17 Skin graft
Synopsis
History of skin grafting mirrors many of the important advances of plastic surgery.
There are complex biologic mechanisms that allow skin grafts to take.
There are a variety of types of skin grafts that can be selected based on clinical application.
Technique should be adjusted to avoid most complications.
There are exciting areas of research that will help minimize donor sights and improve function and appearance of skin grafts.
Historical perspective
Gaspare Tagliacozzi, one of the first reconstructive surgeons (1545–1599),1 described pedicled skin flaps from the arm for nasal reconstruction in patients while at the same time research was conducted by Giuseppe Baronio (1750–1811), who performed and published for the first time skin grafts in a lamb (Degli innesti animali, 1804).2 Jonathan Warren in Boston and Joseph Pancoast in Philadelphia were among the first to perform and describe autologous full-thickness skin grafting in humans using the arm as a donor site. Paul Bert, a French politician, showed in his thesis that skin graft survival is only possible if blood vessels of the recipient site are able to revascularize the graft. His work popularized this technique.3 For the techniques, Reverdin, a French student, described harvesting skin islands with the scalpel tip with transfer to a first web space wound.4 He discovered that these skin islands not only temporarily covered, but also actively stimulated, wound healing. Reverdin’s work was rapidly accepted and performed by multiple surgeons in the US. The term “dermoepidermoid” graft was introduced by Leopold Ollier (1830–1900), who also first used the split-thickness skin graft (STSG) to close entire wounds.5 He noticed that healing was achieved faster and wound contraction was minimized. The German surgeon Carl Thiersch (1822–1895) further developed the technique of skin grafting and introduced the concept of wound bed preparation by removing granulation tissue to achieve a clean wound bed to facilitate graft revascularization. The English ophthalmologist, John Reissberg Wolfe (1824–1904), from Glasgow, published the use of a full-thickness skin graft for ectropium correction.
With the development of skin harvest techniques the number of publications reporting clinical results of skin grafts increased exponentially. Later, James Carlton Tanner (1921–1996) revolutionized burn surgery by the introduction of meshing to expand skin grafts in order to cover larger wounds with minimal donor site morbidity.6
Reverdin’s technique was modified by John S Davis (1872–1946), who elevated the skin with a needle in 1914 to facilitate harvesting, a technique that he called “pinch graft.”4 In 1920 Ricardo Finochietto (1888–1962) developed a knife to elevate larger skin areas manually by controlling the thickness of the skin graft. Ten years later, the invention of the shave blade by Humby further facilitated skin harvest.
Anatomy and physiology
Stem cells and regeneration of skin
Basal epithelial keratinocytes are the committed stem cells of the epidermis. Constant self-renewal provides a new protective layer at the skin surface.7–12 Hair follicles contain multipotent stem cells that are activated upon the start of a new hair cycle and upon wounding to provide cells for hair follicle and epidermal regeneration. In the hair follicle stem cells reside in the bulge area. Bulge cells are relatively quiescent compared with other cells within the follicle.9,10 However, during the hair cycle, bulge cells are stimulated to exit the stem cell niche, proliferate,13 and differentiate to form the various cell types of the hair follicle.14 In addition, bulge cells can be recruited during wound healing to support re-epithelialization.15,16
Hair follicles
Hair differentiates in a craniocaudal direction, 9 weeks after gestation, as mesenchymal cells populate the skin to form the dermis. Specialized cells in the dermal layer stimulate epithelial cells to proliferate and migrate downward into the epidermis, forming hair canals. The complete developed hair follicle contains an ectoderm-derived matrix and an underlying mesoderm-derived follicular papilla. There are three bulges that attach to the hair bulk. At the base the erector muscle develops, the middle part gives raise to the sebaceous gland, and the superficial bulge develops into an apocrine unit (Fig. 17.1).
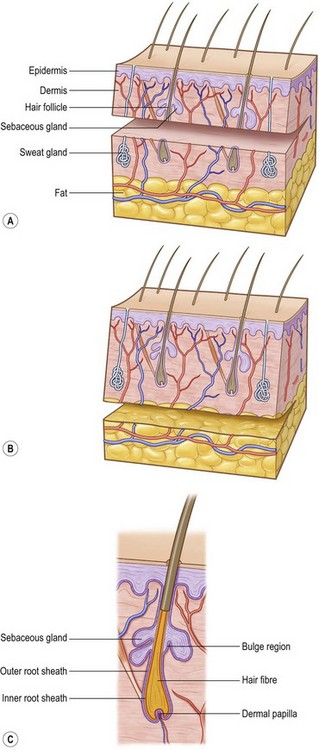
(Revised from Orgill, D. P. Excision and skin grafting of thermal burns. N Engl J Med 360: 893–901, 2009.62)
Although no new hair follicles are made postnatally, the lower portion of the hair follicle regenerates in order to produce new hair. Some of this capacity has been linked to the presence of multipotent epithelial stem cells. These cells can be found in the lowest permanent portion of the hair follicle – the bulge.13 Bulge stem cells are activated during the transition from telogen to anagen, to restart hair growth.
Science
Mechanisms of skin graft take
Skin grafting is the transfer of autologous skin cells left in anatomic order but without an intact blood supply. Therefore time and the recipient surrounding conditions limit the vitality. The operative procedure allows for nearly immediate coverage of large wound areas. Meshed grafts allow further expansion of skin but leave multiple small wounds that are re-epithelialized, mainly from the mesh within a few days. Skin can also be expanded through multiple small skin island grafts (as in Reverdin’s technique) that stimulate granulation tissue, probably by excreting growth factors. In STSGs, keratinocytes on the basal layer show high proliferation rates, which may ultimately stimulate growth factor excretion.17
Three phases of skin graft take are commonly described: (1) serum imbibition; (2) revascularization; and (3) maturation (Fig. 17.2).
Serum imbibition
In the first days, before the graft revascularizes, oxygen and nutrients diffusing through the plasma between the graft and the wound bed will nourish the skin graft. Huebscher in 188818 and Goldmann in 189419 theorized that skin grafts might be nourished by host fluid before vascularization of the graft occurs. They referred to this as “plasmatic circulation.”18,19 Later, Converse et al.20 altered the term to “serum imbibition,” as fibrinogen changes into fibrin that fixes the skin graft on to the wound bed in the absence of real plasmatic flow. Converse’s studies show that skin grafts gain up to 40% of their initial weight within the first 24 hours after grafting and then this gain is reduced to 5% at 1 week postgrafting.20 In the first hours, passive absorption of serum from the wound bed causes edema, which resolves when the revascularization is functional (Fig. 17.2).
Revascularization
Revascularization is critical for long-term skin graft survival. Early studies in the 19th century21–24 suggested a connection between the wound bed and graft vessels, referred to as inosculation,18,19,25,26 but the mechanism of revascularization remained unclear for many years.
Three hypotheses of revascularization are supported by the literature, each of them probably contributing to the process: anastomosis, neovascularization, and endothelial cell ingrowth. Anastomosis is the process of reconnection between the blood vessels in the recipient site wound bed and the graft.21,23,24,27–29 Neovascularization is characterized by new vessel ingrowth from the recipient site into the skin graft. The last mechanism describes endothelial cell proliferation and sliding from the recipient site, utilizing pre-existing vascular basal lamina as a structure, while in the graft endothelial cells gradually degenerate.22,24,30–32
The process of revascularization begins as early as 24–48 hours after grafting.33,34 Many authors describe vessel ingrowth mainly from the wound bed and less so from the wound margins, since no significant increase in blood vessels was seen in graft margins after skin grafting.21,24,33–36 Studies supporting vessel ingrowth from the host as the main mechanism of skin graft revascularization have been controversial with respect to time course and the mechanism of host–graft vessel interactions.23,37 Some early studies demonstrated by intravenous injection with radioisotopes that blood flow in the graft was established 4 days after grafting.38 Similarly, studies using India ink showed graft vessel stain as early as 2 days postgrafting.23 More recently, it was demonstrated, using a transgenic tie2/pacZ mouse model, that vessel ingrowth appears in the periphery of the graft (following blood vessel regression in the graft) from day 3 until day 21.39 Zarem et al.22 suggested that the process of vascularization of full-thickness skin grafts in the mouse is dominated by vascular ingrowth from the recipient using a modified transparent skin chamber. Henry and Friedman37 proposed the theory that endothelial cells of superficial graft vessels degenerate and that host vessels profit from the basement membrane-covered infrastructure for new vessel ingrowth. In 1967, other investigators confirmed this theory using the graft hamster cheek pouch and showed a similar vessel pattern before and after grafting.40 Converse and Ballantyne further investigated endothelial cell ingrowth into the graft using diaphorase, a marker of viable vascular endothelium. They found increased diaphorase levels in the graft bed 4 days after grafting, supporting the theory of vascular ingrowth from the host. As very few functional anastomoses were present, the authors concluded that both mechanisms, inosculation and vascular ingrowth, were important in the process of revascularization.32 Vessel regression was also supported by NADH diaphorase activity loss during the first 4 days after grafting that was probably taken up by new vessel ingrowth.30,39,40 The conclusion that endothelial cells utilize preformed tunnels of basal lamina was triggered by the observation that initially empty graft vessels subsequently became infiltrated with leukocytes and that ingrowing vessels used the white blood cell-filled channel as a conduit.22 Later studies showed a central refilling of the graft vasculature as early as 48 hours, leading to the conclusion that early ansastomosis between host and graft vessels may play a major role in graft revascularization, as vessel growth takes about 5 µm/hour and angiogenesis would take at least 5 days to reach the 600-µm-thick murine dermis.22,41 In 1987 Demarchez et al.42 supported this hypothesis by grafting athymic mice with human STSGs. Double labeling of cross-reacting antifactor VIII and a human specific antitype IV collagen antibody showed initial anastomosis between graft and host vessels. Murine host cells gradually replaced vascular structure and the extracellular matrix of the skin graft. Later studies confirmed this hypothesis by observing a similar blood vessel network of the skin graft at the donor site and after revascularization of the graft between 96 and 120 hours after grafting.33
Capla et al. further showed that about 20% of blood vessels supporting the microcirculation on day 7 after grafting occurred by bone marrow-derived endothelial progenitor cell-derived vasculogenesis.39 Recent studies measured increased levels of growth factors related to hypoxia-induced angiogenesis (hypoxia-inducible factor-1 and vascular endothelial growth factor) 24–240 hours after grafting, a process that may also contribute to host vessel ingrowth (Fig. 17.2).33
Skin appendages and functional structures
Hair follicles, sweat glands, and dermal nerves can often be transferred within thick, STSGs and full-thickness skin grafts (Fig. 17.1). Thin STSGs will not allow the transfer of hair or other adnexal glands, as the regenerating bulb is not harvested. Hair regrowth can occur in STSGs but, due to the shallow depth of harvest, is rather unlikely. Full-thickness and composite grafts will show hair regrowth 2–3 months after grafting.