(1)
Department of Dermatology, Freiburg University Medical Center, Freiburg, Germany
Abstract
Shortly after 1901, when the rules of Mendelian inheritance had been rediscovered, the concept of mosaicism was developed in both plants and animals. Mosaic patterns were described first in maize and citrus fruits, and subsequently in apple and pineapple. Between 1925 and 1940, twin spots were documented in maize and apple, and they were induced by x-rays in Drosophila. In the second half of the 20th century, twin spotting was reported in other plants such as tomato and tobacco, and it was experimentally induced in soybean and snapdragon. During the fifties, Barbara McClintock developed the concept of epigenetic mosaicism by studying ears of maize. In 1961, Mary Lyon discovered the mechanism of X inactivation that represents a particular form of epigenetic mosaicism. During the seventies, the lines that Alfred Blaschko had described in 1901 were rediscovered and gained general recognition as a possible pattern of mosaicism in human skin. Several other mosaic patterns of cutaneous mosaicism were delineated during the nineties of the past century. – From a clinical point of view, it is difficult but important to discriminate mosaic phenotypes from similar segmental patterns reflecting chimerism. A mosaic originates from one zygote, whereas a chimera originates from the fusion of two or more different zygotes.
Virtually all human skin disorders, including common diseases such as psoriasis, drug eruptions, or acne, sometimes display mosaicism. It is important to realize, however, that mosaicism also occurs as a physiological phenomenon. All human beings represent, to some degree, mosaics because today we know that mutual monoallelic expression of genes gives rise to functional mosaicism in both women and men. In other words, humans always display epigenetic mosaicism. On the other hand, all mammalian organisms will develop, during their lifetime, various forms of genomic mosaicism that may originate from diverse mechanisms such as postzygotic new mutation, mitotic recombination resulting in loss of heterozygosity, or other forms of allelic loss. Most of these mechanisms have first been studied in plants or animals. Conversely, some forms of mosaicism such as the superimposed segmental manifestation of both autosomal dominant and polygenic traits have initially been recognized in human disorders.
2.1 Historical Beginnings
During the first half of the twentieth century, mosaic patterning was first described in plants and subsequently in animals. Concepts explaining mosaic phenotypes of animals and plants were already proposed shortly after 1901, the year when the Mendelian rules of inheritance had been rediscovered. In 1904, the zoologist Valentin Häcker [20] mentioned, in an article on animal breeding, the word “Mosaikbastarde” (mosaic bastards) to denote animals showing the traits of their parents arranged in a “mosaic-like distribution” on the various parts of their body. In 1913 Collins [5] used the term “mosaic” to describe a variegated pattern of seeds in maize. It took a rather long time, however, until the concept of mosaicism was also applied to human disorders.
2.2 Mosaicism in Plants
Mosaic patches are easily seen in plants (Fig. 2.1). Since the rediscovery of the rules of Mendelian inheritance in 1901, formation of mosaicism has been reported, initially under the term “somatic segregation” [32], in various species such as maize [5, 9, 10, 38], citrus fruits [16, 52], apple [8, 32], pineapple [6], tobacco [57], and Dahlia [33]. In 1935, Emerson et al. [11] suggested that mosaic seeds of maize “may also be interpreted as somatic mutations.”
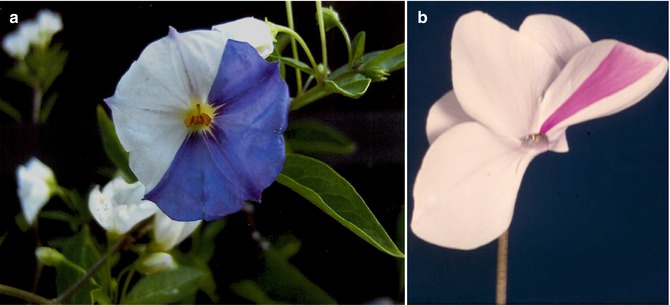
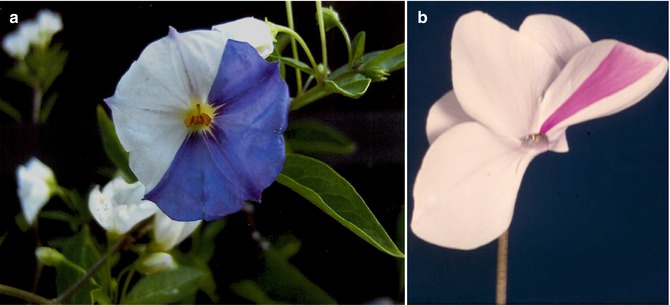
Fig. 2.1
Mosaic flowers in (a) Solanum species (Courtesy of Dr. Ulrich Langenbeck, Friedrichsdorf, Germany) and (b) Cyclamen species
In 1937, twin spotting in maize and apple (Fig. 2.2a) was comprehensively described by Donald F. Jones from New Haven, Connecticut, and interpreted as a result of somatic crossing-over [31]. He already distinguished allelic from nonallelic twin spotting and even described “twin spots within twin spots” in seeds of maize as well as “twin stripes” in oranges, maize kernels, and apples. He concluded that “paired stripes may be expected in all fruits and flowers that are heterozygous for color in the epidermis.” Jones even anticipated the concept of revertant mosaicism in the form of “reversal of recessive changes to the original dominant condition” when writing: “However, somatic crossing over offers no solution for recessive mosaics returning to the dominant condition. In a few cases this apparently does occur.”
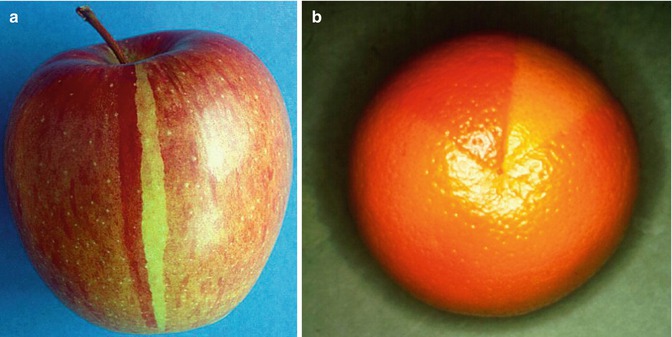
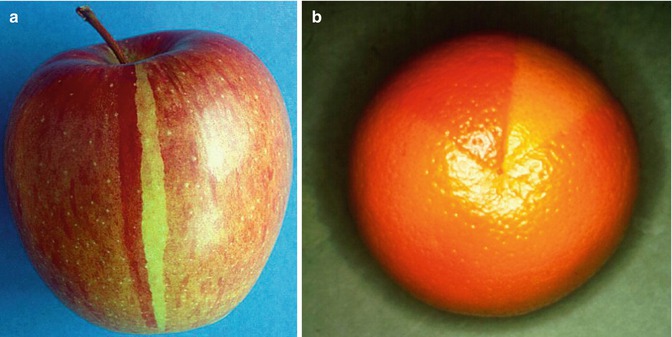
Fig. 2.2
Twin spotting (a) in an apple and (b) in an orange (b: Courtesy of Dr. Frédéric Cambazard, St. Etienne, France)
Twin spotting has been described in many other plants such as tomato (Fig. 2.3) [48], Dahlia species (Fig. 2.4) [33], tobacco [12], soybean [60], and snapdragon [27].
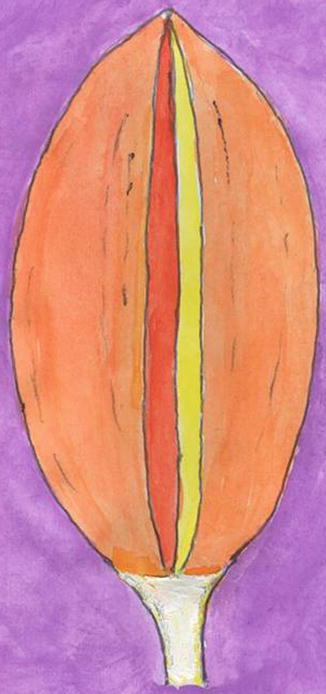
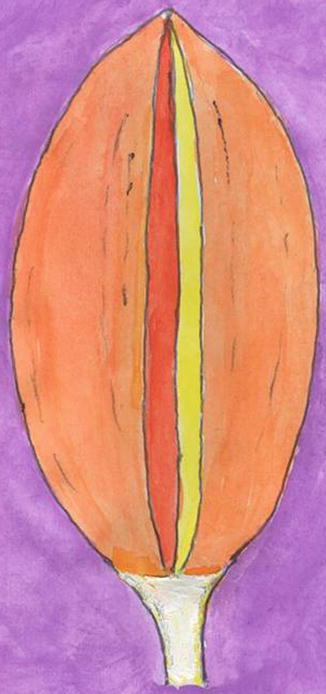
Fig. 2.4
Historical picture of twin spotting in a Dahlia petal. Painted from a figure published by W.H.J. Lawrence in 1929 [33]
By investigating mosaic traits in maize, Barbara McClintock [39] developed her concept of “jumping genes” and thus opened the new field of epigenetic mosaicism, an achievement for which she later received the Nobel Prize.
2.3 Mosaicism in Animals
During the first half of the past century, mosaic patterns were described in various species such as Drosophila [7, 40, 45], pigeon [28], budgerigar (Fig. 2.5) [54], domestic fowl [47, 51], rat [3], rabbit [46], and guinea pig [61]. Hollander [29] published a seminal paper on mosaic effects in domestic birds. In 1927, Curd Stern [55] reported on the experimental production of mosaicism in Drosophila.
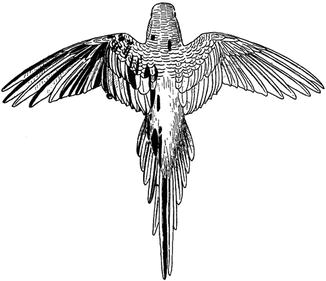
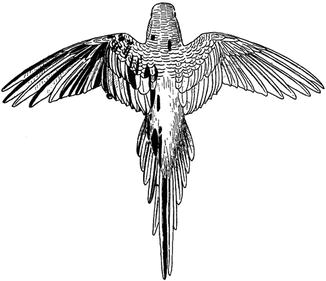
Fig. 2.5
Mosaic plumage of a budgerigar [54] (Reproduced with permission from Revue Suisse de Zoologie, Geneva, Switzerland)
In 1929, John T. Patterson [44], a zoologist from Austin (Texas), first described “twin areas” produced in Drosophila melanogaster by x-ray treatment of eggs or young larvae. In the integument he found paired spots being “adjacent or close to each other,” in the form of mutant clones of either “yellow” or “singed.” Analogously, he was able to produce, in the eyes of Drosophila, “twin areas” of different mutant colors [43]. Subsequently, somatic crossing-over to explain both single mosaic spots and twin spots was extensively studied by Stern [56].
Today, the Drosophila wing spot test as developed by Graf et al. [17] is used to test various chemicals for their mutagenic or recombinogenic effects or for their action of antigenotoxicity [18]. Larvae of the animal are exposed to the test substance, and subsequently the wings of the fly are microscopically examined for the presence of single or paired spots (Fig. 2.6). Moreover, the twin-spot technique can be used to study the function of imaginal disc epithelial cells in Drosophila [2]. Twin spots have also been induced experimentally in mice [13].
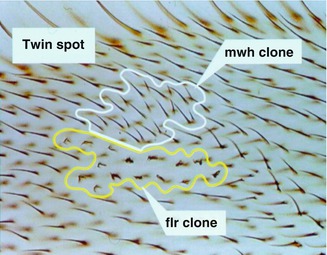
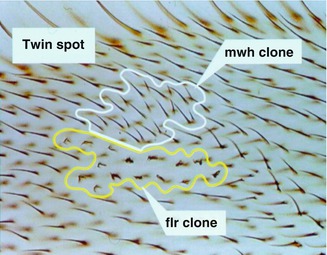
Fig. 2.6
Twin spotting in the Drosophila wing spot test. The two homozygous cell clones (mwh multiple wing hair, flr flare) originated from somatic recombination induced by a chemical mutagen (Courtesy of Dr. Hansjörg Frei, Zurich, Switzerland)
More recently, an FLP recombinase that recognizes sites consisting of short DNA sequences is used in Drosophila to induce postzygotic recombination resulting in mosaicism [34, 35]. Griffin et al. [19] developed a “twin-spot generator” technique that generates paired green and red spots being detectable already as single cells (Figs. 2.7, 2.8, and 2.9). The method has been used to investigate the cellular dynamics of eye or leg regeneration in imaginal discs of Drosophila [58]. By applying the same technique, Yu et al. [62] labeled the sister clones, derived from a common neuroblast, simultaneously in different colors within the antennal lobe of Drosophila instars. In this way they were able to visualize the complete developmental sequence of different neuronal lineages and thus to get more insight into the complex embryogenesis of the brain of Drosophila.
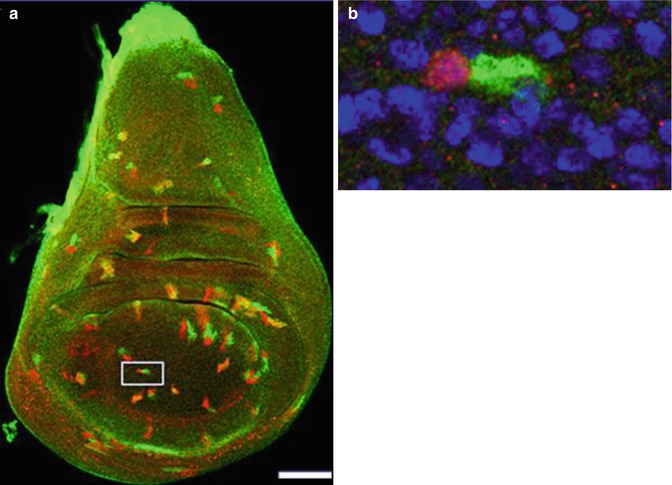
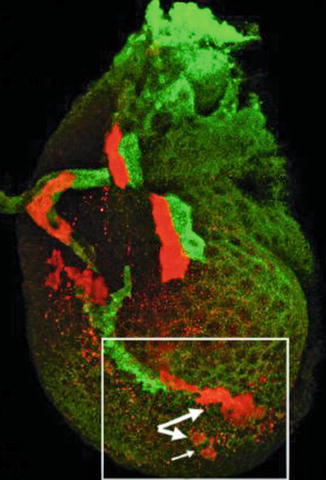
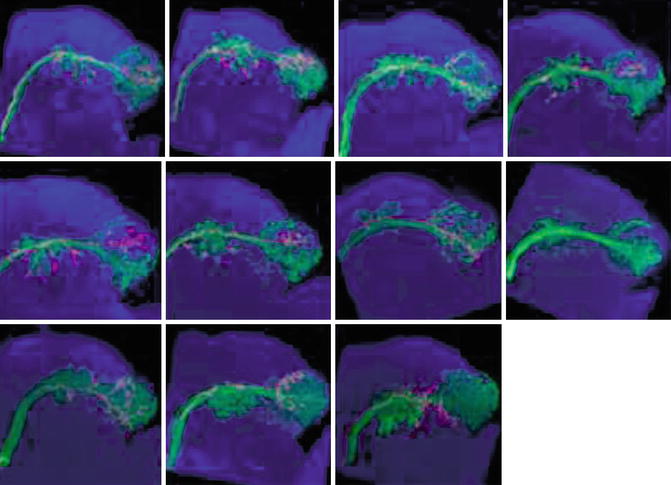
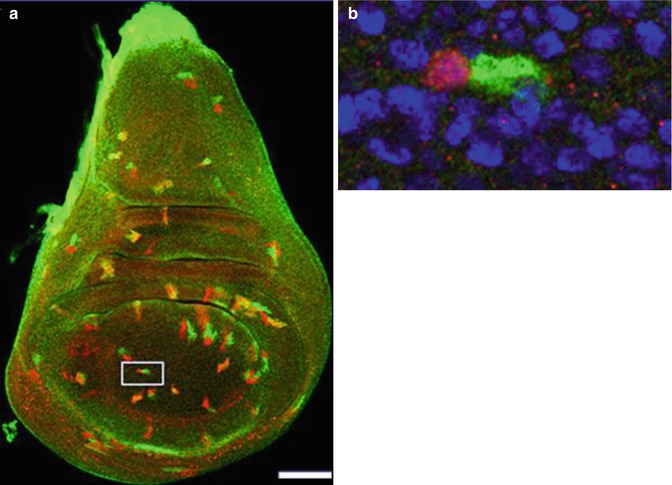
Fig. 2.7
(a) Imaginal wing disc of Drosophila showing green and red twin spots induced by the twin-spot generator technique. (b) Inset shows twin spot at the two-cell stage [19] (Reprinted with permission from Nature Publishing Group)
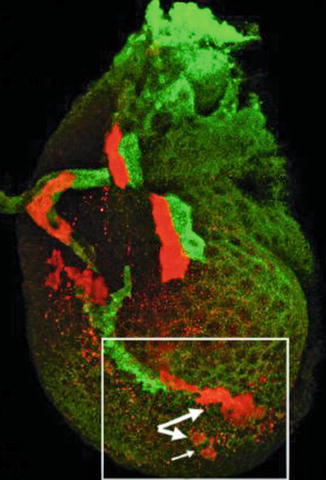
Fig. 2.8
Separation of clones in an imaginal leg disc of Drosophila, visualized by the twin-spot generator technique. Large arrows indicate separated clones. Small arrow indicates an almost separated clone [19] (Reprinted with permission from Nature Publishing Group)
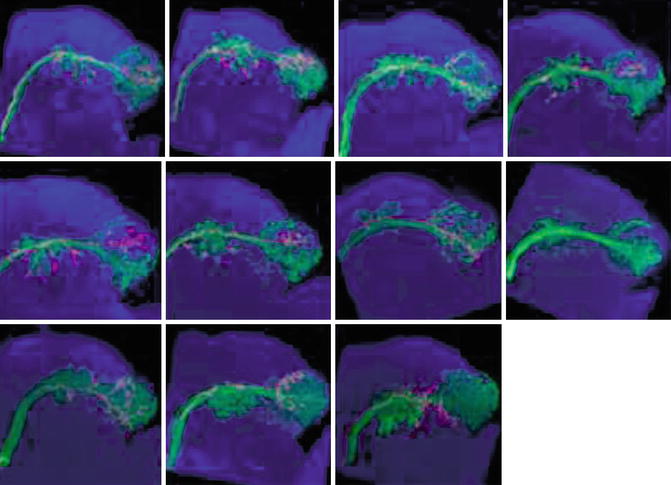
Fig. 2.9
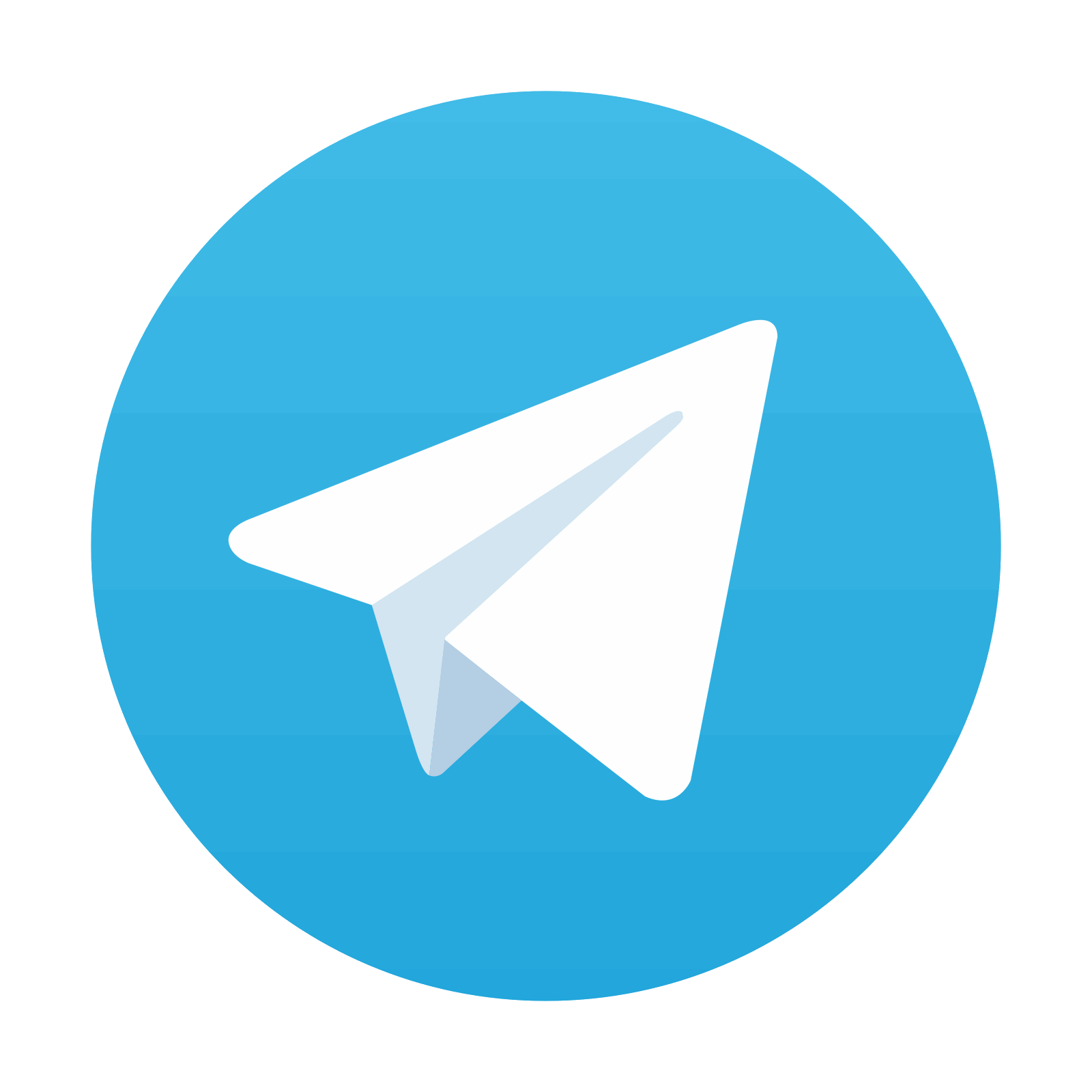
Identification of sequentially derived neurons in the antennal lobe of a Drosophila instar by twin-spot MARCM (mosaic analysis with a repressible cell marker) [62] (Reprinted under license of Creative Commons)
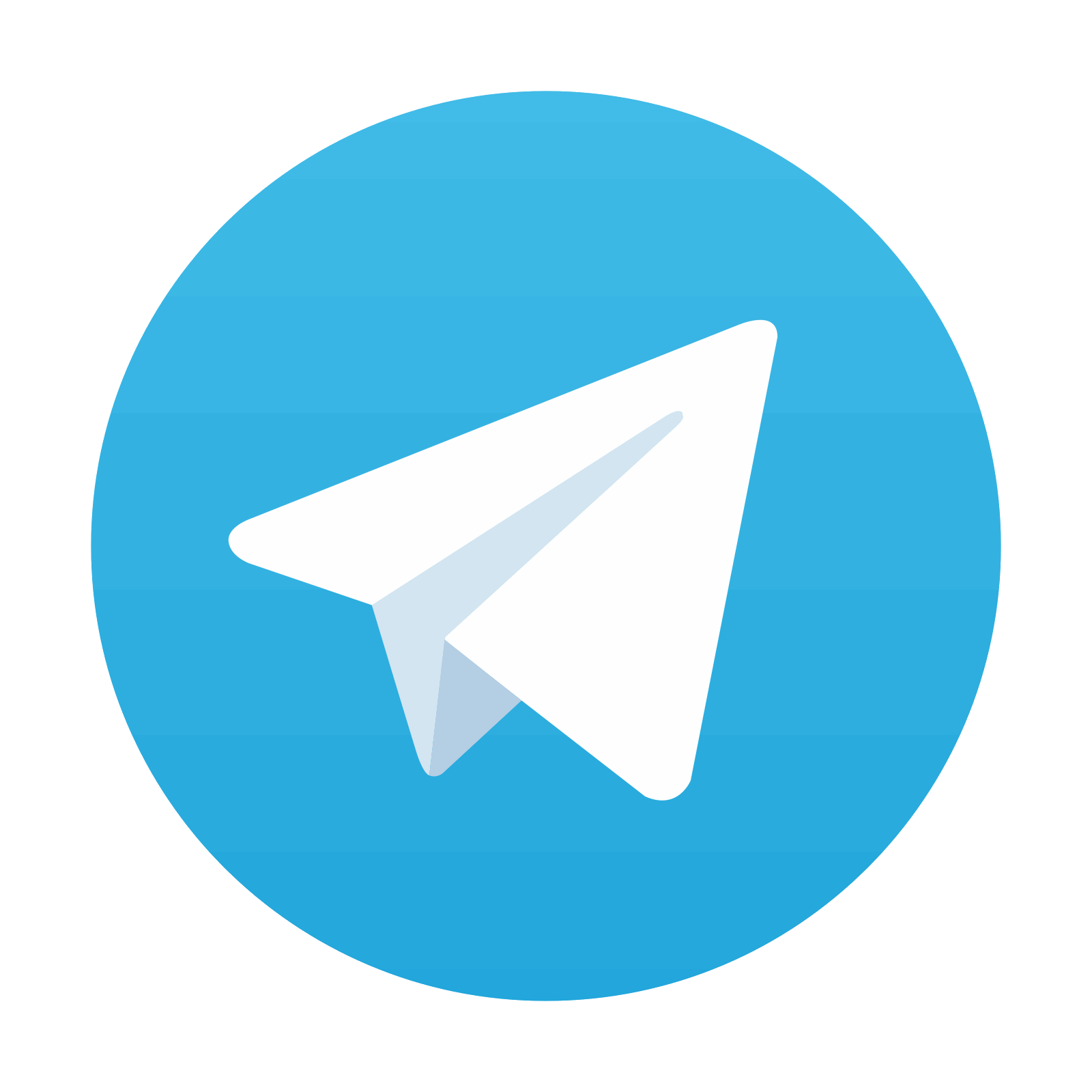
Stay updated, free articles. Join our Telegram channel

Full access? Get Clinical Tree
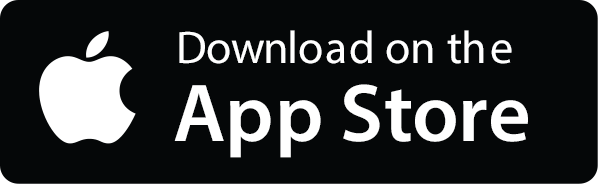
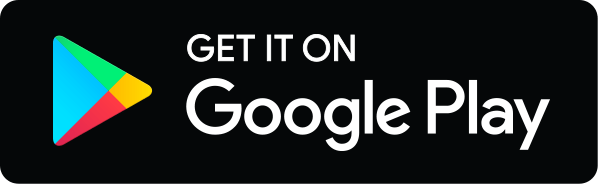
