Markers
MNC
BM-MSC
CD45
+
−
CD31
+
−
CD34
+
−
CD105
+
+
CD14
+
−
CD44
+
+
CD29
+
+
HLA-DR
+
−
HLA-ABC
ND
+
CD106
_
−
CD146
+
+
CD90
+
+
CD13
+
+
CD133
+
−
CD73
+
+
CD49d
+
+
CD166
+
+
CD54
+
+
ABCG2
−
+
ALDH
−
+
CD117
+
+
CD11a
ND
−
CD11b
ND
−
SOX2
+
+
Nanog
+
+
Oct-4
+
+
SSEA-4
+
−
CD83
+
−
CD86
+
−
CD209
ND
−
140b
ND
+
CD56
+
−
4.7.3 Subcutaneous Fat-Derived Stem Cells
New insights on WAT biology emerged when a number of studies reported the existence of stem cell population isolated from human subcutaneous adipose tissue, showing characteristics similar to the mesenchymal stem cells isolated from bone marrow [150–152]. These subcutaneous adipose tissue-derived stem cells were found to possess extensive proliferative capacity and the ability to differentiate into multiple cell lineages [5, 25, 92, 153–155]. The relative ease and frequency of clonogenic cells, accessibility, abundance, and replenishing ability were some reasons for interest in subcutaneous adipose tissue as a stem cell source. Until the identification of stem cells from omentum fat, the term adipose-derived stem cell (ASC) was the terminology commonly used to refer the stem cells of subcutaneous fat alone. Attention in considering subcutaneous adipose tissue as a reservoir of stem cells was really undertaken only after the findings of Zuk and his co-workers, in the year 2001 [4]. The translation of his findings associated with the easy sampling of adipose tissue with its low risk and morbidity attracted many new investigators. Subsequently, increasing evidence is accumulating on the pivotal role of subcutaneous fat-derived stem cells owing to their proliferative capacity and multilineage differentiation ability [25, 92, 149, 152–154]. In a wide perspective, a range of names has been used to describe the adherent cell population isolated from adipose tissue, e.g., lipoblasts, preadipocytes, processed lipoaspirate (PLA) cells, adipose-derived stem/stromal cells (ASCs), adipose-derived adult stem (ADAS) cells, adipose-derived adult stromal cells, human multipotent adipose-derived stem cells (hMADS), and adipose mesenchymal stem cells (AdMSCs) [155, 156]. To address the problem, a consensus reached by investigators at the 2004 conference of the International Fat Applied Technology Society (IFATS) in Pittsburgh has proposed a standard nomenclature by adopting the term ASCs to identify the isolated, plastic-adherent, multipotent cell population [158].
4.7.4 Surface Antigenic Profiles of Subcutaneous Fat-Derived Stem Cells
Despite nearly a decade of research efforts, the phenotypic and functional characteristics of ASCs remain obscure. Although fibroblastic morphology of cells is one of the characteristics to identify MSC, such characterization alone is insufficient to confirm the isolation of a true population. Thus, immunophenotypic characterization of cell surface markers becomes an ideal characteristic feature of stem cells. Many attempts have been made to develop a cell surface antigen profile, in order to better purify and identify ASCs. Over the past 6 years, many papers have reported on characterization of cell surface markers at stromal vascular fraction or at different stages of ASC culture. Considerable progress has been made towards phenotypic characterization of ASC; however, the expression profile changes as a function of time in passage and plastic adherence [159, 160]. Besides, some cell surface markers have been detected with highly consistent patterns of expression (CD13, CD29, CD73, CD90, CD105, CD166, CD133, CD45, CD31, MHC I, and MHC II) on the surface of ASCs by different literatures [2, 25, 150, 153, 161–163]. Still considerable heterogeneity in the full range of ASC surface markers had been reported. Some of these variations are found in the cell population within a single culture, indicating either the presence of mixed population of cells or the modulation of cell surface proteins during cell culture. Furthermore, the comparative analysis of phenotypic expressions had been confounded by the differences in thresholds used to report positivity in staining by different research groups [12, 157, 164–166].
Despite the in vitro identification of ASCs made possible through the markers put forth by ISCT, their role with respect to other cell populations, such as side population, endothelial progenitor population, and specifically cell adhesion molecules within extracellular matrix, is not clear, and there is a lack of consensus in the results of other MSC-specific markers as well. Although ASC shows very similar expression patterns as that of BMSC, it still remains unclear in the aspects of ASC-specific marker [25, 150, 153, 163]. On the contrary, there are also reports specifying the variations exhibited among certain markers of ASC when compared to BMSC and vice versa [2, 12, 150, 165], further making cell surface marker expression study on ASC an arduous task. For example, it was identified that ASCs express CD49d and not CD106, whereas bone marrow MSCs express CD106 but not CD49d. This reciprocal expression pattern is interesting because CD106 is the cognate receptor of CD49d, and both of these molecules represent part of a receptor–ligand pair that has an important role in hematopoietic stem cell homing to, and mobilization from, the bone marrow [166–169]. Despite the lack of CD106 in ASC, CD49d along with the higher expression of its counterpart, CD29, together forming VLA-4, is supposed to play a role in mobilization and homing [25, 137, 150, 157, 166, 170, 171]. In addition, it is predicted that high expression of CD44 is required for firm adhesion of MSC to the endothelium. Data shows that CD44 is activated by PDGF [92] that plays an important role in exogenous migration to the injured site by interaction with hyaluronate. Besides, CD13 was also identified to be a potent marker that plays a vital role in angiogenesis and migration [137]. This wealth of knowledge on these markers about their crucial migration and homing evokes that these markers impersonate a CAM that performs these aforesaid functions in ASC. Although the existence and functionality of certain ASC-specific markers are known, there is uncertainty among the specificity and functionality of several other markers of ASC.
Overall, the ASCs were identified by expressions of various markers such as Stro-1, CD9, CD10, CD13, CD29, CD44, CD90, CD49a, CD49b, CD49d, CD49e, CD49f, CD54, CD55, CD59, CD166, CD71, CD117, CD19, CD146, and HLA-ABC. Similarly, there is evidence supporting that the surface markers CD31, CD45, HLADR, CD11b, CD14, and CD34 were decreased in expression or were lost with passage, suggesting that adherence to plastic and subsequent expansion will select for a relatively homogenous cell population compared with the SVF [2, 4, 155, 156, 162, 166]. One of the key issues yet to be resolved is the absence of comprehensive information on certain specific markers of ASC, especially the cell adhesion molecule that interacts with the cytoskeleton of MSC. This might enhance the understanding of ASC as an instrument of curative therapeutics involved in the applications of neovascularizations, angiogenesis, and treatment of other vascular disorders. Furthermore, these definitive cell surface markers of ASC would help not only to distinguish them from other cell population in cell culture but also to enable purification of ASC from uncultured SVF. The description on the existence of heterogenous and homogenous cell surface markers that can reliably specify ASC as depicted (Table 4.2) facilitates researchers to explore further therapeutic potentials of stem cells with SVF as well as ASC. Besides, as mentioned earlier, the discrepancy on the change in surface marker expression in culture condition is also an important issue to be addressed. The diagrammatic representation of the flow cytometric characterization of cell surface markers of various cell lineages in different media under long-term culturing was analyzed by our research group and the data has been illustrated (Fig. 4.1) [172].
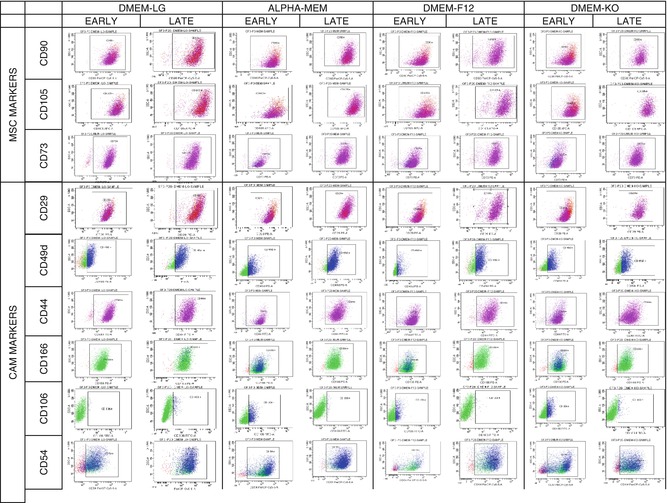
Table 4.2
Cell surface marker expression profile of freshly isolated SVF versus cultured SF-MSC
Markers |
SVF |
SF-MSC |
---|---|---|
CD45 |
ND |
− |
CD31 |
+ |
− |
CD34 |
+ |
− |
CD105 |
+ |
+ |
CD14 |
ND |
− |
CD44 |
+ |
+ |
CD29 |
+ |
+ |
HLA-DR |
− |
− |
HLA-ABC |
ND |
+ |
CD106 |
+ |
− |
CD146 |
+ |
+ |
CD90 |
+ |
+ |
CD13 |
+ |
+ |
CD133 |
+ |
− |
CD73 |
+ |
+ |
CD49d |
+ |
+ |
CD166 |
+ |
+ |
CD54 |
+ |
+ |
ABCG2 |
+ |
+ |
ALDH |
+ |
+ |
CD117 |
− |
+ |
CD11a |
ND |
− |
CD11b |
ND |
− |
SOX2 |
ND |
+ |
Nanog |
ND |
+ |
Oct-4 |
ND |
+ |
SSEA-4 |
ND |
− |
CD83 |
ND |
+ |
CD86 |
ND |
+ |
CD209 |
ND |
ND |
140b |
ND |
+ |
CD56 |
ND |
− |
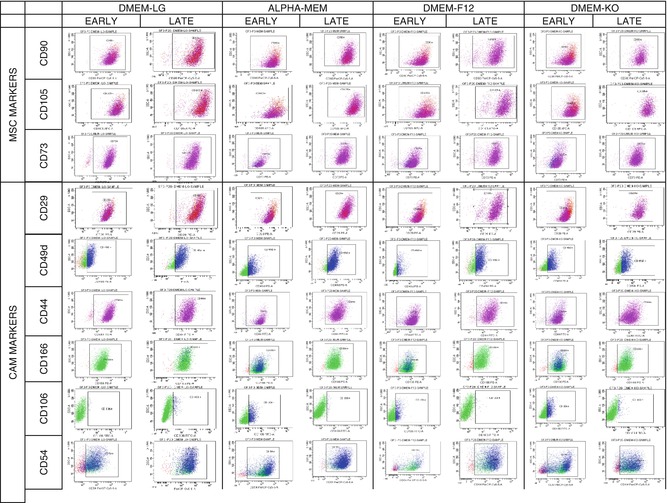
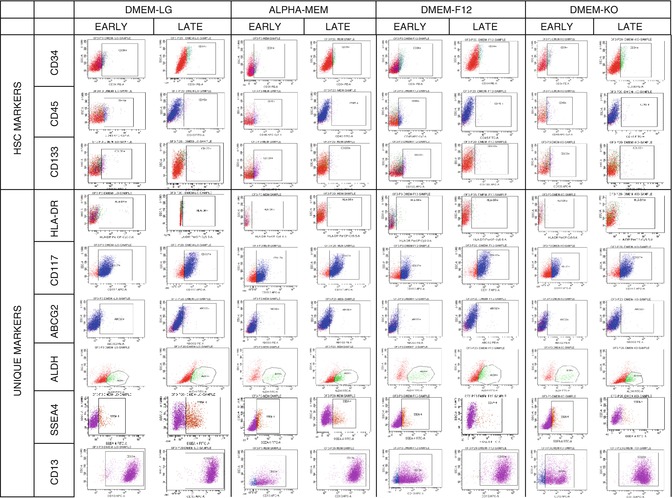
Fig. 4.1
Flow cytometric characterization MSC and cell adhesion molecules of ADSC at different media under long-term culturing
4.7.5 Omentum Fat-Derived Stem Cells
Similar to the existence of subcutaneous fat-derived stem cells, there are evidences that visceral fat does possess putative stem cell population [15, 173]. Data reported that identification of putative stem cell population in omental adipose tissue could represent a very useful tool to investigate, at the cellular level, the molecular mechanism and process involved in the onset of obesity and related metabolic dysfunctions. It is due to the fact that omentum fat is more closely related to the cardiovascular and metabolic complications rather than subcutaneous fat tissue, suggesting the existence of functional difference associated with the regional distribution of fat depots. This is further supported by both animal and human studies [15, 173], demonstrating that subcutaneous adipose tissue liposuction influences BMI and overall weight, whereas only reduction of visceral fat can also substantially ameliorate metabolic parameters [174]. Thus, existing evidence supports the reduction of omental fat tissue to be beneficial, and hence, omentum fat can be used as an ideal source for further stem-cell-oriented clinical applications. Apart from this, existence of mesenchymal stem cells in omentum fat has also emerged as a source of stem cell therapeutics for treating a wide horizon of diseases. However, there have been very scanty citations with human omentum fat-derived stem cells.
4.7.6 Surface Antigenic Profiles of Omentum Fat-Derived Stem Cells
While an extensive body of research exists pertaining to phenotypic characterization of stem cells from the bone marrow and subcutaneous adipose tissue, the phenotypic characterization of omentum fat-derived stem cells is in its infancy. Existing evidence on immunophenotypic characterization of omentum fat at early passage reveals the similarities in the expression of cell surface markers of omentum fat in comparison to subcutaneous fat and bone marrow [175, 176]. Omentum fat was found to express a wide range of markers. The heterogenous stromal vascular fraction isolated from omentum fat was found to express cell surface markers specific for hematopoietic stem cells, mesenchymal stem cells, cell adhesion molecules, endothelial cells, and other non-stem cell populations as well.
Although omentum fat is a potent source of stem cells with certain attributes, there are no unique accepted biomarkers that have been reliably identified yet. Identification of these unique markers would bring the research on omentum fat-derived stem cells to the forefront of regenerative medicine. In addition, it also leads to the identification of the cellular and molecular mechanisms of disease states. From the expression profile of the markers identified so far in current studies, it was shown that, under culture condition, the omentum fat expresses the markers (CD90, CD105, and CD 73) as reported by ISCT [46] and also expresses various cell adhesion molecules such as CD29, CD44, CD31, CD106, CD54, CD49d, and CD166. These studies suggest migratory and regenerative potentialities of omentum fat-derived MSC. In addition, cell surface markers such as CD34, CD31, CD45, CD133, and HLA II were found to be decreasing/negative in their expression [15, 16, 177, 178] of cultured ASC but seemed positive in uncultured heterogenous fraction [15, 16, 137]. Apart from the expression of the routine cell surface markers, omentum fat also expresses markers similar to embryonic stem cells: Oct-4, SOX2, Nanog, and so on. This may not only explain the multipotent nature but also suggest a pluripotent property of omentum fat that might enhance its applicability. The tissue-specific cell surface markers in relation to the isolated SVF versus the cultured ASCs of omentum fat are illustrated (Table 4.3).
Table 4.3
Cell surface marker expression profile of freshly isolated SVF versus cultured OF-MSC
Markers |
SVF |
OF-MSC |
---|---|---|
CD345 |
+ |
− |
CD31 |
+ |
− |
CD34 |
+ |
+ |
CD105 |
+ |
+ |
CD14 |
+ |
− |
CD44 |
+ |
+ |
CD29 |
+ |
+ |
HLA-DR |
+ |
+ |
HLA-ABC |
+ |
− |
CD106 |
+ |
− |
CD146 |
ND |
− |
CD90 |
+ |
+ |
CD13 |
ND |
+ |
CD133 |
+ |
− |
CD73 |
+ |
+ |
CD49d |
− |
+ |
CD166 |
− |
+ |
CD54 |
+ |
+ |
ABCG2 |
− |
+ |
ALDH |
+ |
+ |
CD117 |
+ |
+ |
CD11a |
ND |
− |
CD11b |
ND |
− |
SOX2 |
ND |
+ |
Nanog |
ND |
+ |
Oct-4 |
ND |
+ |
SSEA-4 |
ND |
− |
CD83 |
ND |
+ |
CD86 |
ND |
+ |
CD209 |
ND |
+ |
140b |
+ |
+ |
CD56 |
ND |
− |
The biomarkers mentioned above were in regard to the early passage conditions. However, retention of marker positivity for longer culture periods is of utmost importance to confirm the unique markers specific to MSC derived from omentum fat. So far there are no identities of prospective biomarkers available for extensive culturing of omentum fat-derived ASCs as they are novel and emerging sources of stem cells. Thus, our research group focuses on omentum fat-derived ASC optimization, and the prospective biomarker identification study carried out in long-term cultures showed similar expressions of all the markers tested with slight variations [178]. Two attributes clearly became evident regarding the withholding capacity of the cell surface marker expression through extensive culturing. Firstly, omentum fat does not lose its phenotypic characteristics in prolonged culture condition and maintains the similar phenotypic expression throughout. Secondly, it is evident that the stemness nature is not affected, and there is no change in expression profile due to the difference in media composition including higher glucose concentration under extensive culturing. Hence, it can be concluded that omentum fat can be regarded as an efficient alternative source of curative therapeutics similar to that of subcutaneous fat and bone marrow with regard to the cell surface antigenic expression profile. However, being the novel emerging source, it demands several independent research works on identification of tissue-specific cell surface markers that can be reliable and further explored as a potential therapeutic target as well for regenerative medical applications.
4.8 Conclusions
Currently, expanding human MSCs in culture is complicated by one of the facts that characteristic surface markers used to identify the cell populations continue to change. Accumulating evidences report that this change in marker expression profile of mesenchymal stem cells is either due to the long-term in vitro culture condition or due to the different media/serum composition. Thus, the search for tissue-specific surface antigens representing the pure, native MSC population remains one of the most challenging topics of MSC research. This chapter provides a deep insight into some of the lineage-specific markers and emphasizes immunophenotyping as a tool to identify existing tissue-specific markers, as well pave way to identify further novel biomarkers. This comprehensive informatics on surface antigenic profile characteristics offers a new avenue of investigation towards molecular understanding of stem cell self-renewal and differentiation when integrated into existing stem cell research strategies. In addition, this serves to resolve the existing enigma on the prospective biomarker identification. However, much is needed to be explored to investigate its far wider potentials.
References
1.
Friedenstein AJ. Stromal-hematopoietic interrelationships: Maximov’s ideas and modern models. Haematol Blood Transfus. 1989;32:159–67.PubMed
2.
Gronthos S, Franklin DM, Leddy HA, Robey PG, Storms RW, Gimble JM. Surface protein characterization of human adipose tissue-derived stromal cells. J Cell Physiol. 2001;189(1):54–63.PubMed
3.
Pittenger MF, Mackay AM, Beck SC, Jaiswal RK, Douglas R, Mosca JD, Moorman MA, Simonetti DW, Craig S, Marshak DR. Multilineage potential of adult human mesenchymal stem cells. Science. 1999;284(5411):143–7.PubMed
4.
Zuk PA, Zhu M, Mizuno H, Huang J, Futrell JW, Katz AJ, Benhaim P, Lorenz HP, Hedrick MH. Multilineage cells from human adipose tissue: implications for cell-based therapies. Tissue Eng. 2001;7(2):211–28.PubMed
5.
Gimble JM, Katz AJ, Bunnell BA. Adipose-derived stem cells for regenerative medicine. Circ Res. 2007;100(9):1249–60.PubMed
6.
Dhanasekaran M, Indumathi S, Rajkumar JS, Sudarsanam D. Effect of high glucose on extensive culturing of mesenchymal stem cells derived from subcutaneous fat, omentum fat and bone marrow. Cell Biochem Funct. 2013;31(1):20–9.PubMed
7.
Stolzing A, Coleman N, Scutt A. Glucose-induced replicative senescence in mesenchymal stem cells. Rejuvenation Res. 2006;9(1):31–5.PubMed
8.
Li YM, Schilling T, Benisch P, Zeck S, Meissner-Weigl J, Schneider D, Limbert C, Seufert J, Kassem M, Schütze N, Jakob F, Ebert R. Effects of high glucose on mesenchymal stem cell proliferation and differentiation. Biochem Biophys Res Commun. 2007;363(1):209–15.PubMed
9.
Weil BR, Abarbanell AM, Herrmann JL, Wang Y, Meldrum DR. High glucose concentration in cell culture medium does not acutely affect human mesenchymal stem cell growth factor production or proliferation. Am J Physiol Regul Integr Comp Physiol. 2009;296(6):735–43.
10.
Prunet-Marcassus B, Cousin B, Caton D. From heterogeneity to plasticity in adipose tissues: site-specific differences. Exp Cell Res. 2006;312(6):727–36.PubMed
11.
Gimble JM. Adipose tissue-derived therapeutics. Expert Opin Biol Ther. 2003;3(5):705–13.PubMed
12.
Zuk PA, Zhu M, Ashjian P, De Ugarte DA, Huang JI, Mizuno H, Alfonso ZC, Fraser JK, Benhaim P, Hedrick MH. Human adipose tissue is a source of multipotent stem cells. Mol Biol Cell. 2002;13(12):4279–95.PubMedCentral
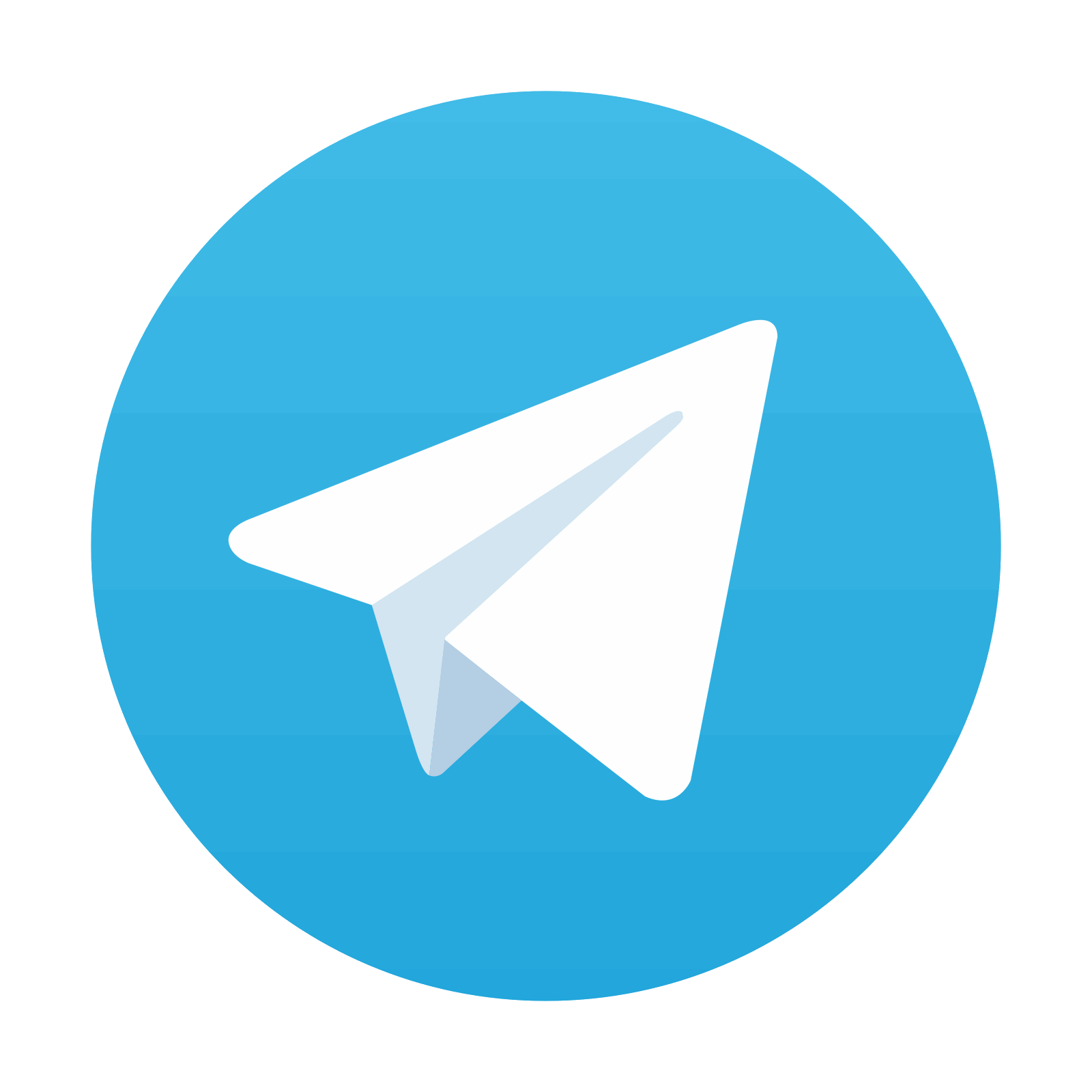
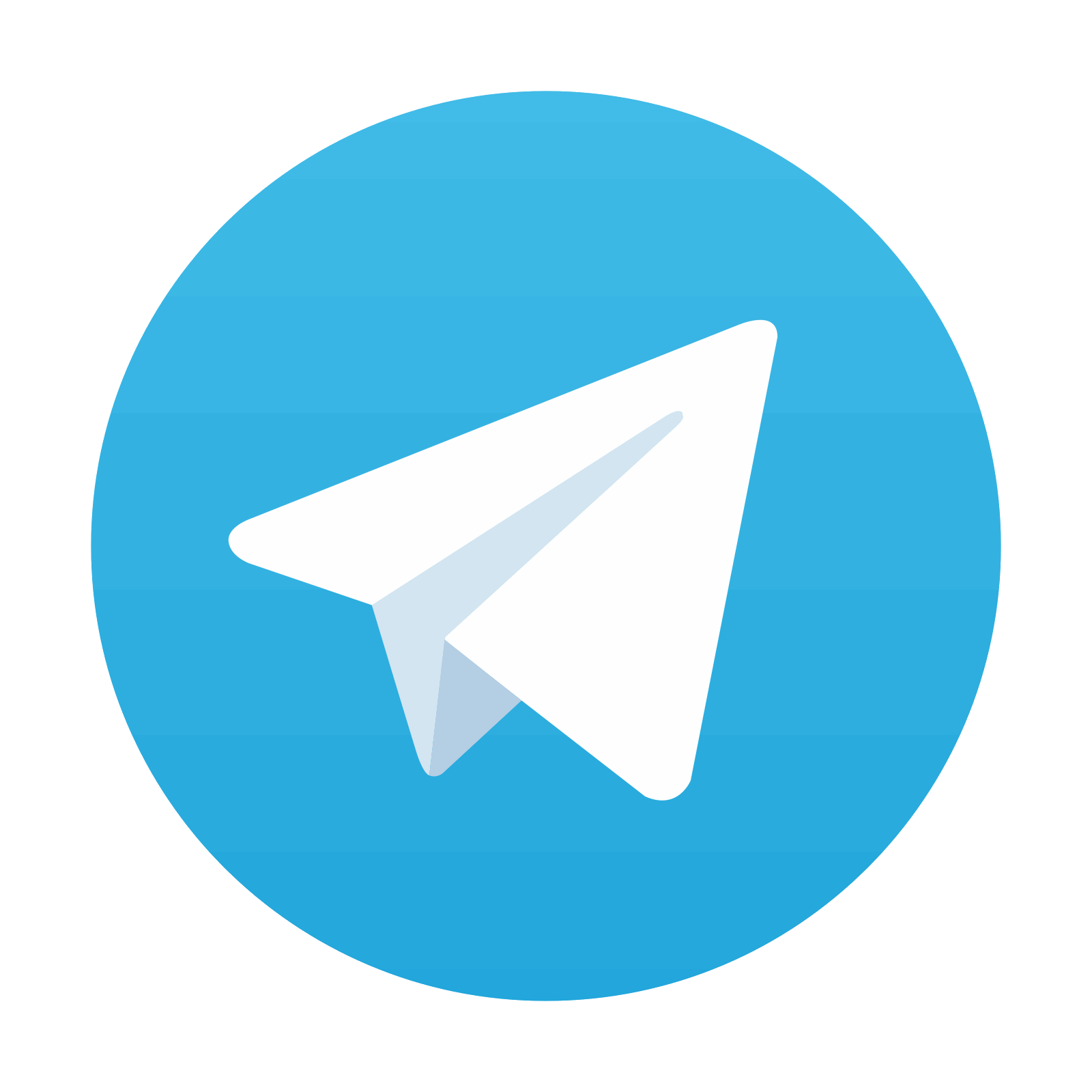
Stay updated, free articles. Join our Telegram channel

Full access? Get Clinical Tree
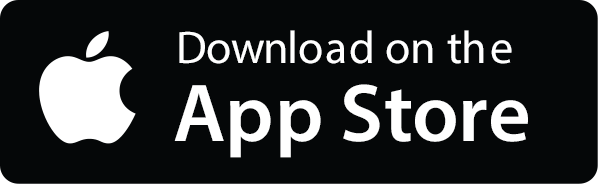
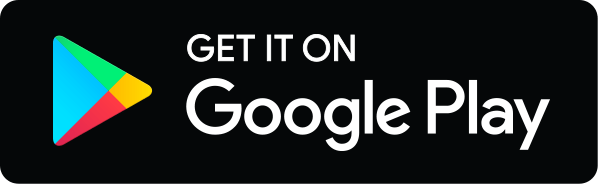