The development of DNA technology and improved knowledge of the structure and function of the human genome have led to the identification of the causative genes responsible for the different forms of epidermolysis bullosa (EB) and provided the opportunity to determine the precise location and type of mutations present in EB patients, allowing diagnosis of this disease at the level of the defective gene itself. The large genetic heterogeneity of EB, however, precludes the direct use of molecular testing for EB diagnosis. In addition, only a few diagnostic or research laboratories in the world are equipped to perform mutational screening, which is still labor intensive and associated with considerable costs, because most mutations are unique to one or a few families. This article reviews the most popular methods used in EB molecular analysis.
The development of DNA technology and improved knowledge of the structure and function of the human genome have led to the identification of the causative genes responsible for the different forms of epidermolysis bullosa (EB) and provided the opportunity to determine the precise location and type of mutations present in EB patients, allowing the diagnosis of this disease at the level of the defective gene itself. The large genetic heterogeneity of EB, however, precludes the direct use of molecular testing for EB diagnosis. In addition, only a few diagnostic or research laboratories in the world are equipped to perform mutational screening, which is still labor intensive and associated with considerable costs, because most mutations are private (ie, unique to one or a few families). The laboratory diagnostic approach to EB involves at first the use of immunofluorescence epitope mapping (IFM) and transmission electron microscopy (TEM) examination of a skin biopsy to determine the level of skin cleavage and the presence of morphologic alterations of epithelial adhesion structures and the defective expression of specific protein components. IFM and TEM enable definition of the major EB type and, in most cases, the subtype and identification of the protein component targeted by the mutations. These analyses define the candidate genes for mutation screening and they must precede molecular testing, which should be primarily considered when (1) prenatal or preimplantation diagnosis is being planned, (2) the mode of genetic transmission cannot be delineated from the family pedigree in combination with IFM and TEM findings, and (3) a gene-replacement therapy protocol is foreseen (see the article by Sybert elsewhere in this issue for further exploration of this topic).
Mutation identification in a given family is required for prenatal diagnosis, although linkage analysis using markers (either intragenic polymorphisms or tightly linked microsatellites) for the mutant gene may be an alternative method when the mutation is unknown (see the article by Sybert elsewhere in this issue for further exploration of this topic). This approach cannot be applied, however, to EB types presenting locus heterogeneity (ie, junctional EB [JEB] and EB simplex [EBS]). Prenatal testing by linkage analysis is restricted to dystrophic EB (DEB) families, because all DEB cases result from mutations in a single gene, COL7A1. Prenatal diagnosis by indirect DNA analysis requires the availability of DNA samples from several family members (at least of both parents and a previously affected child) to identify the marker allele that segregates with the mutant gene in the kindred.
Besides its crucial relevance for prenatal diagnosis, mutation identification is recommended to provide accurate genetic counseling regarding the mode of inheritance and the risk of disease recurrence in sporadic cases presenting with mild to moderate DEB, which can result from either dominant de novo or recessive inherited mutations.
Molecular testing can also be an excellent way to confirm the diagnosis for very rare EB variants caused by mutations that invariably occur at the same nucleotide position. A typical example is the recurrent dominant missense mutation at nucleotide 74 (c.74C>T/p.P25L) of keratin 5 gene causing EBS with mottled pigmentation.
Finally, patient genotyping can be required when Kindler syndrome is suspected. Kindler syndrome diagnosis is difficult to achieve in the newborn and infant because of the clinical overlap with other forms of EB. Moreover, TEM and IFM findings on a skin biopsy can be insufficiently specific to establish a diagnosis of Kindler syndrome. Mutational screening of the causal gene FERMT1/KIND1 represents the best technical option for Kindler syndrome diagnosis confirmation.
When considering molecular testing in the diagnostic approach of an EB patient, it should be kept in mind that the sensitivity of any gene screening procedure is not 100%. The mutation detection rate in the most complex and frequently analyzed gene, COL7A1, can vary from 60% to 95% depending on the screening technique used. The detection rate in other genes, such as those encoding for laminin-332 (LAMA3, LAMB3, and LAMC2), integrin α6β4 (ITGA6 and ITGB4), collagen XVII (COL17A1), and plectin (PLEC1), ranges from 83% to 95%, as deduced from mutational studies of large patient cohorts performed in molecular diagnostic laboratories worldwide. The mutation detection rate seems to be lower (67%) in keratin genes. Personnel involved in genetic counseling should inform affected families asking for molecular testing about the sensitivity of the mutation screening strategy performed. The inability to detect a mutation can be intrinsic to the resolving power of the chosen method or related to the mutation site, which could lie outside the coding exons and flanking intronic junctions (ie, within noncoding regulatory elements or deep intronic regions that are usually not screened). In addition, all the polymerase chain reaction (PCR)–based methods applied in routine diagnostics are unable to detect large deletions or duplications encompassing the entire gene or one or more exons.
This article reviews the most popular methods used in EB molecular analysis.
Sources of genetic material
Mutational and linkage analyses are performed using DNA from the proband and his or her relatives. Among the latter, the most important samples are those of the patient’s parents. DNA can be extracted from any tissue. In EB diagnostics constitutional DNA is usually obtained from the white cells present in a blood draw, which is stored in tubes containing EDTA. Standard sampling is 5 mL of EDTA blood from adults and children and 2 to 3 mL from infants, although adequate DNA amount can be obtained from 0.2 mL of blood or less. Whenever a blood draw cannot be performed, buccal swabs may represent an alternative source to obtain constitutional DNA. The DNA amount retrieved might be insufficient for the screening of large genes, however, such as COL7A1. DNA can also be extracted from the skin biopsy used for immunomapping investigations or from keratinocyte-fibroblast cell cultures derived from the skin biopsy. Cultured cells also represent a source of messenger RNA (mRNA), which in many circumstances is useful to verify mutation consequences on splicing of the pre-mRNA. In addition, total RNA can be used as the target molecule for mutational screening procedures (see later). DNA extraction from lesional and nonlesional skin can be of interest if revertant or somatic mosaicism is suspected. Sperm is the proper source of constitutional DNA to verify the presence of a known mutation in the proband’s father when the occurrence of a paternal gonadal mosaicism has to be proved.
Screening of unknown mutations
Mutational detection strategies have been developed for all genes involved in EB. Preliminary to mutation search is the choice of the gene to be analyzed. In particular, EB types showing locus heterogeneity, such as JEB and EBS, can require screening of more than one gene. IFM findings can allow identification of the defective protein (eg, laminin-332 or collagen XVII or α6β4 integrin in JEB) and guide mutation search. Nevertheless, IFM is not adequate to distinguish the mutant polypeptide subunit in altered multimeric proteins. In these cases, multistep testing can be devised. When a gene coding for a chain subunit of a multimeric protein is more frequently targeted by mutations compared with the genes coding for the other subunits, then that gene should be screened at first. In JEB with laminin-332 deficiency, molecular analysis should start with LAMB3 testing, thereafter continue with LAMC2, and terminate with LAMA3. In JEB with pyloric atresia, ITGB4 should be screened at first, followed by ITGA6, which is very rarely mutated.
Mutation scanning techniques are based on PCR amplification of target DNA or RNA sequences. Because missense, nonsense, and splice site mutations represent the most common types of nucleotide changes underlying EB, the screening strategies have been designed to detect point mutations. Selective and rapid amplification of each exon and intron-exon boundary is obtained using genomic DNA (gDNA) as a template and specific primer pairs that anneal on flanking intronic sequences. Alternatively, overlapping primer pairs that anneal on exons and cover the entire coding region can be used when reverse transcribed mRNA (complementary DNA [cDNA]) is chosen as a template. The latter strategy depends on the availability of an RNA source, which in turn implies laboratory facilities and tools for setting up primary keratinocyte-fibroblast cell cultures. Because mRNA comprises all the coding regions but does not contain intervening sequences, the advantage of a cDNA-based screening strategy resides in the amplification of a reduced number of PCR fragments.
Conversely, gDNA-based screening strategies imply, in case of genes with many large introns, the amplification of a greater number of PCR products, each encompassing one or a few exons and flanking sequences. Blood from which the gDNA is extracted is easy to obtain, however, whereas procedures for deriving primary cultured cells are time consuming and more expensive. Mutation search on gDNA is favored by most of the diagnostic laboratories.
Genomic DNA-based strategies are available for all genes involved in EB, whereas cDNA-based strategies have been developed for a limited number of genes, in particular the largest ones, such as COL7A1, ITGB4, ITGA6, LAMB3, and LAMC2. Seventy-two primer pairs (72 PCRs) are commonly used to amplify all 118 COL7A1 exons using gDNA, whereas the entire COL7A1 coding region is comprised in only 22 cDNA amplicons. To amplify ITGB4, ITGA6, LAMB3, and LAMC2 exons, 38, 26, 23, and 23 primer pairs are usually used with gDNA as a template, whereas 12, 9, 8, and 8 PCR fragments can be generated from cDNA, respectively.
Molecular testing of keratin 14 gene (KRT14), which is involved in EBS, is complicated by the presence of two inactive pseudogenes, whose sequences could be coamplified with functional KRT14 sequences. Current gDNA-based strategies for KRT14 mutational screening have been conceived to avoid pseudogene amplification. These protocols imply single-step allele-specific PCR with primers that specifically anneal on functional KRT14 sequences, or exploit restriction sites on gDNA to exclude the pseudogene sequences from the subsequent PCR.
Once chosen, the target molecule’s gene segments are amplified using PCR; then, the most accurate way to characterize them is to obtain the exact DNA sequence. In this manner, aberrant variations can be identified and their presence confirmed in family members. Direct sequencing can be labor intensive because of the size of the gene but, especially when a cDNA-based strategy is used, it represents a relatively simple and reliable technology endowed with high sensitivity (<95%). Moreover, if a gene has been extensively screened and a large mutation database is already available, “priority” strategies based on gDNA amplification and direct sequencing of priority regions, which are defined according to the number and frequency of the reported mutations, can be devised. Priority screening is a general principle that also works well in genotyping new patients belonging to specific populations with a well-characterized constellation of recurrent and unique mutations. This strategy may speed diagnosis and improve the mutation detection rate in highly complex genes necessitating the generation and sequencing of many amplicons, such as LAMA3, LAMB3, LAMC2, and COL7A1 genes.
Nevertheless, systematic DNA sequencing has the disadvantage of being expensive and time-consuming for analysis of genes with a large exon number, such as COL7A1. Alternative strategies have been developed over time with the purpose to identify a single amplicon for sequencing. These include single-strand conformation polymorphism (SSCP), denaturing gradient gel electrophoresis (DGGE), conformation sensitive gel electrophoresis (CSGE), denaturing high-performance liquid chromatography (DHPLC), and protein truncation test (PTT). By using adapted apparatus, the first four methods are able to detect local mismatches within heteroduplex DNA molecules that form following hybridization between wild-type and mutant DNA ( Fig. 1 A). Their sensitivities vary greatly depending on the size of the amplicon screened. SSCP and CSGE have low detection levels (<70%–75%), but are easy to use. DGGE and DHPLC are more complex to set up, but once optimized they are very efficient with a mutation detection rate of up to 95%, approaching that of DNA sequencing. PTT is conceived for screening very big genes using either RNA or DNA and can detect only mutations that lead to protein truncation. It is important to consider that these methods allow the identification of point variations, which can be either true mutations or polymorphisms without any relation to the disease. The pathogenic role of a sequence variant can be obvious by looking at its nature, position within the gene, and frequency. If doubts remain, functional tests at the mRNA and protein levels should be considered.

SSCP
This method works well with small PCR fragments (<150 bp), which need to be denatured, and then run in nondenaturing polyacrylamide gels. During electrophoresis, the mobility and migration of single-stranded and reannealed double-stranded DNA molecules depend on both their size and the conformation assumed on the basis of nucleotide sequence composition. If two alleles differ for a point mutation, the PCR fragment spanning this variation is expected to result in four types of single-stranded and two types of double-stranded molecules that appear on a gel as four bands with a different migration speed. If the two alleles are identical, only two bands are observed. SSCP has been applied to mutational screening of COL7A1 in DEB families.
DGGE
The method has the best performance with fragments of up to 500 bp. It allows the detection of point variations within double-stranded DNA fragments (heteroduplex analysis) run on a gel containing an increasing denaturant concentration (gradient gel). In this condition, homoduplex PCR fragments resulting from the reassociation of complementary strands of one allele have different mobility and migration in respect to heteroduplex PCR fragments formed between two alleles, which are different for the presence of the mutation. Optimization of this method requires that the gradient of denaturing agent concentration be adapted to the sequence of the analyzed gene fragments. Also, this method is used for COL7A1 mutation detection.
CSGE
This method is expected to have high sensitivity for fragments of up to 600 bp. Experimentally, it was shown to yield mutation detection rates of up to 75% when applied to COL7A1 screening. CSGE was developed for heteroduplex analysis in a modified polyacrylamide gel electrophoresis that enhances the tendency of single mismatch to produce conformational changes in mildly denaturing environment. It allows one to discriminate homoduplexes from heteroduplexes of PCR fragments in virtue of their differential electrophoretic mobility. Protocols for mutation detection by CSGE have been reported for most EB genes.
DHPLC
This is an efficient, semiautomatic, high-throughput system for fragments of up to 600 bp. For these reasons, DHPLC technology is being increasingly used in EB diagnostics. In particular, it has been optimized for molecular diagnosis of DEB and JEB. The principle through which the DHPLC functions is the identification of heteroduplex DNA molecules formed by mixing, denaturing, and reannealing the PCR products amplified from alleles differing in sequence because of point mutations (see Fig. 1 ). The PCR fragments are automatically loaded on a polystirene column and eluted with an acetonitrile gradient buffer. Under partial denaturing conditions, fragments containing mismatches (heteroduplexes) are released from the column easier than perfectly matched fragments (homoduplexes). The DHPLC apparatus records the elution profiles of amplicons by an ultraviolet lamp and shows them as chromatographic peaks. By comparing the elution profile of an amplicon generated from the testing sample with the one from a standard homozygote, aberrant heteroduplex profiles can be easily recognized, and the corresponding PCR product sequenced to precisely identify the mutation.
PTT
This method consists of synthesizing a double-stranded cDNA by reverse transcription of the total RNA using primers that encompass specific regions of the studied gene. Using a forward primer that contains the phage T7 promoter as an extra sequence, the freshly synthesized cDNA fragment can be transcribed and then translated in vitro using a coupled rabbit reticulocyte lysate system in the presence of tRNA labeled with radioactive amino acids. The in vitro synthesized peptides are resolved according to their size by electrophoresis on a denaturing gel, and then transferred onto a membrane by Western blot. Finally, the membrane is autoradiographed and the size of the peptides corresponding to the screened sample is determined by comparison with control peptides synthesized by a wild-type gene. A DNA-based PTT protocol has been developed and widely used for the detection of truncating mutations within the large exon 32 (3.3 kb) and 33 (7.2 kb) of the PLEC1 gene in EBS with muscular dystrophy. In addition, PTT has had a limited application for screening COL7A1 mutations in patients with recessive DEB.
From what has been reviewed, it is evident that all currently available technologies have individual merits and limitations. Assessment of sensitivity degree of each method is in absolute not so obvious and the success in detecting a sequence variation often relies on the possibility for a given diagnostic laboratory to combine different scanning techniques. Future approaches that could ease EB mutation detection include the automated resequencing of entire genes using customized microarrays spotted with partially overlapping oligonucleotides spanning the sequence of the target genes. Customized genechips are suited for hybridization with gene segments PCR-amplified from the DNA sample to be tested. Appropriate software has been conceived to analyze the hybridization signals on chips and to convert them in DNA sequence. The advantage of the genechip approach consists of the possibility that a single array can assemble up to 300 kb of sequence, allowing the analysis of multiple genes (in theory all the EB genes).
Current mutation scanning methods cannot detect large deletions or duplications encompassing the entire gene or involving gene segments. Such rare complex DNA rearrangements have been reported in a few EB patients. For the characterization of these cases different screening strategies have been conceived. These make use of several research tools, which comprise Southern or Northern blots, long-range PCR amplifications, real-time quantitative PCRs, and fluorescent in situ hybridization analysis. A combination of these techniques is always needed to precisely define the mutation. They cannot be routinely used in diagnostics and their application remains confined to the research field of EB molecular genetics.
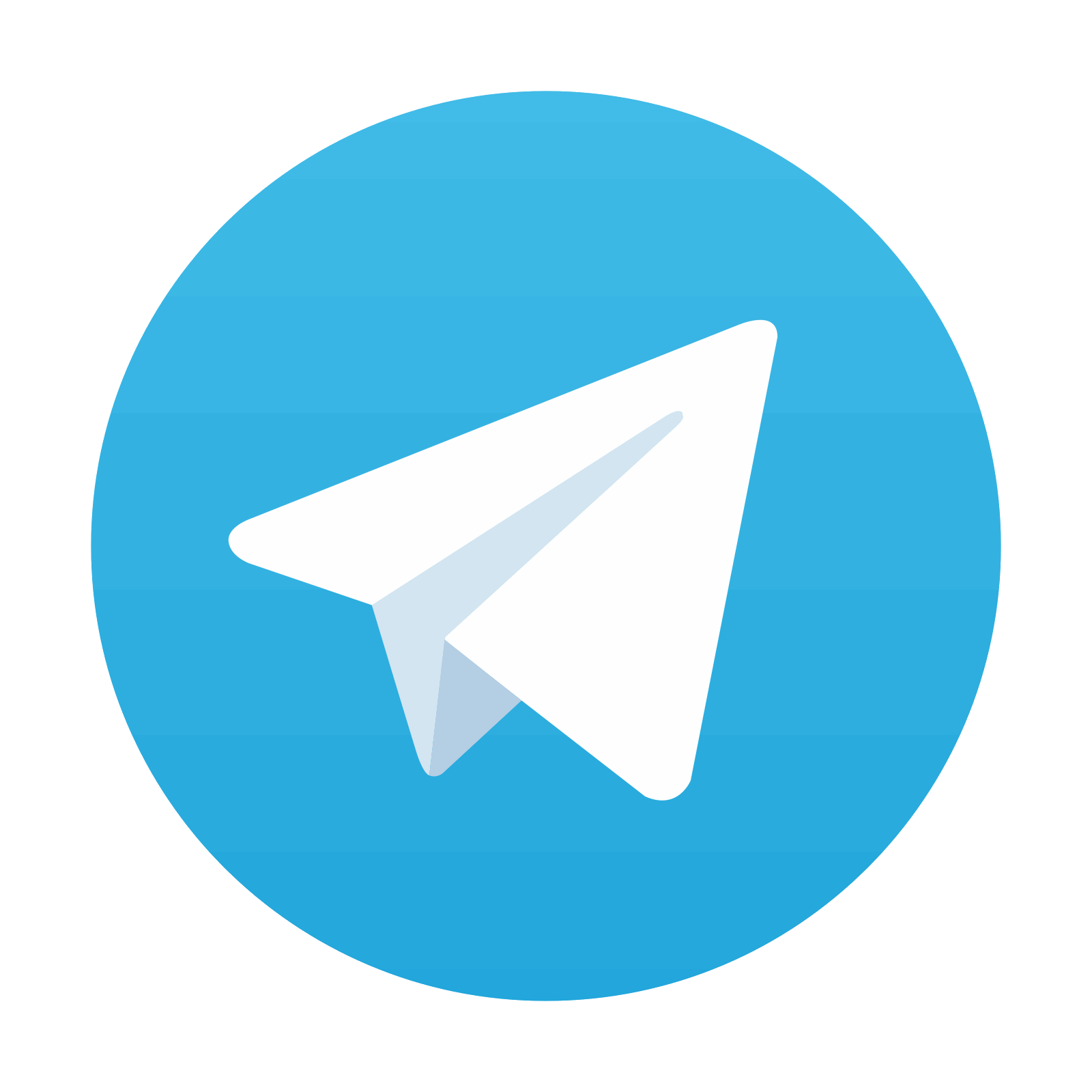
Stay updated, free articles. Join our Telegram channel

Full access? Get Clinical Tree
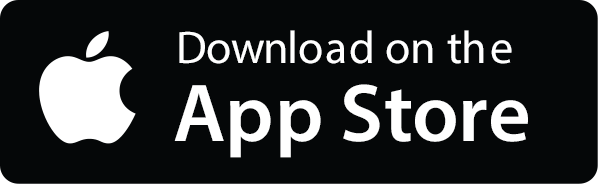
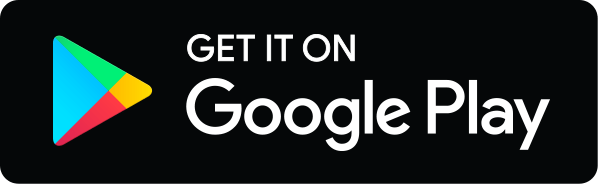