1% of the volume of the dermis. They are delicate, straight, freely branching fibers that prove very resilient. These fibers are thicker in the lower portion of the dermis and become thinner as they approach the epidermis. Elastin differs from collagen not only structurally but also chemically. Desmosine is an amino acid unique to elastin. About 0.4% of the dry weight of the dermis is made up of fine branching fibers that, unlike collagen, stain black with silver stains and are known as reticulin. Their axial periodicity is identical to that of collagen. Reticulin fibers in the papillary dermis serve to anchor the basal lamina [17].
The amorphous ground substance in which the fibers and cells lie contains acidic GAGs. In dermis the major forms are hyaluronic acid, chondroitin sulfate, and dermatan sulfate.
The skin is supplied with both sensory and autonomic nerves. It is innervated with about one million afferent nerve fibers; most terminate in the face and extremities, and relatively few supply the back. The sensory nerves, unlike autonomic nerves, possess a myelin sheath up to their terminal ramifications.
The papillary dermis is heavily innervated with unmyelinated nerve fibers that transmit the sensations of temperature, pain, and pruritus. Three types of special nerve end organs also exist in the dermis. Vater-Pacini corpuscles are large end-organs that are located in the deeper portions of the dermis and subcutis and mediate a sense of pressure. They measure up to 1 mm in diameter and have their greatest concentration at the tips of the fingers and toes. A few are present in the nipple and anogenital regions. Meissner corpuscles are located in the dermal papillae and mediate the sense of touch. They occur only on the ventral aspects of the hands and feet and are most concentrated in the fingertips. The mucocutaneous end-organs are found in the papillary dermis of the modified hairless skin of the glans, the prepuce, the clitoris, the labia minora, the perianal region, and the vermilion border. The autonomic nervous system supplies fibers to the arrector pili muscles, the blood vessels, and the eccrine and apocrine glands. The sebaceous glands are not innervated, and their functioning depends on endocrine stimuli. The autonomic nervous system controls vasoconstriction, contraction of the arrector pili muscles, and glandular secretion.
The dermal vasculature consists of intercommunicating plexuses. The subpapillary plexus lies within the papillary dermis and runs parallel to the epidermis to furnish a supply of capillaries, arterioles, and venules to the dermal papillae. The deeper plexuses are composed of larger vessels and surround hair follicles and eccrine glands. The dermal lymphatics are associated with the vascular plexuses.
Smooth muscle occurs in the skin as the arrector pili muscles of the hair to pull the follicle upward with contraction. There are also smooth muscles fibers in the scrotum and the areolas. Striated muscle occurs in the skin within the neck as the platysmas and in the muscles of expression of the face. Special aggregates of smooth muscles are found between the arterioles and the venules in the skin. These serve to shunt blood from the arterial to the venous system directly and thus bypass the capillary system.
PART 3.1.7 APPENDAGEAL STRUCTURES
Humans have several million eccrine sweat glands distributed over most skin sites, but they are more concentrated in the axilla, forehead, palms, and soles. In some areas they number as many as 600 glands per square cm. Eccrine sweat glands are the most numerous skin appendages and are responsible for the production of sweat. They have a cylindrical spiral duct lined with epidermal cells extending from their visible opening in the epidermis down into the deep dermis, where the duct becomes coiled and convoluted into a ball [18]. This secretory coil manufactures the odorless sweat, which rises up the duct to be released on the skin surface. It is thought that the duct of the gland has the ability to modify the sweat as it flows upward by removing salts or water.
The sweat glands control both body temperature and excretion, and they are under the control of the cholinergic nervous system. The evaporation of sweat has a cooling effect. The glands respond to environmental temperature but also to other stimuli, such as UV light, emotional stress, and increases in body temperature. On the palms and soles, the secretion from the glands serves to increase surface friction.
Sweating appears to involve activation of myoepithelial cells, which line the ducts of the glands. Although sweating is considered to be a continuous process, it seems that sweat is ejected in small bursts, suggesting a peristaltic action by the ducts. The composition of eccrine sweat is similar to that of plasma, although more dilute, and was documented about 40 years ago. It includes, in decreasing relative concentration (mg %): Cl– (320), Na+ (200), lactic acid (35), K+ (20), urea (15), ammonia (5), Ca++ (2), glucose (2), Mg++ (1), amino acid (1), and creatinine (0.3).
The apocrine glands are tubular glands attached to the hair follicle and, like the sebaceous glands, develop in association with it [19]. Although rudiments are found covering the entire surface of the fetus, the glands become canalized and functional almost exclusively in selective areas including: the axillae, the anogenital regions, the areola, the external auditory canal, and the eyelids. In humans, apocrine gland secretions are milky and viscous but without odor. Odor production is related to bacterial action on apocrine secretions at the skin surface.
After puberty, apocrine gland secretion is in response to emotive stimuli. Adrenergic nerves control secretory activity, in contrast to the cholinergic control of eccrine function. The function of the glands in the human species has been much debated; studies have demonstrated that the apocrine skin glands of mammals produce various glycoconjugates and antimicrobial substances, which may serve as a nonspecific defense on the skin surface [20]. In other mammals the glands serve a sexual function.
Sebaceous glands [18] secrete sebum, which forms the majority of the lipid that covers the skin and hair. They are found in all areas of human skin except the palms, soles, and dorsum of the feet. Sebaceous glands are usually associated with hair follicles, except for those on the nipples, areola, and labia minora. The greatest concentrations (reportedly as high as 400–900/cm) are found on the scalp, face, upper chest, and shoulders.
The glands are holocrine and thus form their secretion by decomposition of their cells. New cells are formed continually from the lining of the gland by cell division to replace those lost. No motor innervation has been demonstrated in humans. During the generation of sebum, cells at the periphery of the lobule undergo division. As the daughter cell moves toward the center of the lobule, it synthesizes lipids. As the sebum accumulates, the cell increases in volume as much as 150-fold. When synthesis is complete cell rupture occurs. This process from cell division to rupture requires approximately 14 days. The relatively long delay must be taken into consideration when designing drugs, cosmeceuticals, and therapies aimed at altering sebum.
Sebaceous gland activity is under hormonal control. It is stimulated by androgens of both gonadal and adrenal origin. In human males the glands are minute during the prepubertal period but undergo vast enlargement at puberty, when their output increases more than fivefold. Eunuchs secrete about half as much sebum as normal males but substantially more than boys; it seems that the secretion is dependent on adrenal androgens. Adult women secrete only a little less than men; their sebaceous activity appears to be maintained by androgens from the ovary and the adrenal cortex. Estrogens and anti-androgens, such as cyproterone acetate, inhibit sebaceous secretion in humans. On the other hand, relatively small doses of potent androgens can cause enlargement of the glands and an increase in sebum production.
Human sebum is composed of triglycerides (57.5%), wax esters (26.0%), squalene (12.0%), free fatty acids (10%), and, to a minor extent, cholesterol and cholesterol esters. Epidermally derived lipids differ in lacking wax esters and squalene and having much higher proportions of cholesterol esters and cholesterol. There are marked differences in sebum composition among species. The origins of sebaceous lipids and their composition are different from those of the epidermal lipids [21]. The purpose of sebum is not entirely understood, but sebum has been shown to have thermoregulatory functions, and plays a role in the regulation of immunological functions and inflammatory processes. In addition, sebum contains lipids with antimicrobial activity [22]. While excessive sebum production has been associated with the development of acne vulgaris, lack of sebum production in prepubertal children is not associated with any skin abnormalities.
The hair follicle is quite variable, depending on its location. In adults, deep terminal hairs are found on the scalp and male beard area. Hair on the extremities and trunk is located more superficially in the skin. Vellus hairs are present on the female face and on the nonbearded areas of the male face. Lanugo is soft fine hair that covers the fetus and is shed prior to birth.
In general, the cross-sectional shape of terminal Caucasian scalp hair is round and somewhat curly; African American scalp hair is oval, sometimes flattened, and usually kinky; hair in Asians is round in cross-section and straight. These relationships do not apply to pubic hair, beard hair, and eyelashes, which have similar features in all races and are typically oval.
Hair color is due to the distribution of melanosomes within the hair shaft produced by melanocytes in the hair bulb. These are transferred to cells of the hair matrix similar to the transfer from melanocytes to keratinocyte in the epidermis. Three types of melanosomes are present in hair. Eumelanins are seen in dark hair, and pheomelanins predominate in blond hair. The intensity of color is related to the number of fully melanized melanosomes produced. Gray hair and white hair are due to a decreased number of melanocytes that produce fewer melanosomes.
Despite the vast body of knowledge regarding the anatomy, biology, and function of hair, humans are still unable to induce hair regrowth in the many disorders that result in hair loss, or to induce permanent hair removal in states of excessive hair. The cost of hair care in terms of time and money spent is huge in many cultures, and the psychological impact of hair disorders should not be underestimated.
The nail plate is composed of keratinized cells that originate in the nail matrix. As discussed previously, there are no keratohyalin granules. The proximal nail fold forms the cuticle. The nailbed does not contribute to the development of the nail plate but serves as a base for the adherent keratinous nail plate.
PART 3.1.10 BARRIER FUNCTION AND PERMEABILITY
For the cosmetic industry, by and large, the skin has been synonymous with the epidermal permeability barrier, located in the stratum corneum. This is a somewhat restricted view, but nevertheless remains in place due to the fact that skin moisturization—a function that is of prime interest to the industry—is dependent on a functional permeability barrier. As the saying goes, a good moisturizer is not necessarily a barrier product, but all barrier products are good moisturizers. The two-compartment, “brick and mortar” organization of the healthy stratum corneum, with its organized lipid membranes, do “lock in” moisture and prevent dry, chapped skin. However, the significance of other types of barriers in the skin, i.e., UV barrier, antioxidant, and antimicrobial defenses have all begun to be appreciated and emphasized, as evidenced by the proliferation of active ingredients targeted to them. This growing recognition, in concert with an improved understanding of the other areas of the skin, is critically important to novel thinking leading to new functional ingredients for this important cosmetic and personal care category.
a. The Barrier Property of Skin: A Historical Perspective
The barrier property of skin against influx and efflux of water was acknowledged as early as the 1800s, but the conclusive evidence was provided by the work of Irvin Blank and Scheuplein, who convincingly demonstrated its location in the stratum corneum (horny layer) [23]. The barrier properties are of interest to different scientific disciplines and industry; especially the pharmaceutical and personal care sectors that are interested in delivering drugs transdermally or active ingredients for topical delivery to improve the skin conditions (anti-aging, moisturizers, skin lighteners, etc.) respectively. For the dermatologists, inherited and acquired skin diseases such as various types of ichthyosis, atopic dermatitis, and psoriasis all have a compelling barrier dysfunction associated with it [24]. Extensive damage to the barrier, as in third-degree burns, can be lethal; attesting to the significance of a functional permeability barrier to prevent life-threatening dehydration of the body.
Details of barrier (and stratum corneum) formation, its structural complexity, and chemical characterization
Early investigators interpreted what appeared as a “basket weave” structure of the SC in routine histological studies as an indication that it is a passively occluding structure on the skin surface—a more or less uniform membrane functioning like “Saran wrap.” Investigators who followed them have dispelled the notion of the SC as a “basket weave” structure passively occluding the surface. A battery of investigative techniques (electron microscopy, laser confocal microscopy, two-photon microscopy, as well as biochemical characterization of lipids and proteins) have led to the understanding first of a “brick and mortar” organization of SC, and a later concept of the SC as being a composite material with several characteristics of a “smart” material [24]. In this latest model, the bulk of the SC is made up of corneocytes, which are the “bricks.”
Before discussing the secretion of the lamellar lipids (pro-barrier lipids) and the further processing of the lipids in the SC extracellular domains (the “mortar”), a brief description of a multiplicity of the phenomena that occur in the SC is warranted.
These phenomena include: a description of the keratinocyte differentiation, synthesis of proteins (keratins, filaggrin, involucrin, loricrin, desmosomal components, enzymes involved in cornification) and lipids (which are packaged with lipid metabolizing enzymes and anti-microbial peptides within secretory organelles) in the form of lamellar bodies (Odland bodies, keratinosomes).
Keratinocytes (keratinizing epithelia) account for about 95% of the stratified epidermis (other cell types are melanocytes, Langerhans cells, Merkel cells), and based on its position within the strata as well as its progressive differentiation, is designated as belonging to several layers including: the basal layer (stratum basale), the spinous layer (stratum spinosum), the granular layer (stratum granulosum), and the cornified layer (stratum corneum
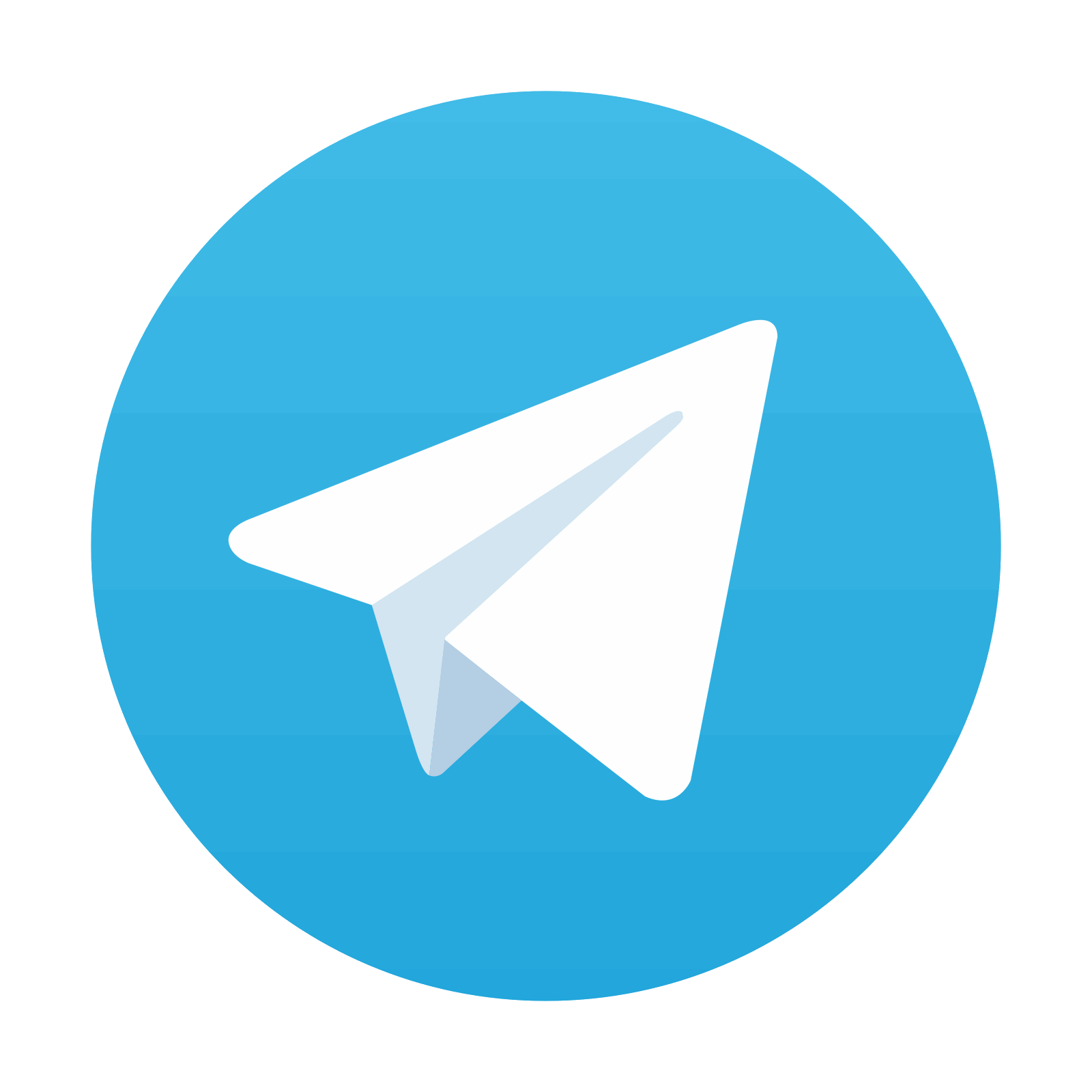
Stay updated, free articles. Join our Telegram channel

Full access? Get Clinical Tree
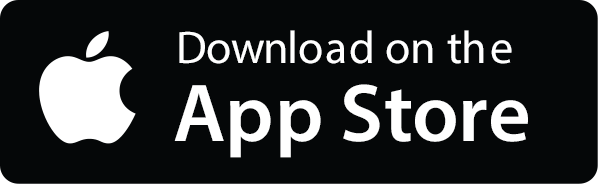
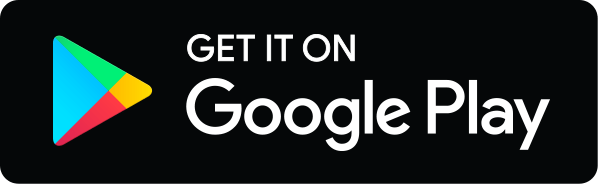