The main goal of reconstructive microsurgery must be an optimal functional and esthetic reconstruction meeting the individual trauma site requirements with minimal donor site morbidity. The authors discuss new microsurgical options for extremity salvage: indications for reconstruction versus amputation, timing of free tissue transfer, reconstruction of soft tissue and bone, and functional muscle transfer. They discuss indications and contraindications for these procedures, along with emphasizing the important points of each.
- •
Isolated complex extremity injury requires immediate specialized attention via an interdisciplinary approach.
- •
Key steps in surgical management include radical tissue debridement, adequate fracture stabilization, and reconstruction of viable structures—if necessary, by use of autologous blood vessels or nerve grafts.
- •
A key step in surgical management and one of the most powerful tools for infection control is an early and radical debridement of devitalized, and potentially contaminated, tissue.
- •
Although still considered essential components in reconstructive surgery, local flaps and skin grafting are often associated with an increased rate of wound complications and compromises concerning results, enabling new techniques of free tissue transfer, functional composite free flaps, and preexpanded and chimeric flaps to become better options in extremity salvage surgery.
Introduction
During the past 40 years, evolution in reconstructive microsurgery has substantially influenced limb salvage surgery. Despite continuous improvement in trauma management leading to a significant reduction in primary limb amputations, complex injuries involving extremities remain a particular challenge for reconstructive surgeons. In the past, limb salvage procedures were often impended by local tissue status; modern methods of microsurgical free tissue transfer now allow an early, “custom-fit” wound management, aimed to optimally meet the individual functional and esthetic requirements of the affected trauma region. State-of-the-art reconstructive microsurgery has opened a wide range of possibilities in limb salvage surgery allowing immediate fracture and soft tissue repair with only minimal compromise in functional outcome. Key steps in surgical management include radical tissue debridement, adequate fracture stabilization, and reconstruction of viable structures (if necessary, by use of autologous blood vessels or nerve grafts). Subsequently, tissue defects should be covered by sufficient well-vascularized tissue, optimally matched to meet the individual tissue requirements at the recipient site. The authors outline the impact of recent microsurgical advances on extremity salvage and reconstruction.
Initial preoperative assessment
Initial stabilization and diagnosis should be performed via a multidisciplinary approach according to the established Advanced trauma life support protocol. For extremity trauma, immediate attention primarily targets hemorrhage control. Once the patient is stabilized, a focused clinical evaluation is performed. Assessment of injured extremities must consider trauma mechanisms, wound dimensions, and all functional components (soft tissue, nerves, vessels, and bones). These components must be examined individually and in combination.
Radiographic imaging should also be focused on the trauma zone including directly adjacent joints. In case of potential vascular involvement, Doppler sonography or angiography is essential for evaluation and planning of surgical treatment, especially because vascular damage can potentially exclude the use of local flaps.
Initial preoperative assessment
Initial stabilization and diagnosis should be performed via a multidisciplinary approach according to the established Advanced trauma life support protocol. For extremity trauma, immediate attention primarily targets hemorrhage control. Once the patient is stabilized, a focused clinical evaluation is performed. Assessment of injured extremities must consider trauma mechanisms, wound dimensions, and all functional components (soft tissue, nerves, vessels, and bones). These components must be examined individually and in combination.
Radiographic imaging should also be focused on the trauma zone including directly adjacent joints. In case of potential vascular involvement, Doppler sonography or angiography is essential for evaluation and planning of surgical treatment, especially because vascular damage can potentially exclude the use of local flaps.
Indications for reconstruction versus amputation
Indications for limb salvage procedures versus primary amputation must be evaluated individually for each patient, considering not only local trauma site but also patient age, presence of other concomitant injuries, patient comorbidities, patient socioeconomic status, and patient motivation. Despite major advancements in reconstructive microsurgery, severe lower extremity trauma (Gustilo IIIB-IIIC) is still associated with amputation rates of up to 40%. In general, indications for microsurgical free flap reconstruction include the following :
- •
Trauma-induced injures with substantial bone and muscle defects
- •
Osteomyelitis
- •
Deep burns
- •
Tumor excisions
- •
Vascular disease
The most commonly used classification system for lower extremity trauma has been the Gustilo classification, introduced in 1976 and subsequently modified in 1984 ( Table 1 ). This classification analyzes not only wound dimensions and the presence or absence of neurovascular injury but also wound contamination and trauma energy impact.
Gustilo Classification | Definition |
---|---|
Type I | Wound <1 cm, minimal contamination, low-energy and simple fracture |
Type II | Laceration >1 cm; moderate soft tissue damage with adequate bone coverage |
Type IIIA | Extensive soft tissue damage, massive contamination but adequate bone coverage |
Type IIIB | Extensive soft tissue damage with periosteal stripping and bone exposure, flap coverage is usually required |
Type IIIC | Arterial injury associated and requiring repair |
Numerous algorithms have been established, designed to estimate the viability of damaged tissue and to assist in determining whether amputation is necessary or whether limb salvage of traumatized extremities is recommended and promising. These include the Mangled Extremity Severity Score, the Limb Salvage Index, the Predictive Salvage Index, and the Hannover Fracture Scale. Besides providing an analysis of local tissue status, these scores also specifically consider arterial and venous vascular injury as a prognostic factor and warm ischemia and shock time. A prospective evaluation of 556 consecutive patients from 2001 on showed that, because of high specificity, low scores reliably predicted limb salvage potential. In contrast, because of low sensitivity, trauma evaluation scores did not reliably identify patients possibly requiring an amputation. Current data suggest that these scores can be useful tools in the decision-making process (when used cautiously). They should not be used as the principal means for reaching difficult decisions. From an economic point of view, unless amputation is inevitable, surgeons should always consider limb salvage, which will yields lower costs and higher utility compared with amputation.
Special attention must be paid to complete traumatic limb amputation. If the patient’s life is at stake, the principle of “life before limb” will obviously be the primary objective. In this case, immediate replantation remains fairly uncommon. Indications for primary amputation may include advanced patient age, severe crush injuries with complete transsection of essential anatomic structures, prolonged warm ischemia time, or presence of potentially life-threatening concomitant injuries. In the case of traumatic amputation, warm ischemia can be tolerated up to 8 hours and cooling may extend the time-frame for replantation to 24 hours. A study published in 2005 demonstrated that plantar sensation is no longer a limiting prognostic factor when evaluating possible limb salvage, because it showed comparable functional outcomes in plantar sensation between patients initially lacking and patients with permanently preserved plantar sensitivity.
Thus, a surgeon must perform realistic risk-benefit stratification to determine whether amputation is justified. When considering salvage procedures, expectations must be tempered by a realistic assessment of potential outcome, because protracted attempts for limb salvage may have a negative impact on the patient’s social, physiologic and psychological subsistence. In some cases, limb amputation may even be the preferable strategy because it may reduce the duration of hospitalization and rehabilitation. Several studies even suggest that patients with primary amputation show equally good functional outcomes as those who underwent limb reconstruction. The lower extremity assessment project demonstrated reconstruction results in 2-year outcomes equivalent to those of amputation.
In conclusion, individual patient assessment remains the key step in determining whether limb salvage or primary amputation is indicated. Through a careful risk-benefit assessment, surgeons should try to optimally meet outcome expectations while keeping morbidity at the lowest possible level.
Timing
Since Marco Godina introduced the concept of the “emergency” free tissue transfer in the 1980s, surgical treatment of severe tissue trauma has experienced a substantial change. Early, fastidious trauma zone debridement followed by immediate restoration of affected longitudinal structures and early defect coverage by transferring adequately vascularized tissue has proved to reduce the incidence of osteomyelitis and fracture nonunion. In his study from 1986, Godina showed that delayed microsurgical reconstruction following trauma (between 72 hours and 90 days) was associated with the highest risk of infection and flap loss. Thus, he proposed the concept of “early” microsurgical reconstruction within 72 hours after trauma. Despite the proved advantage, the optimal timing for free tissue wound coverage remains controversial. Several consecutive studies analyzing the correlation between time until reconstruction and complication rate or functional outcome proved to be consistent with Godina’s initial results, showing that early microsurgical reconstruction significantly reduced bone union time, number of surgical procedures, and infection rate. In contrast, a study from 2008 showed no potential disadvantage associated with delayed lower extremity reconstruction. Byrd and colleagues demonstrated the importance of early soft tissue coverage after removing all devitalized tissue, thereby preventing bacterial colonization. Complex and contaminated wounds should be converted into surgically clean wounds to allow primary closure.
There remains a wide and misleading variety of terms used for defining windows during which posttraumatic free flap wound closure is recommended. To reliably compare reconstructive results between individual institutions, a standardized terminology for the timing of free flap coverage is essential. Thus, Ninkovic and colleagues implemented a simple and versatile terminology, analogous to the established nomenclature defining the phases of normal wound healing.
According to this terminology, flap closure timing is subdivided into 3 categories:
- 1.
“Primary free flap closure” (12–24 hours)
- 2.
“Delayed primary free flap closure” (2–7 days)
- 3.
“Secondary free flap closure” (>7 days)
Primary flap cover for crucial closure prevents further tissue damage caused by desiccation and facilitates vascular ingrowth from the new surrounding soft tissue. Well-vascularized muscle free flaps provide healthy tissue, thereby allowing a radical debridement of the trauma zone. Because the primary goal in the treatment of complex extremity injury is a quick and functionally optimal recovery, the treatment of choice is the primarily free flap cover within the first 24 hours after injury. This minimizes morbidity, tissue infection rate, requirement for secondary surgical procedures, rehabilitation time, and total duration of hospital stay.
Primary free flap closure with primary reconstruction is defined as a combination of definite functional reconstruction of longitudinal structures (bone, vessels, nerves, and tendons using grafts if it is necessary) combined with free or pedicled flap coverage following surgical debridement as a single-step procedure within a period of 24 hours ( Figs. 1 and 2 ).
Delayed primary reconstruction is defined by free flap defect closure within 2 to 7 days after the trauma. Again, definite reconstruction of longitudinal structures can be performed within this time frame or later according to the wound condition and type of injury. It is important to consider that by delaying timing of definite defect closure, surgical options for reconstruction of longitudinal structures are significantly reduced without possibilities to replace the missing structure (eg, bone, tendon, nerve) with graft because of a higher rate of infection. However, this coverage allows the possibility of starting immediate physical medicine and, soon, definitive secondary reconstruction ( Figs. 3 and 4 ).
Secondary free flap closure signifies closure with a free flap later than 1 week after the trauma. In general, reconstruction of soft tissue and temporary bone stabilization may be achieved. However, definite reconstruction of longitudinal structures and bone defects is performed later ( Figs. 5 and 6 ).
Surgical technique
Radical Debridement
A key step in surgical management and one of the most powerful tools for infection control is an early and radical debridement of devitalized, and potentially contaminated, tissue. Debridement should be performed like resection of malignancy, which means through healthy tissue and always under tourniquet control. It minimizes blood loss during debridement and alleviates evaluation of traumatized tissue. Tendons and essential neurovascular structures should be debrided and preserved, provided they are intact. In case of damage or complete transsection, essential structures should be primarily repaired either through direct coaptation or via interposition of vessels, tendon, nerve, or bone grafts.
Fracture Stabilization
For skeletal stabilization, external fixation is the initial method of choice. In case of minimal soft tissue destruction and wound contamination, primary internal fixation can be performed.
Since the imposition of microsurgery in the 1960s, the traditional concept of the “reconstructive ladder” has undergone a rapid and substantial evolution. In the past, the primary objective was a simple wound closure using local flaps and skin grafts. Modern microsurgery allows reconstruction of complex bone and soft tissue defects with excellent esthetic and functional outcomes. Thus, the reconstructive ladder has undergone “surgical acceleration” toward becoming the “reconstructive elevator.” Although local flaps and skin grafting are still considered essential components in reconstructive surgery, they are often associated with an increased rate of wound complications and compromises concerning results. Consequently, the importance of local flaps is increasingly receding into the background. Today, local and pedicled flaps are still recommended for small to medium-sized defects, which cannot sufficiently be treated by direct closure or skin grafts. In case of complex extremity trauma, local flaps are often damaged and therefore unusable. Further compromise of a severely injured extremity by sacrificing local tissue should be avoided. Therefore, free tissue transfer provides the most appropriate repair for severely injured extremities.
Modern techniques range from supermicrosurgical free tissue transfer, functional composite free flaps, and preexpanded and chimeric flaps to innervated functional myocutaneous flaps. Today, through constant improvement in surgical technique and understanding of perforator anatomy, microsurgical free tissue transfer is considered a routine technique that opens almost endless possibilities in extremity salvage surgery.
Reconstruction of Soft Tissue
In general, there are 3 principal indications for free flap coverage of traumatized lower extremities: soft tissue defects in the distal third, extensive soft tissue defects at any level, and “salvage free flaps” in case of nonreplantable amputation. Because of the huge variety of flaps available for reconstruction, flap selection must aim to optimally meet the specific functional and esthetic requirements of the recipient site such as tissue volume and surface, vascular pedicle length, and possible functional exigencies. In general, free flaps consist of a composition of different tissues (bone, muscle, adipose, fascia, and skin); consequently, they are referred to as “composite free flaps.” Examples include (functional) myocutaneous, fasciocutaneous, or osteo(myo)cutaneous flaps. All flaps have unique characteristics in terms of functionality, durability, vascular supply, and blood flow. For instance, myo(cutaneous) flaps have a blood supply that is 3 times that of fasciocutaneous flaps. This improved perfusion has great influence on bone healing and infection resistance. Furthermore, muscle flaps provide large wound coverage and they can be separated longitudinally along the muscle fibers, allowing optimal coverage of defects with complex surface topography and obliteration of dead space. Fasciocutaneous flaps like the anterolateral thigh flap can be harvested as sensate flaps, allowing various combinations of tissue components. They additionally show excellent surface texture, allowing perfect tissue contouring with superb esthetic results. Their lack of bulk makes them especially applicable in areas requiring minimal-thickness soft tissue transfer for defect coverage. Also, several studies could demonstrate the positive impact of fasciocutaneous flaps on chronic osteomyelitic wounds. Therefore, there is an upcoming trend toward using cutaneous flaps in reconstructive surgery. A study from 2005 analyzed the versatility of free fasciocutaneous flaps as an alternative to free muscle flaps for the reconstruction of traumatic open tibial fractures. There was no statistical difference in terms of flap survival, rate of postoperative infections, chronic osteomyelitis, and stress fractures. Although free muscle transfer is still recommended for extensive 3-dimensional defects with a large dead space, fasciocutaneous flaps proved to be a reliable and effective alternative for covering traumatic open tibial fractures. Deepithelialization of fasciocutaneous flaps even allows obliteration of dead space in extensive defects, thus representing a feasible alternative to muscle flaps.
Special attention must be paid to the reconstruction of the highly sensitive weight-bearing area of the foot. Here, choice of flap remains controversial and is mainly determined by localization and extent of the foot defect. For restoration of weight-bearing areas, the use of free muscle flaps combined with split-thickness skin grafts is recommended. In non–weight bearing areas, fasciocutaneous flaps should be preferred. Because of its size, the latissimus dorsi free flap proved to be ideally suited for covering extensively damaged soft tissue. Used as a “cross leg free flap,” it even allows simultaneous coverage of both feet in a single-step procedure. A study from 1998 demonstrated lower rates of ulcerations with the use of free muscle flaps compared with faciocutaneous flaps. The glabrous skin surface of the foot sole and palm has very distinctive characteristics; it is thick and dense in structure and histologically shows a high concentration of sweat pores and nerve endings. A unique structure of adipose tissue stabilized by multidirectional fibrous septae ensures its capacity to withstand pressure and shearing force. Current clinical evidence shows no significant necessity for special sensory reconstruction, although it improves quality of life and reduces time for rehabilitation.
Although a variety of free flaps are commonly preferred for specific indications in extremity reconstruction, a fixed assignment of flaps for certain defects or flap standardization is not recommended. A key principle must be individual flap selection depending on individual recipient site requirements. Preoperative planning must consider patient age, anatomic and physiologic preconditions, dimensions, volume and composition of tissue required, pedicle length and diameter, possible need for motoric or sensory innervation, and donor site morbidity.
Supermicrosurgery, or “perforator-to-perforator surgery,” represents a modern technique of free tissue transfer in which the diameter of anastomosis does not exceed 0.8 mm. Donor site tissue is harvested in a superficial approach, leaving the underlying fascia intact and thereby, presumably, reducing donor site morbidity. Consequently, this dissection results in pedicles of limited length and caliber. Additionally, this technique uses small-caliber perforators as recipient site vessels, leaving major vessels unaffected. Subsequently, because of minimal pedicle length, in most cases, one cannot respect the basic principle of performing anastomosis outside of the trauma zone. A study from 2009 analyzed the versatility of supermicrosurgery for extremity reconstruction in 42 consecutive patients. Results showed the technique to be feasible and efficient in the hands of an experienced surgeon. The proposed benefits are described as a reduction in flap harvest time and donor site morbidity, an increase in the number of available perforators, and a decrease in swelling as a result of minimal muscle manipulation. In extremity reconstruction, however, these advantages must be critically weighed against the increased risk of anastomosis complications and flap failure caused by direct exposure to the trauma zone. If anastomotic failure occurs, surgical options for anastomosis repair and revision are usually limited because of minimal vessel dimensions. Consequently, patient safety and prevention of any potential surgery-related complications are of highest priority. Optimal outcome must not be compromised by a sophistication of operating techniques, and individual indication for a supermicrosurgical approach must be well justified.
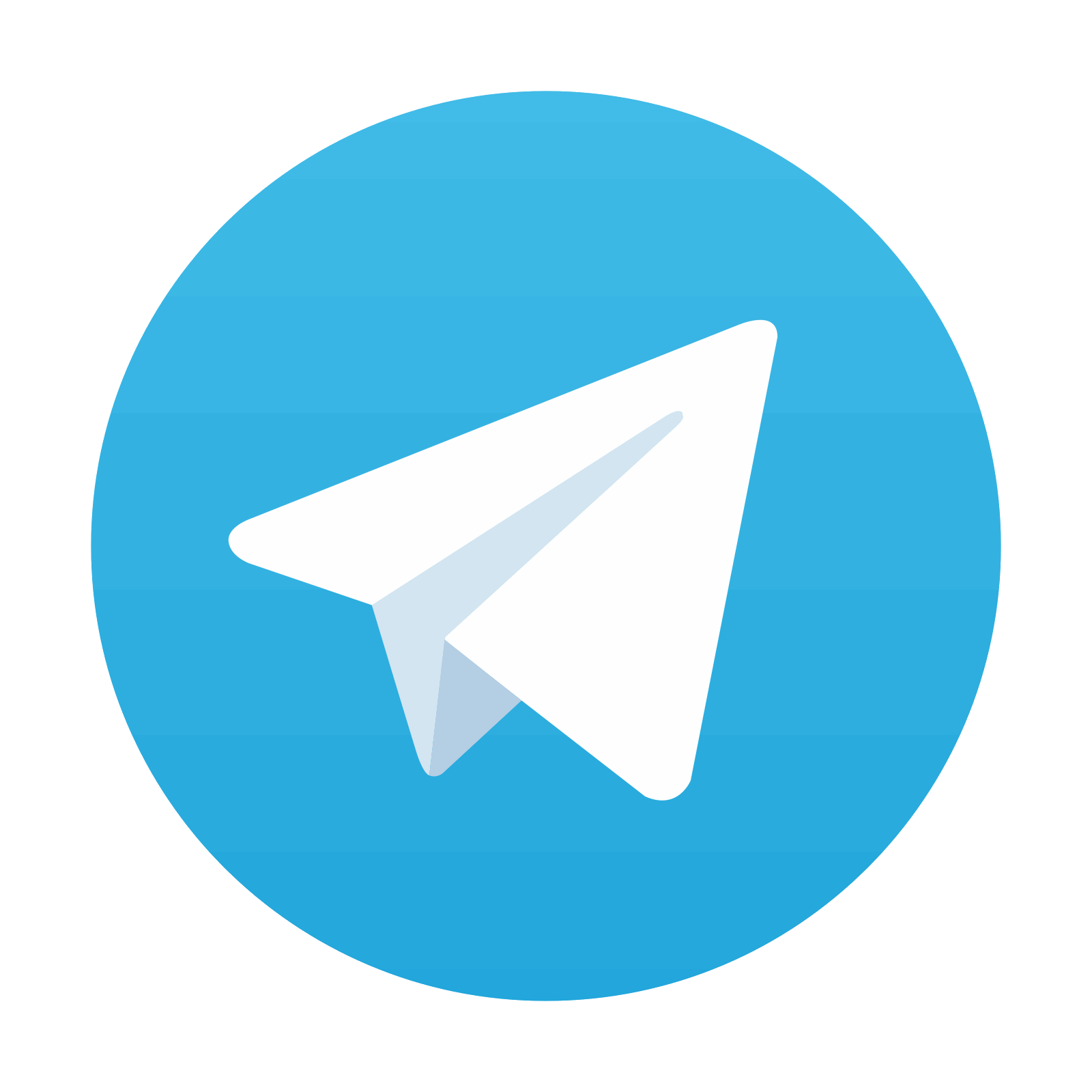
Stay updated, free articles. Join our Telegram channel

Full access? Get Clinical Tree
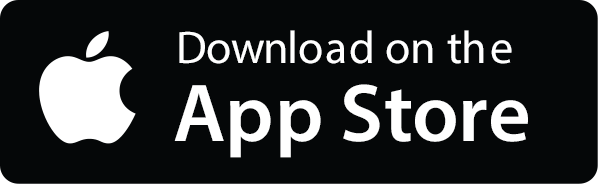
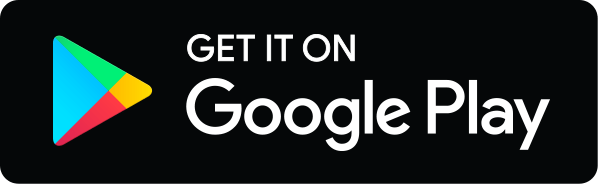