hair during water-setting
c. Temporary shapes induced by long-term deformations
d. Limitations of water-setting
3.3.4.4 Changes in hair shape by hot iron treatments
c. Mechanical action of the hot iron
3.3.4.5 Physical processes taking place inside hair during hot ironing
b. Phase changes and transitions in hair
c. The mechanisms of water and hot iron setting are different.
e. Heat transfer from hot iron to hair
f. Unwanted consequences of friction and rising
hair temperature above Tg
3.3.4.6 Hair shape changes induced by permanent waving solutions
b. Physical and chemical processes taking place inside
hair during permanent waving
3.3.4.7 Hair shape changes induced by alkaline relaxers
b. Physical and chemical processes taking place
inside hair during alkaline straightening
c. shapeless hair a new straight shape
3.3.4.8 Hair shape changes induced during grooming practices
a. Static vs. dynamic changes in hair shape
b. Friction vs. bending forces in dynamic changes in hair shape
3.3.4.9 Hair volume and changes in hair shape
b. Main challenges in creating volume
c. Back-combing and “static fly away”
3.3.4.10 Frizz in hair and changes in shape
3.3.4.11 Body in hair and changes in hair shape
3.3.4.12 Hair shape changes induced by styling polymers
3.3.4.13 Role of styling polymers in changing hair shape
a. Temporary setting of hair with aerosols
b. Temporary setting of hair with gels and mousses
c. Mechanical properties of welding seams and spots
d. Effect of polymer glass transition temperature
(Tg) on styling
e. Adhesive strength at the polymer/hair interface
The key physical properties and mechanistic events related to changes in hair shape can be better understood when we recall that hair is a biopolymer that shares many of the physical and chemical features possessed by synthetic polymers. In fact, we can learn a great deal about hair’s mechanical behavior by comparing its properties with those of synthetic polymers having a similar structure. For instance, synthetic semi-crystalline polymers, like hair, possess crystalline melting and amorphous phase glass transition temperatures (2–4).
Furthermore, the composition of hair’s architecture is such that it can exhibit two types of physical behavior.
- ● One type of behavior is related to the hydrophilic structure of the globular proteins in the amorphous phase. The protein hydrophilic groups in this structure are capable of forming hydrogen bonds with water. This aspect of the structure makes it highly water swellable and susceptible to plasticization by moisture. It is responsible for the viscoelastic and softening effects produced in hair by water; Max Feughelman refers to this aspect as the “gel structure of the amorphous phase” (6).
- ● The other type of physical behavior stems from the non-gel structure of the intermediate filaments (IFPs). This structural aspect is comprised of an amorphous network of hydrophobic, and stiffer protein segments. These are covalently cross-linked by disulfide bonds, as well as an ordered arrangement of crystalline proteins regions, which are responsible for both shape stabilization and hair strength.
The entire amorphous phase acts mechanically in parallel with the crystalline phase and gives rise to the unique combination of viscoelasticity and strength typical of hair. This behavior is also found in semi-crystalline polymers and, incidentally, there is a remarkable similarity in the architecture and properties of hair, and a particular type of cross-linked semi-crystalline polymers, namely; those that have been synthesized to behave as shape memory polymers (SMP) (7). These polymers, like hair, also exhibit two and even more types of physical behaviors. These multiple types of behavior result in most cases from the various chemical groups or structures grafted onto main polymer chains introduced with the purpose of storing various temporary shapes (8–11).
As will be discussed later in this chapter, hair, with both its amorphous and crystalline structures, can also act as a shape memory polymer (SMP) and the mechanistic aspects related to changes in its shape can be better understood by using concepts from this field of polymer science. We would point out here that this cross-fertilization of ideas, or technology transfer, is an excellent approach for hair product developers to employ when seeking new ideas.
3.3.4.2 THE SHAPE MEMORY PROPERTIES OF HAIR
a. Definition of shape memory materials
In the scientific literature, C. Liu and C.B. Chun (8) define a shape memory material as follows:
“Shape-memory materials are those materials that have the ability to ‘memorize’ a macroscopic (permanent) shape, be manipulated and ‘fixed’ to a temporary and dormant shape under specific conditions of temperature and stress, and then, later, relax to the original, stress-free condition under thermal, electrical, or environmental command. This relaxation is associated with elastic deformation stored during prior manipulation” (2).
Currently, the most rapidly growing area in the field of shape memory materials are shape memory polymers (SMPs), and they can be either purely amorphous or semi-crystalline (12–14). Figure 1 shows a sequence of captions displaying a shape memory polymer changing its shape from a coiled temporary to a straight permanent shape after this event is triggered by elevating its temperature (15). Shape memory polymers can store temporary shapes and can recover their permanent shape by a suitable stimulus, either electrical, chemical, or temperature.
Figure 1) Transition of shape from temporary (spiral) to permanent (rod) of a polymer synthesized from poly-(ε-caprolactone) dimethylacrylate and butyl acrylate (15).
Various scientists working in this field have already listed the main physical requirements for a semi-crystalline polymer to display shape memory properties. According to C. Liu et al., these are as follows (8):
- 1) a relatively sharp glass transition and/or a high crystalline phase melting temperature that can be used to promptly fix the secondary shape at low temperatures and trigger shape recovery at high temperatures,
- 2) superelasticity (low loss modulus, high deformability) above the transition temperature that leads the shape recovery and avoids residual strain (permanent deformation) and,
- 3) complete and rapid fixing of the temporary shape by immobilizing polymeric chains without creep thereafter.
Synthetic polymers with shape memory properties consist primarily of two main features in their chain architecture. These are:
- ● A primary polymer network with network points, or cross-links that serve as a memory mechanism for the generation of a permanent shape, and
- ● A structure of secondary groups attached to the main network that serves as memory mechanism for the formation of temporary shapes (8).
These groups can be pendant functional moieties, grafted chains, polymer blocks, interpenetrating networks, or crystalline domains; they act as switches to release the temporary shape when activated by suitable external stimulus (see Figure 1) (7). Moisture, heat, light, and electric fields are examples of external stimulus that can release temporary shapes stored in SMPs with pendant groups or structures sensitive to them (see Figure 2) (8, 9).
Figure 2) Molecular mechanisms of shape memory polymers according to C. Liu et al. (8)
b. Hair is a biopolymer with shape memory properties.
In the scientific literature, biopolymers such as silk and collagen have also been reported to have shape memory properties, and thus, they have been classified as shape memory materials (16, 17). Hair is a biopolymer that also behaves as a shape memory polymer. Clear manifestations of this behavior and properties are the following:
- 1) hair has a permanent shape,
- 2) its shape can be changed either temporarily or semi-permanently, and
- 3) it can “remember” or reverse to its permanent shape when treated appropriately.
Beauticians and cosmetic scientists, aware of these properties, have been creating fashionable hairstyles for years that can be reversed when desired. An example of hair’s ability to recover its permanent shape can be seen in Figure 3, where it is shown that a curly temporary water-setting in a woman’s hair (Figure 3a) can return to its original straight shape after washing the hair (Figure 3b). Also, Figure 4 displays a sequence of micrographs of a hair fiber recovering its permanent shape from temporary straight to its curly permanent shape.
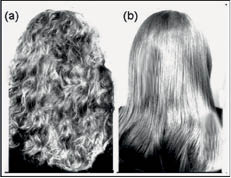
Figure 3) Captions of hair in a woman before (a) and after (b) it recovers its straight shape from a water temporary setting (18).
Figure 4) Captions showing sequence of a single hair fiber recovering its permanent shape from a straight temporary shape to a curly shape after water immersion (18).
As in the case of synthetic polymers, the permanent and temporary shape memory sites in hair reside in its crystalline and amorphous phases. In the case of hair, these polymeric entities are of natural origin and located in the macro-fibrils of the cortical cells. These can be listed as follows:
- a) a protein crystalline phase forming intermediate filaments which serves as permanent shape memory and is also capable of storing a temporary shape when its crystalline structure unfolds or when it denatures above its melting temperature Tm.
- b) a disulfide bond cross-linked protein amorphous phase capable of storing temporary shapes when it becomes soft at the hair’s glass transition temperature Tg, or when it undergoes disulfide bond interchange. This structure also contributes to the stability of the crystalline phase, and
- c) an extended structure of hydrophilic protein groups located at the surface of globular proteins in the amorphous phase. Its structure forms a gel, is sensitive to moisture, and is also capable of storing a temporary shape.
c. Temporary shape memory in hair
The most common temporary or secondary shapes in hair are stored by physical means. The position of storage is in the protein regions with high levels of hydrogen bonding, i.e., in the soft gel structure of the amorphous phase (6). A typical example of this mechanism is water-setting. The gel structure in hair is ubiquitous in all types of hair and plays a crucial role in most temporary shapes imposed by hair movement and daily grooming practices. It is part of the amorphous phase, and behaves like a material that exhibits variable viscosity depending on the shear rate it is exposed to (i.e., pseudoplastic with a yield stress). This type of behavior can turn hair from very soft and flexible to very hard and brittle and, in doing so, it can virtually lock-in almost any temporary shape in hair. The viscosity of the gel structure depends on the hair’s moisture content and, when hard and brittle, it presents a high opposition to the imposition or recovery of any type of shape in the hair. Temporary shapes in hair can also be stored by processes involving vitrification of the disulfide cross-linked amorphous phase and rearrangements of the crystalline phase. A typical example of this is the case of setting hair with hot irons.
The ideal creation of temporary shapes in hair shouldn’t involve permanent and irreversible modifications to the protein architecture of hair. This means that after creating a temporary shape, the hair’s permanent shape memory should remain intact, i.e., irreversible protein denaturation in crystalline filaments or irreversible breakage of covalent bonds in main protein chains and pendant groups should not be allowed to occur. If these irreversible phenomena occur, the hair may lose part or all of its permanent shape, becoming shapeless, and the imposition of a temporary shape will thus be irrelevant. As will be seen later, when hair is heated above its melting and glass transition temperatures with hot irons, temporary shapes can be created but at the expense of producing some of these unwanted irreversible phenomena.
d. The apparent permanent shape in hair
The hair shape that we normally perceive in our natural hair in everyday life is an apparent shape that results from a number of unavoidable mechanical effects. These unavoidable effects are primarily caused by hair’s own weight and by inter-fiber interactions in the hair assembly. Both effects produce a temporary shape that is stored in the gel structure of hair and is always present in our everyday life. It is a form of quasi-permanent water-setting. Therefore, the hair shape that we have in our natural hair is not the “real” hair’s native shape, but rather it is a very close representation of it.
We call this close representation an “apparent permanent shape” or temporary shape in equilibrium with the permanent shape. Other factors that contribute to the formation of the “apparent permanent shape” are hair length, relative humidity, and type of hair; i.e., it also depends on whether the hair is naturally straight, wavy, or very curly.
The “real” permanent shape of hair is only manifested when we immerse a single hair fiber in water so that the temporary shape imposed by its own weight and other interactions is released from the gel structure. For instance, experiments have shown that a high percentage of hair fibers from Caucasian origin that appear wavy in shape, regardless of their degree of waviness or curliness, turn into almost perfect circular coils when immersed in water (18)!
We probably have all observed these effects at one time or another upon seeing our own hair fibers floating in a pool of water. These effects are shown in Figure 5 where six single hair fibers from different origins are displayed as follows: (a) Oriental, (b), (c), (d) Caucasian, (e) Mulatto, and (f) of African origin. The shape of the Caucasian and Mulatto fibers was wavy/curly before equilibration in water. Observe in this Figure that the hair fibers of Caucasian, Mulatto, and African origin formed almost perfect circles after ten minutes of water immersion, except for the one of Asian origin. This means that the real permanent shape of most hair fibers that appear wavy in a hair assembly, is actually coiled and circular, and that the wavy pattern results from deformations mostly imposed by their own weight as the fibers hang from the scalp. Another important implication from these observations is that when hair emerges from the follicle, the shape of a high percentage of hair fibers of Caucasian origin is never wavy but rather circular with a predetermined radius of curvature. Thereafter, as the hair fiber grows longer it actually becomes wavy because it is deformed by both its own weight and the interaction with other hair fibers in the scalp.
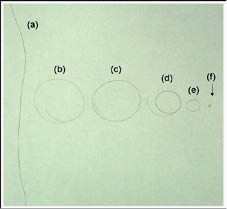
Figure 5) Picture showing six single hair fibers after their shape was equilibrated in water as follows: (a) Oriental, (b), (c), and (d) Caucasian, (e) Mulatto, and (f) of African origin (18).
Figure 6) Picture showing four single hair fibers before and after they were hung vertically as follows: (a) Oriental, (b) and (c) Caucasian, and (d) of African origin (12). The shape of hair fibers depicted in (a), (b), (c), and (d) captions had been equilibrated by water immersion for ten minutes (18).
The above-mentioned effects are better visualized in Figure 6 where it can be seen that the circular shapes of two equilibrated Caucasian hair fibers (b) and (c) turn into wavy patterns (f) and (g) as the hair fibers hang. In Figure 4 it can also be observed that the circular coil of an African hair fiber (d) presents a very small tight curly pattern, and does not open its coil upon hanging. The hair fiber of Oriental origin (e) did not change its straight shape very much after being hung.
The observations made on the behavior of African hair are not new and rather confirm what is already known by cosmetologists and beauticians; namely, that hair with a very tight coil is not readily opened by its own weight when hanging from the scalp regardless of its length. The tight curly hair will be opened, however, when we impose a force, either with the fingers or with other devices. As will be seen later in this chapter, hair with very tight curls, such as that depicted in Figure 6d, has a very small radius of curvature, and its modification requires processes that modify a larger number of memory sites in the hair.
The modifications introduced to real permanent shapes in hair by its own weight also explain well-recognized hair shape variations often observed in people’s hair when the environmental humidity changes. Higher levels of moisture result in hydrogen bond breakage in the gel structure and, therefore, moisture affects the “apparent permanent shape” of hair.
If the permanent shape is very strong, the hair will recover its real permanent shape, either curly or limp. For instance, people with wavy hair know very well that when the relative humidity is high their hair may become curlier. In contrast, people that have straight or fine straight hair as the real permanent shape may experience a higher level of hair limpness or straightening at high relative humidities. Furthermore, the formation of this “apparent permanent shape” also gives rise to differences in hair shape depending on its position on the head. For the sake of simplicity in the following paragraphs the term “real permanent shape” will be used when referring to hair shapes without any imposed deformation; and the term “permanent shape” will be used to refer to hair shapes in equilibrium.
e. Permanent shape memory in hair
The real permanent shape of hair is cast in the cortical cells during its life cycle through the anagen, catagen, and telogen phases (19–20). It is predetermined genetically and permanently imprinted in the hair follicle by the process of keratinization when the proteins in the amorphous phase are cross-linked by disulfide bonds. Figure 7 shows typical examples of hair with different types of permanent shape. Whether the hair is straight, wavy, or extremely curly, its permanent memory shape essentially resides in the composition ratio of ortho- to para-cortical cells. At the microscopic level, however, the permanent shape of hair is imprinted in the two-phase architectural structure of hair which results from the crystalline keratin micro-fibrils embedded in a highly cross-linked amorphous network of proteins (see Figure 8). More specifically, it is imprinted in the primary, secondary, and tertiary protein structures of crystalline and amorphous phases, chemically and physically stabilized by disulfide and isopeptide covalent bonds and by hydrogen bonds, salt bridges, and dispersion forces. Both protein phases stabilize hair shape and also strengthen the fibers mechanically.
Figure 7) Captions showing three types of natural permanent hair shape as follows: Oriental (left), Caucasian (center), and African (left).
Figure 8) Schematic representation showing two hair fibers with different shape. The permanent shape of hair, either curly or straight, is imprinted in the hundreds of micro-fibrils and amorphous protein networks forming the two-phase protein arrangement in the cortical cells (18).
f. Changes to the permanent shape in hair
Permanent changes in hair shape have very different requirements than those already outlined for temporary shape changes. While temporary changes do not require irreversible modifications to the hair structure, permanent changes do require this process. Irreversible modifications are needed, first, because it is necessary to erase or delete the permanent shape from the memory of hair, and second, because it is necessary to build a new permanent shape in the hair structure when faced with consumer demands and needs. The latter step usually requires irreversible modifications to the crystalline phase, the introduction of new chemical bonds, and the rearrangement of proteins in the amorphous phase. Thus, permanent changes in hair shape require irreversible modifications to one or two properties of sites where the permanent memory of hair shape resides.
The irreversible changes may be in the form of chain scission, chemical modification of protein-pendant groups, and breakage of disulfide bonds. They can also involve irreversible protein denaturation, irreversible melting of crystalline regions, or a combination of all these factors (21). Thioglycolate salt solutions and very high pH alkaline creams used in permanent waving and hair relaxers, respectively, involve exactly this type of irreversible protein denaturation. The former is selective to the breakage of disulfide bonds in the protein chains and does not have a strong impact on other chemical bonds. The latter also breaks disulfide bonds but is less specific to them and, it breaks other bonds in the protein chains as well. In addition, the phenomenon also produces irreversible modifications to the crystalline regions and to the whole hair architecture itself (22).
One factor often encountered in treatments geared to produce a permanent change in the shape of hair is that in many instances, they do not produce a real durable permanent change. As a result, there is a partial and gradual reversion to the original fiber shape. Lack of performance of active ingredients, poor formulation design, or poor hair treatment conditions are often the cause of this reversal. Consequently, in the case of permanent waving, various treatments have to be applied periodically to maintain the wavy shape (18). In the case of hair straightening with alkaline relaxers, shape reversion is difficult to correct because after the straightening process, the hair fibers are left weakened due to the high degree of broken covalent and other protein bonds produced by these treatments.
Shape reversion phenomena occurring after permanent waving or alkaline relaxer treatments are usually difficult to explain when using conventional mechanisms of disulfide bond breakage and re-formation. However, the shape reversion processes can be easily explained when it is considered that hair is a shape memory biopolymer with a permanent shape stored in its “memory.” As will be discussed later in this chapter, in order to successfully induce permanent changes in hair shape, not only do disulfide bonds have to be broken, but also other chemical and key structural components of the hair memory need to be modified. Otherwise, the induced shape will be semi-permanent and eventually the fibers will undergo shape reversion.
Understanding the shape memory properties of hair can pave the way to optimize processes and produce successful permanent changes in hair shape. Therefore, in the following paragraphs shape memory polymer concepts will be used to review changes in hair shape, and we encourage the reader to use this information as a foundation for innovative thinking in this field.
3.3.4.3 CHANGES IN HAIR SHAPE BY WATER-SETTING
Water-setting, or the process of changing hair’s shape with water, is by far, among all the temporary setting processes, the easiest to produce in hair. It only entails the use of water and a relatively low-temperature drying process. In order to understand it mechanistically using concepts from the science of SMPs, let us first review the key generic steps necessary to produce shape changes in semi-crystalline polymers (6), and then apply them to hair. Upon thoughtful comparison, polymers also require the same three key steps described above for changes in hair shape:
- 1) making fluid or softer at least one of the polymer phases,
- 2) imposing mechanically a new shape followed by stress relaxation in the soft/fluid phase, and
- 3) immobilizing the soft/fluid phase to lock-in the new shape. In general, temporary shape changes in SMPs also require keeping the polymer structure intact where the permanent shape memory is located (11).
Thus, when we want to impose a temporary shape on a semi-crystalline polymer, or by analogy, in hair, the first requirement is to keep the main polymer architecture intact. To be more specific, in the case of water-setting this implies that the disulfide bond cross-linked protein matrix and the protein crystalline regions should not be modified. Water-setting usually does not produce these modifications except when high temperatures, or large deformations are involved in the production of the temporary shape. High temperatures above 80°C may already start to produce disulfide bond breakage; while deformations higher than 5% already induce alpha to beta transitions in the crystalline phase which may be partially irreversible (23).
b. Physical processes taking place inside hair during water-setting
As outlined in the previous paragraph the imposition of temporary shapes, either in polymers or in hair, requires three main steps (see Figure 9). For the water-setting of hair, step 1 consists of softening the gel structure of the amorphous phase by wetting the hair and allowing water to penetrate into the cortex. This first step will ensure that hydrogen bonds in the gel structure are broken by water. It is important to mention here that when water breaks hydrogen bonds in hair proteins, after step 1 the hair still has all of its covalent bonds intact, and therefore, the permanent shape memory is also unchanged (see Figure 10). As will be seen later, this contrasts with other processes where the hair becomes shapeless and completely loses its real permanent shape. In step 2, once the weak hydrogen bonds are broken, the hair is set into a new configuration with plastic rollers or with the fingers to create the desired new shape, which can be either that of light hair waves or tighter curls.
Figure 9) Schematic representation of three steps required to create a temporary shape by water-setting. The three-step process is essentially based on the breakage of hydrogen bonds in the gel structure of hair (step 1), imposition of temporary shape (step 2), and re-formation of hydrogen bonds to lock-in the new shape (step 3) (18).
Figure 10) Schematic representation of hydrogen bond breakage by water dipoles during water-setting. Other covalent bonds are not affected.
Finally, in step 3, the hair has to be rapidly dried to extract water molecules in order to re-form the hydrogen bonds in the gel structure. This process will cause hardening of the hair, thereby locking-in the temporary shape and imposing it over the primary hair shape. Drying can be achieved by just exposing hair to atmospheric conditions or it can be accelerated with the aid of blow-dryers. If the drying process is too slow and the hair rollers are removed, the crystalline micro-fibrils and amorphous matrix with its combined elastic force will push for hair to recover its permanent shape and the temporary shape will be lost. Fast drying with blow-dryers will ensure that water is rapidly removed from the gel phase before the hair can elastically recover its permanent shape. In this manner the new shape will be locked-in.
c. Temporary shapes induced by long-term deformations
Another form of water-setting is the one that takes place when hair is subjected to involuntary deformations for extended periods of time at room temperature and high moisture conditions. This situation occurs, for instance, when sleeping, using hats, etc. In these cases the assembly of hair fibers is held in a configuration for various hours, and after freeing the hair from the confined environment a temporary change in hair shape is produced. The phenomenon is caused by stress relaxation in the gel structure of the amorphous phase.
As was previously discussed, the gel phase of the hair is plasticized when the amorphous phase contains moisture. Water from the environment is absorbed in the gel structure and breaks a limited number of hydrogen bonds at protein neighboring sites. When there are no mechanical stresses applied to hair, the broken and unbroken bonds stay in equilibrium. However, when deformations appear as a consequence of imposed deformations, i.e., during sleep, use of hats, etc., broken and unbroken bonds undergo a bond interchange process and the protein chains move to relax internal stresses. This process of internal stress relaxation in the gel structure locks-in the temporary shape that is produced during sleep (bed hair), or with the use of hats, etc. (see Figure 11).
Figure 11) Caption showing typical appearance of bed hair (18)
The imposition of new temporary shapes on hair, either during sleep, use of hats, etc., or during water-setting, will immediately produce internal elastic stresses in the structures where the real permanent shape resides. This occurs primarily in the crystalline regions and in the disulfide protein matrix. These internal stresses will remain there as long as the temporary shape is in place and are needed for the hair to recover its permanent shape. Temporary shapes imposed by water-setting will maintain their secondary character as long as the two following conditions are met. First, the elasticity of the crystalline and amorphous phases does not change, and second, that the gel structure of the amorphous phase remains unchanged. The first condition was previously reviewed and it was referred to as a requirement for superelasticity of the SMPs. This condition is required to ensure that the polymer will always remember its permanent shape. The second condition was also reviewed previously and it relates to the absence of relaxation in the soft phase; it is required to lock-in the temporary shape as long as it is necessary until it is released by an external stimulus.
Shape changes induced by water-setting can, rapidly or almost instantaneously, be reversed by water-wetting. The process of liquid water absorption by hair causes rapid hydrogen bond breakage, softening, and swelling of the gel structure, thereby releasing the temporary shape and allowing the permanent shape to be quickly recovered. If the hair didn’t behave like a shape memory biopolymer, it would not remember and recover its permanent shape and would stay deformed like a viscous resin. Thus, water-wetting acts as an external stimulus to quickly release the temporary shape from hair.
d. Limitations of water-setting
Unfortunately, shape changes induced by water-setting in hair are temporary and cannot be maintained as long as we would like nor can they be dormant forever. It is known, for instance, that shape changes induced by water are slowly recovered even when no water-wetting processes are applied. Temporary shapes stored in the gel structure are gradually lost because, as has previously been explained by Max Feughelman (6), the gel structure of phase M in hair is viscoelastic, and even at very low moisture contents it will always relax to the Elastic Modulus of the crystalline phase. Thus, all types of temporary shapes induced by water-setting eventually revert back to the permanent shape by a stress-relaxation process and this occurs even under very dry conditions. The process may take months but the shapes will reverse! The memory shape properties of the gel structure in hair are transient, and temporary shapes induced by water-setting cannot be maintained for very long periods.
Another important aspect of water-setting is that, depending on the type of hair, there is a limit on the type of initial shape that can be imposed. This fact becomes more clearly understood when we analyze the following questions:
- ● How tight can we induce a curl in hair that is very straight, or how straight can we render hair that is very kinky?
- ● Can this induced curl be achieved just by using a water-setting process? In other words, is it possible to put very tight curls in natural straight hair just by breaking and re-forming hydrogen bonds? Or is it possible to render very kinky hair straight just by breaking and re-forming hydrogen bonds?
Obviously, the answer to the above is no. Everyone who has tried to straighten extremely curly hair just by using water-setting will fail.
This failure can be explained by the fact that the gel structure has only limited mechanical stiffness, and therefore, cannot completely counter the strong elastic recovery forces of permanent shape coming from the crystalline and disulfide cross-linked protein phases. In other words, the gel structure is a weak physical memory and cannot store large changes in hair shape. Thus, the strength and degree of hair curliness is very important in temporary shape changes induced by water-setting. The straightening of extremely curly hair may require additional physical modifications of other, stronger protein structures that store temporary shape. Likewise, this type of reasoning can be applied for the imposition of tight curls in straight hair with water-setting.
According to the ongoing discussion, there appears to be a rule that relates strength of shape change, and number of memory sites required to achieve the changes in shape. This rule always prevails when imposing temporary changes in hair shape. In the case of straightening curly hair, the rule is: the tighter the curl, the more the elements in the architectural sites in hair’s memory need to be modified to make it straight. This means that for any particular type of hair, there is a limit in the degree of shape changes that can be imposed by water-setting. In fact, this is the reason for the use of harsher temporary hair-shaping techniques, other than water-setting, for the straightening of curly hair, such as the use of hot irons and other treatments. As will be seen later in the following paragraphs, the rule of changing shape has important consequences not only for the imposition of temporary shapes but also for engendering those of permanent nature.
3.3.4.4 CHANGES IN HAIR SHAPE BY HOT IRON TREATMENTS
Changing the hair shape with hot irons is a very old practice. Museums of history already report drafts of hot iron designs made in the 18th century (see Figure 12). Hot irons became popular in the early 1900s for the straightening of extremely curly hair of African origin (24). Later, over the years the application gradually extended to other types of hair to create/enhance stronger curls or to create straight patterns in hair. Currently, there are two main types of hot irons, namely, those of cylindrical shape and those with flat plates (see Figure 13). The use of hot irons with flat plates has become very popular for the straightening of wavy hair. The wanted effects from those who use it nowadays range, in terms of achieving long-lasting behavior—from totally irreversible, or permanent types, to those of long duration and reversible behavior. Most hot iron treatments used today leave only a short temporary change in hair shape. As a result, cosmetic formulators are perennially looking for new hot iron technologies that can extend the persistence and long-lastingness of heat treatments.
Figure 12) Caption displaying draft describing hot irons used in 1858 (24)
Most of the progress made in the treatment of hair with hot irons has produced innovations in their design and in the use of new ingredients or formulations to aid in the hot ironing process. Innovations in hot iron design comprise, to mention a few: more efficient temperature control, new ceramic materials to improve surface smoothness, better and effective hot iron/hair contact area, use of steam, and the incorporation of infrared heating elements (25). To this end, progress in the area of ingredients and formulations has led to the development of two types of products; namely,
- 1) those based on the use of polymers geared to enhance shape effects and also to reduce hair damage (26), and
- 2) those using ingredients that modify or introduce new bonds in hair.
The latter type of products in most cases requires the incorporation of heat to modify the permanent shape memory of hair; either by introducing new cross-links, or by changing the disulfide cross-linking structure of the proteins. Examples of these are the formaldehyde (Brazilean) and sodium bisulfite (Japanese) treatments; both will be reviewed later in another paragraph.
Figure 13) Main modern types of hot irons, flat hot iron (top), and rod hot iron (bottom)
A typical hair-straightening process with hot irons consists of the following steps: 1) pre-wash and condition hair with the shampoo and conditioner of choice, 2) dry hair until it keeps about 80% of its moisture content, 2) divide hair into thin swatch sections and then apply the hot iron. The temperature of the hot iron, depending on the type of desired effects, is usually selected between 160 and 230°C. The hot iron is passed two or three times over the hair swatches from root to tip at a speed of approximately one inch/sec. During application the hot iron is held firmly but gently with the hand, so the resulting force of friction will allow smooth sliding over the hair surface, and also, will enhance heat transfer at the interface of hot iron/hair. Excessive force application with the hand should be avoided since this will unnecessarily increase friction at the hot iron/surface interface causing the hot iron to slow down or completely to stop. If this happens the hot iron will damage the hair swatch. If properly used, flat hot irons produce immediate straightening effects in hair (see Figure 14).
Figure 14) Changes in hair shape before (a) and after (b) treatment with a flat hot iron. The hair shape has been changed from permanent semi-curly to temporary straight.
Good contact between the hot iron and hair is always necessary for efficient heat transfer. It is important to recognize that heat transfer from the hot iron plates to the hair swatch is always poor because hair, like wool, is a poor heat conductor. Furthermore, because of hair irregularities at the cuticle sheath surface and in view of the formation of air pockets located between hair fibers in the swatch, there is always poor thermal contact between them. This condition causes uneven temperature gradients between hot iron surface and hair swatch. Measurements carried out by placing a thermocouple inside a hair swatch have shown that hair fibers placed in a row 100 micrometers below the hot iron surface always sense at all times an average of 20 to 25°C less than those at the row surface contacting the flat iron plate (27).
c. Mechanical action of hot irons
The mechanical action of hot irons on hair is different depending on whether we try to impose a curl on straight hair or whether we try to straighten curly or wavy hair. For instance, if we place a swatch of straight hair in a hot iron of cylindrical shape at 210°C, and press on the hair statically without motion, the hair will acquire a curled shape. In contrast, if we place a swatch of wavy or curled hair between the plates of a flat hot iron at 210°C and let it be there statically without motion, it will not become straight; the action of flattening alone will not lead to changes in hair shape from wavy/curly to straight. For hair to become straight it needs to be in motion so its length can be stretched at least until the curl opens and the hair becomes straight. Thus, there is a big difference in the way the hot iron acts mechanically. This difference depends on whether the change in shape is from straight to curly or from curly to straight. Very curly hair needs a relatively high friction imposed at the interface between the hot iron and the hair surface so the iron plates can stretch and open the curl. In contrast, the process of changing straight hair to curly is not dependent on whether the hair is in motion or not.
Other factors that influence the friction process during the straightening of wavy hair with flat irons are the type of products applied to hair before hot ironing. Hair swatches to be ironed may contain polymers, surfactants, and oils on the surface prior to the ironing process. These ingredients, in particular, include polymers that have been placed there to aid in the styling process. The polymers appear to have an effect in preventing hair damage by eliminating uneven harsh temperature gradients that may create hot spots on the hair swatch (28). Polymers and oils can have, however, a negative effect on the hot ironing process as they can excessively increase or lower the interfacial friction between the iron and the hair surface (34). High levels of friction may cause the hot iron to stop or to produce lifting of cuticle cells and tear them away from the hair (see Figures 15 and 16), while very low friction may reduce the uncoiling of the curl compromising the efficiency of a hair-straightening process (27).
Fig 15) Micrograph of a single hair fiber showing cuticle cell deformation and lifting after treatment with a hot iron at 210°C. The colored sections represent lifted cuticle cells showing patterns of light interference (27).
Figure 16) Micrograph showing fragments on cuticle cells left on plate of hot iron after a hair bundle was treated with a hot iron. Cuticle cell removal was produced by excessive friction at hot iron/hair interface (27).
3.3.4.5 PHYSICAL PROCESSES TAKING PLACE
INSIDE HAIR DURING HOT IRONING
Anybody who has witnessed for the first time the application of hot irons to hair is struck by the hissing sound of water vapor or steam coming out of the hair as the hot iron is passed over the hair swatches. At the microscopic level this means that a large quantity of “free” and “bound” water molecules are being rapidly extracted from the protein sites in the amorphous phase. Water-absorbing groups such as amino, carboxyl, and other functional groups capable of forming hydrogen bonds lose their water in this process. As a result, the gel structure of the amorphous protein phase changes from a soft and highly plasticized gel to a hardened amorphous mass.
Experimental observations indicate that rapid water evaporation from hair starts to take place when the hot iron reaches temperatures slightly higher than 100°C. Moreover, if the temperature of the hot iron is maintained at 120°C for 15 seconds while it is in contact with the hair swatch, the hair will be fully dehydrated. This is not surprising since bulk water starts to evaporate rapidly at 100°C. Yet, observations made by scientists in the laboratory and by many stylists confirm that when the temperature of the hot iron is set between 100 and 120°C, it is difficult or almost impossible to efficiently impose a temporary shape on hair, either straight or curly (27).
Furthermore, work carried out in this area indicates that the shape of a wavy hair swatch does not change efficiently from wavy to straight even when the temperature of the hot iron is increased to 150°C. These experiments also indicate that it is not until the hot iron reaches temperatures between 150 and 230°C, that a noticeable and efficient change in shape from wavy to straight starts to appear in hair (27). These observations indicate that the physicochemical processes that are responsible for inducing changes in the shape of hair occur at temperatures ranging between 165 and 230°C, and when the hair is almost fully dehydrated. Incidentally, the only protein processes that take place inside hair within this temperature range are the melting of crystalline proteins and the softening of the amorphous matrix. The relation of these two processes with shape changes in hair will be discussed below.
b. Phase changes and transitions in hair
The thermodynamic transitions taking place in hair when its temperature is increased from 25 to 240°C have been the subject of numerous studies (29–31). Most hair types have been shown to undergo two main thermodynamic transitions, namely a first-order transition associated to the denaturation and melting of its crystalline structure, and a second-order transition associated to the softening or glass transition of its amorphous protein structure. The melting of the crystalline structure in virgin hair occurs at Tm ~ 156°C, and the softening of the amorphous disulfide cross-linked protein phase occurs at its glass transition temperature, Tg ~ 144°C.
The occurrence of these two transitions in most types of hair can be detected and confirmed by Differential Scanning Calorimetry. For instance, in Figure 17 can be seen a typical DSC thermogram showing the endothermic peak related to the melting of the hair crystalline phase. Thus, since the working temperature of a typical hot iron treatment ranges between 150 and 230°C, it is straightforward that both of these transitions take place in the hot iron straightening process. In fact, as will be seen later, the better efficiency in changing hair shape temporarily with hot irons is due precisely to the occurrence of these transitions, as they are the sites with temporary memory properties.
Figure 17) Caption of a typical thermogram obtained by DSC from hair (32).
c. The mechanisms of water and hot iron setting are different.
With the material presented thus far, it is not difficult to anticipate that the mechanism by which hot irons impose a temporary shape in hair is very different from that occurring in water-setting. Setting hair with hot irons does not involve the key and crucial typical step of water-setting, namely that consisting of the breakage of a high quantity of hydrogen bonds to soften the gel structure followed by imposition of the new hair shape.
Setting hair with hot irons involves the formation of hydrogen bonds, but they do not contribute to imposing a new shape. In order to better understand the role of hydrogen bonding during hot iron treatments, let us start by analyzing the role of water on the properties of the gel structure in hair. When water molecules penetrate into the hair structure they adsorb and intercalate on protein-functional sites breaking hydrogen bonds between them. The breakage of these hydrogen bonds renders the gel structure of the amorphous phase softer (6). When the water molecules are removed by evaporation, the internal hydrogen bonds between neighboring protein chains re-form and transform the soft gel structure into a hard viscous gel mass.
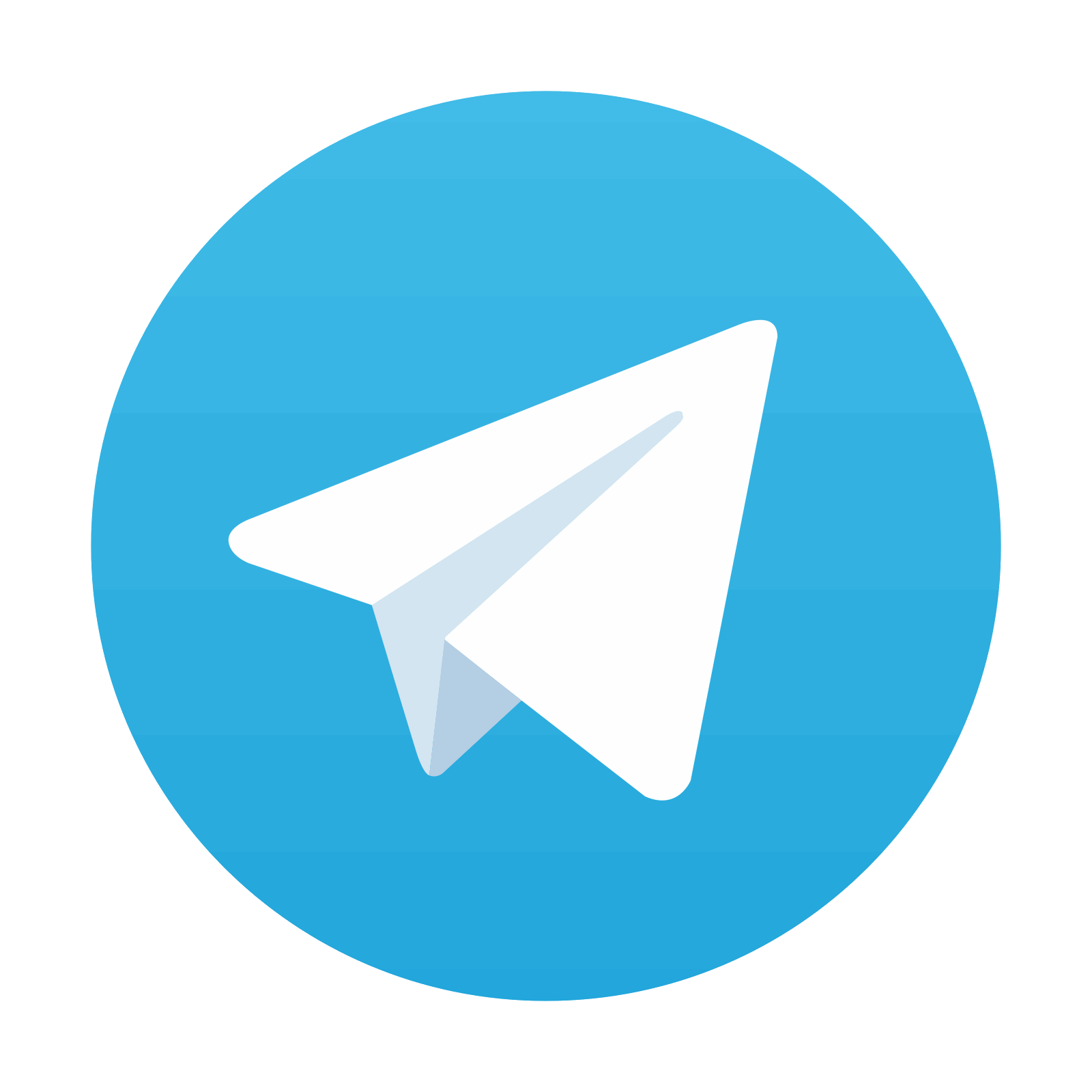
Stay updated, free articles. Join our Telegram channel

Full access? Get Clinical Tree
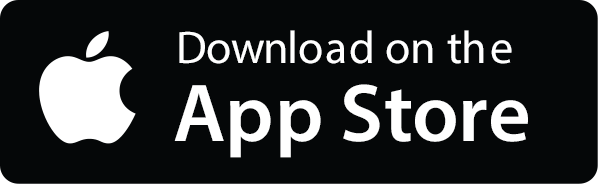
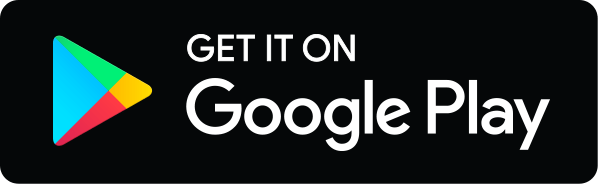