Q—Fluid filtration rate
Kf—Capillary filtration coefficient (capillary surface area x hydraulic conductivity)
Pcap—Capillary hydrostatic pressure
Pi—Interstitial hydrostatic pressure
σ—Reflection coefficient (Permeability)
πp—Plasma oncotic pressure
πi—Interstitial oncotic pressure
After a deep burn, the fluid filtration rate (Q) increases drastically. All components of the Starling equation contribute to the increase in capillary fluid filtration rate [5]. The capillary filtration coefficient (Kf) increases 2–3 times normal after a burn. Capillary hydrostatic pressure (Pcap) increases as a result of increased vasodilation in response to the injury, especially in superficial burns. Interestingly, interstitial hydrostatic pressure (Pi) becomes more negative because of the breakdown of proteins to create smaller and more osmotically active molecules that draw water into the interstitial space. Another theory to explain this negative interstitial pressure suggests that structural changes in collagen and hyaluron contribute to the negative interstitial pressure. The reflection coefficient (σ) clearly decreases with increased permeability. A reflection coefficient of 1 means that no molecule passes through the capillary wall and at 0 there is free flow of macromolecules. Normal reflection coefficient is 0.9 but the value drops after a burn. The plasma oncotic pressure (πp) drops as macromolecules, especially albumin leaks out of the capillary. The value drops by as much as 50% after a burn. The interstitial oncotic pressure (πi) increases as albumin and other large molecules escape.
There are other factors that affect the amount of fluid leaking across the capillary wall. The destruction of collagen leads to increased “interstitial compliance,” which in essence, means that there is more room for interstitial expansion. The most important factor that regulates the amount of edema is the rate of lymphatic resorption of fluids [6]. If lymphatic flow is decreased, such as with compartment syndromes, then less fluid is resorbed back into the intravascular space.

In this formula, πi (interstitial oncotic pressure) has been replaced with πg (subglycocalyx oncotic pressure). This endothelial glycocalyx layer is usually destroyed by local burn injury (and local sepsis) leading to greater fluid loss [10]. The capillaries of the uninjured tissue maintain this layer and thus do not have the same volume of fluid loss. This mechanism may partially explain why oncotic fluids have some efficacy in some part of burn resuscitation.
15.4 A Practical Guide to Burn Resuscitation
Burn shock resuscitation has not been perfected by any means. The fact that there are many different formulas and preferred fluids indicates that no method works very well. The debate on the appropriate volume and endpoints has been going on for over 50 years [11, 12]. The question of whether to use crystalloid versus colloid has also been debated for decades. It is clear that there have been more problems with providing too much fluid—a problem called “fluid creep” [1, 2, 13–15]. Much of the problem of over-resuscitation has been the result of failing to respond to indicators of over-resuscitation such as excessive urine output [2]. It is clear that providing too much fluid does lead to many complications such as respiratory failure and compartment syndromes. The initial resuscitation will influence the ultimate outcome of burn patients. Therefore, it is essential that caregiver optimize burn shock resuscitation.
It is intuitively obvious that fluid resuscitation should be dictated by the patient’s response to fluids. Unfortunately, the endpoints of burn resuscitation are poorly defined [11, 12, 16]. Clearly, formulas provide an estimate of fluid volume based on an “average” patient. But not all burns are the same. It is clear that more fluids are required for any patient who has a deeper burn, delay in resuscitation, smoke inhalation injury, other injuries or receives escharotomies. Drugs and alcohol, commonly associated with burns, also increase the amount of fluid required for resuscitation. The age of the patient also influences the volume required for resuscitation. Young children have higher daily fluid requirements per weight than adults [17, 18], so it is not surprising that their fluid requirements are greater per kilogram than adults. The elderly need fewer fluids than younger adults. Since it is expected that there would be so much variability, it is essential to have guidelines to direct fluid resuscitation.
If one plots the amount of fluid required during burn shock resuscitation, a curve is created with large amounts of fluids required at the start. The fluid requirements rapidly decrease as distant capillary leaks close, and over time, the leak rate slows to a stable maintenance rate. Clearly, the concept that a patient requires “half” of the fluids at 8 h is erroneous and this part of the Parkland Formula needs to be eliminated from the burn literature. The fluid rate must be reduced based on the patient response. The current standard is to target urine output as an indicator for adequate resuscitation. For adults the urine output target is 0.5 mL/kg/h and for children less than 30 kg the goal is 1.0 mL/kg/h. It is clear that urine output is not perfect but if one really reduces fluids to avoid urine output rates greater than those targets, most patients do fairly well. There have been attempts to develop other more accurate endpoints but as of yet, no great endpoints exist [16].
Parkland Formula : 4 mL/kg/%TBSA Burn
Start at a rate to give half during the first 8 h
Do not worry about the second 16 h, just decrease fluids to target urine output of 0.5 mL/kg/h
4 × 100 × 80 = 32,000 mL
Start rate to give half during the first 8 h = 16,000 mL or start at 2000 mL/h
Target urine output = 50 mL/h
If urine output is too low, increase fluids, and if urine output is high, reduce fluids. Since there is a concern for providing too much fluid, some guidelines (American Burn Association’s Advanced Burn Life Support) have suggested using the Parkland Formula at a lower rate (2 mL/kg/%TBSA burn) for superficial burns and raising the rate for patients with deeper burns [20]. The target of 2 mL/kg/%TBSA burn is what is recommended by the Brooke Formula. In actuality, the starting rate is of lesser concern as long as the fluid rate is adjusted based on the patient’s urine output and other physiologic responses.
Pediatric Burn Resuscitation:
Daily Basal Fluid Requirements + 4 mL/kg/%TBSA
Start rate to give half during the first 8 h
Do not worry about the second 16 h, just decrease fluids to target urine output of 0.5 mL/kg/h if >30 kg or 1 mL/h if <30 kg
Daily Basal Fluid Requirements
<30 kg = 2000 × Body Surface Area (BSA)
>30 kg = 1500 × Body Surface Area
or
100 mL/kg for the first 10 kg
+ 50 mL/kg for 10–20 kg
+ 20 mL/kg for >20 kg
Daily Basal Fluid Requirements (using either formula)
2000 × 0.5 (BSA) = 1000 mL/day or by the other formula
100 m/kg × 10 kg = 1000 mL/day
Parkland Formula = 4 × 10 × 50 = 2000 mL
Total Resuscitation Volume = 1000 mL + 2000 mL = 3000 mL in 24 h
Give half in the first 8 h = 1500 mL/8 h = 187.5 mL/h
Target urine output = 10 mL/h
15.5 Endpoints of Burn Shock Resuscitation
Currently the most commonly used endpoint of resuscitation is urine output [19] but it is clear that that guideline is fairly inaccurate. It is common for uninjured people to have periods of low urine output during periods of exercise or mild dehydration—without any sequelae. The same can be said for burn patients who can tolerate short periods of urine output. The philosophy of “permissive hypovolemia” [21, 22] or “permissive hypooliguria” [23] is practiced in many places where fluids are not increased for urine outputs as low as 0.3 mL/kg/h for short periods. There are also periods during burn resuscitation when the patient has greater than the target urine output but they are hypotensive. It is not clear what to do in this situation—many just increase fluids anyway. Others may provide vasopressors to increase blood pressure but there is the risk that vasoconstriction to the injured skin might increase burn depth. In addition, it is not uncommon for the patient’s hemoglobin and hematocrits to rise as an indicator of hemoconcentration. Using the hemoglobin or hematocrit to guide resuscitation tends to lead to the administration of excessive fluids since experience suggests that it takes time for hemodilution to occur. Another option is to measure lactate levels since higher levels are an indication of poor perfusion [24–27]. Again, it is not uncommon for the patient to have higher lactate levels while making adequate urine.
Because of these problems, many burn teams have used more advanced methods of detecting cardiac output and hemodynamics during burn shock. Using central venous pressures (CVP) is unreliable since they rarely rise above normal levels despite excessive urine output [28–31]. The use of pulmonary catheters has also been shown to over-resuscitate burn patients [30, 31]. More modern noninvasive devices have not been any better in guiding resuscitation [12, 16, 32]. In special cases, such as patients with renal or cardiac failure, these devices may be all that is available to guide resuscitation. Fortunately, most healthy patients can tolerate a wide range of inadequate or excessive volumes of fluid.
The major problem in the modern burn unit is from providing too much fluid, not under-resuscitating. There has been an increase in effort to understand why “fluid creep” has become a problem [1, 2, 13–15]. One problem is that the many endpoints do not align. If the patient is not making good urine output, the tendency is to increase volumes. It is clear, however, that many times over-resuscitation is the result of failing to respond to the patient’s response. It is not uncommon to observe a failure to reduce fluids despite excessive urine output. One solution is to utilize nurse-based protocols that provide guidelines for adjusting resuscitation fluids [2, 24]. This problem has also prompted investigators to develop computer-based devices that automatically adjust fluids based on urine flow [33–35]. Another theory is that the increased use of opioids has led to increased vasodilation and thus the need for more fluids [36]. This “opioid creep” phenomenon might be reduced by avoiding automatic narcotic drips and providing pain medications on an as needed basis. The other theory is that when a patient is placed on a ventilator the positive chest pressure reduces venous return [37]. More fluids are required in order to overcome the increased thoracic pressure. Clearly, there is still a great deal of work required to understand how to optimize fluids during burn shock.
15.6 Maintenance Fluid Rate
Evaporative Water Loss
<30 kg = (35 + % TBSA burn) × BSA = mL/h
>30 kg = (25 + % TBSA burn) × BSA = mL/h
We then consider burn shock to be completed when the resuscitative volume reaches the sum of basal requirements + evaporative water loss:
Maintenance Fluid Rate = Daily Basal Fluid Rate + Evaporative Water Losses
Basal Fluid Requirements = 100 mL/kg × 10 = 1000 mL for 24 h or 40 mL/h
Evaporative Fluid Rate = (35 + 50) × 0.5 = 42.5 mL/h
Maintenance Fluid Rate = 82.5 mL/h (1020 mL/day)
Therefore, when the fluid rate for burn resuscitation drops to the calculated maintenance rate, 82.5 mL/h, we consider the patient to be resuscitated and we switch the fluid to D5½NS with 20 mEq KCl. After the initial resuscitation, urine output becomes unreliable due to the resorption of resuscitative fluids and fluids provided in the operating room. In addition, the hypermetabolic changes often lead to spilling glucose or proteins so urine output tends to be high despite normovolemia. We leave the daily fluid target at the maintenance rate until the wound starts to close. Fine tuning of the rate is based on the blood urea nitrogen and creatinine.
15.7 The Choice of Fluid for Resuscitation
The classic teaching has always been to provide an isotonic crystalloid fluid—usually lactated Ringer’s (LR) solution during burn shock. The rationale for using an isotonic crystalloid was that during shock the gap created between the endothelial cells was so large that many proteins, including albumin, passed into the interstitial space. The leak of large proteins occurs not only at the burn site but also away from the burn [39–42]. There is no sense to provide colloid if it were to leak into the interstitial space. Even worse, the extravasated proteins would increase interstitial oncotic pressure and tend to drive more fluid into the interstitium. This protein leakage does occur but capillary leak away from the burn tends to be less severe and tends to close within 6–12 h. In addition, serum albumin levels drop precipitously due to leakage and dilution. There must be a point where the plasma oncotic pressure becomes so low that more fluid leaks than is necessary. Because of this fact, many burn teams are utilizing oncotic fluids more commonly than in the past [19].
There are pros and cons behind using crystalloids versus colloids. Crystalloids have been favored for many years because they are effective most of the time. There is little risk of transmitting infectious organisms compared to human-derived products. They are significantly less expensive than colloids and much easier to store. Therefore they are readily available for the field situation. The main downside for crystalloids is that they are freely permeable from the intravascular space to the interstitial space. Since the interstitial space has 3 times the volume of the intravascular space, one must replace 3–4 times the volume of lost intravascular fluid. In other words, if a patient loses 1 L of blood, then, in theory, one must give 3–4 L of isotonic crystalloid to replace that blood loss. In addition, proteins create the osmotic intravascular pressure that keeps fluid in the intravascular space. As more crystalloid is delivered, the intravascular osmotic pressure gradually decreases to a point that even greater leakage of fluids out of the intravascular space. In theory, this extra interstitial fluid would predispose a patient with large fluid requirements to higher risks of extremity, thoracic and intra-abdominal compartment syndromes. In addition, one may be at higher risk for pulmonary and cardiac compromise. Fortunately, most healthy patients can tolerate the extra volume and will later excrete the extra fluid.
Colloids, in theory, should reduce the volume of fluid needed to resuscitate a patient who is in shock. Colloids should replace blood loss in a 1:1 ratio, so that only 1 L of colloid would be required to replace 1 L of blood loss. In addition, colloids increase the intravascular osmotic pressure to reduce fluid loss through the capillaries. The downside of colloids is that they are more expensive than crystalloids; and there is a slight risk for infectious transmission, at least for human products such as albumin or fresh frozen plasma. The other problem that has been described in the past was that many proteins, including albumin, leak across the capillaries during burn shock. The argument against their use is that since they freely leak out they are wasted and even harmful [39–42]. It is possible that increasing the osmotic pressure in the interstitial space will “draw” more fluids from the intravascular space. In other words, colloids are fine if there is no capillary leak, but if there is an ongoing capillary leak then they are potentially harmful.
After a major burn injury, there is leakage of albumin and other proteins at the burn site and, in addition, distant from the burn site. The capillary leak at the uninjured capillary sites is less severe and lasts 6–12 h. In addition, the albumin levels frequently drop to very low levels (<1 mg/dL) [43–45] so that after several hours there is very little intravascular osmotic pressure to prevent further fluid loss. Therefore, after 6–12 h the addition of exogenous albumin to reduce fluid administration makes sense. When burn directors around the world were surveyed, it was discovered that while most initiated resuscitation with crystalloid, most “cheated” at some time during the first 24 h by adding albumin or another colloid to the resuscitation [19]. During the resuscitation discussion at the 2016 Burn State of the Science meeting [12], most participants admitted to using either albumin or fresh frozen plasma at some time during burn resuscitation. The remaining major dilemma is whether colloids should be started immediately or hours later when crystalloid resuscitation was failing. Everyone agreed that a multicenter prospective trial testing the validity of crystalloid versus colloid is needed.
Several studies have compared the use of albumin versus crystalloids for all types of resuscitation. The use of albumin was tempered by the Cochrane Collaborative, which in 1998 stated that the use of albumin increased the risk of death [46]. In 2012 and 2013, however, the same group reviewed 24 trials and found no difference in mortality [47, 48]. Very large trials have been performed, but not in burn patients. The “Saline versus Albumin Fluid Evaluation (SAFE) Trial” found no increase in mortality in a study with nearly 7000 patients [49]. The “Early Albumin Resuscitation During Septic Shock (EARSS) Study” [50] and “Albumin Italian Outcome Sepsis (ALBIOS) Study” [51] also found no difference in mortality. In 2014, a meta-analysis of all three of the above studies suggested a small reduction in mortality with the use of albumin [52].
There are fewer studies with the use of albumin in burns, and the number of patients is much smaller than the above studies. There are two approaches to studying the use of albumin for burn resuscitation—“immediate use” or delivering albumin as a “rescue” when crystalloid resuscitation volumes exceed predictive values. There were two studies from the 1970s that compared albumin versus crystalloid starting at the initiation of burn resuscitation [53, 54]. Both revealed that the immediate use of albumin reduced the fluids required for resuscitation. The study by Goodwin, et al. found that albumin could lower fluid requirements during burn resuscitation but they found that albumin increased lung water compared to the use of lactated Ringer’s solution [55]. This study led to the philosophy that albumin usage during burn resuscitation had potential adverse consequences. In 2006, Cooper et al. performed a prospective trial comparing lactated Ringer’s solution versus lactated Ringer’s solution containing 5% albumin [56]. They found an insignificant reduction in fluids with the addition of albumin. The philosophy at the time was that there was no need to provide albumin at the start of burn resuscitation.
As stated earlier, albumin was being provided later during resuscitation as burn caregivers “cheated” by adding albumin to improve resuscitations that were providing excessive amounts of fluids [19]. At the same time, the concept of “fluid creep”—providing way too much fluid—was the topic of the burn community. The concept of providing albumin as a “rescue” to reduce fluid requirements in patients not meeting target resuscitation goals became popular. The concept is that around 6–12 h the capillary leak starts to close at the same time as the intravascular osmotic pressures drop to extremely low levels (albumin levels often <1 mg/dL). It makes sense that at 6–12 h, increasing the intravascular osmotic pressures with albumin would slow capillary leakage and reduce fluid requirements. The first study examining albumin for rescue, from Utah, utilized the protocol to add 5% albumin (1/3 rate) to 2/3 rate lactated Ringer’s solution if the patient was receiving volumes twice the Parkland formula (>4 mL/kg/% burn) [57]. This study demonstrated that adding albumin could reduce the amount of fluids used later in the course and it suggested a reduction in mortality. The United States military reviewed their protocol of adding 5% albumin if fluid rates were >6 mL/kg/% burn, and they added vasopressin if the patient was hypotensive [58]. They demonstrated a reduction in abdominal compartment syndrome (36% versus 18%, p = 0.0315). Another study suggested that albumin might reduce extremity compartment syndromes and renal failure [59]. Two more recent studies from Utah clearly demonstrated that the initiation of albumin had a dramatic effect changing the trajectory of resuscitation from greater than target back to reasonable levels [60, 61]. Finally, a report from Michigan suggested that use of albumin for “rescue” reduced the incidence of abdominal compartment syndrome, ventilator days, oxygenation, vasopressor use, and mortality [62]. The volumes, however, were not different in this retrospective trial. Two meta-analyses on the use of albumin on burn resuscitation have been recently published which reveal no differences in mortality, renal failure, and respiratory failure with the use of albumin [63, 64]. In addition, there was a reduction in fluid volumes and compartment syndromes. While the controversy over the use of albumin seems to be lessened, there is still a need for a larger prospective study to evaluate the role of albumin.
There are other colloids that are available but their use has fallen into disfavor. Hydroxyethyl starch (HES) solutions have been used for burn resuscitation since the 1980s [65, 66]. A prospective trial from England compared Hartmann’s solution (crystalloid) versus 1/3 of the predicted crystalloid was replaced with 6% HES. Those patients receiving HES required less fluid and had less interstitial edema [67]. In the same year, another study compared burn patients resuscitated with lactated Ringer’s solution to hyperoncotic 10% HES in 30 patients [68]. The fluids required with HES were less but not statistically significant. The major concern was the finding that the patients treated with HES had a higher mortality and a 25% incidence of renal failure [68]. In 2013, the same group performed another trial with 6% HES 48 burn patients [69]. They found no differences in the amount of fluid delivered, but mortality and renal failure were not different in this study [69]. The use of HES for resuscitation in any patient was greatly discouraged after two very large randomized prospective trials demonstrated a higher risk for renal failure and mortality [70, 71]. The use of gelatins or dextran solutions has not gained favor for any major resuscitation. There are some centers that use fresh frozen plasma for burn shock resuscitation. O’Mara et al. demonstrated that using fresh frozen plasma for resuscitation reduced total resuscitative volumes, weight gain, intra-abdominal pressures, and base deficit compared to lactated Ringer’s solution [72]. The main protein in fresh frozen plasma is albumin so one could argue that it is just another formulation of albumin.
15.8 High Dose Vitamin C and Other Methods to Reduce Inflammation
Ascorbic acid (vitamin C) has gained popularity in many burn centers as an inexpensive drug to reduce burn-related oxidative stress and thus reduce fluid requirements for burn resuscitation. The initial animal studies were performed by Matsuda and Tanaka who found that adding vitamin C to lactated Ringer’s solution would reduce the fluids required for burn resuscitation compared to lactated Ringer’s solution alone [73–75]. They next performed an unblinded prospective trial in 37 burn patients adding 66 mg/kg/h vitamin C to lactated Ringer’s solution. They found that the high dose vitamin C significantly reduced fluid volumes and improved oxygenation compared to lactated Ringer’s alone [76]. Kahn and Lentz found similar reductions in fluid requirements with no increase in complications [77, 78]. When discussing different vitamin C protocols at the Resuscitation Special Interest Group of the 2017 Annual Meeting of the American Burn Association, all centers admitted that vitamin C would induce paradoxical diuresis and that one must beware of hypovolemia [12]. Kahn and Lentz, for instance, stated they would provide fresh frozen plasma if there were signs of hypovolemia. There is a need to expand the studies of the use of high dose vitamin C to determine its efficacy and safety. There is the theoretic potential for renal failure and hypovolemic shock [79]. Hopefully, multicenter trials will lead to a definitive answer to the use of vitamin C.
Another method of reducing oxidative stress and other signals of inflammation is to remove the inciting agents from the blood. The burn team at Utah proposed the use of exchange transfusions for small children and plasmapheresis to “rescue” patients from excessively high volume resuscitations [80–82]. Their data suggest that both techniques reduce fluid requirements. More recently, the teams in Seattle [83] and Wake Forest [84] have supported these findings with 37 and 21 patients, respectively. More expansive studies are needed to corroborate the effectiveness of therapeutic plasma exchange in burn resuscitation.
15.9 Complications of Excessive Fluids
Providing inadequate volumes of resuscitation leads to the complications of hypovolemic shock such as renal failure, increased burn depth, and ultimately death. To compensate for hypovolemia, catecholamines are released which in turn lead to vasoconstriction to peripheral capillary beds, especially the skin and extremities. Typically, the burn is divided into three zones: zone of coagulation where the skin is destroyed, zone of stasis where capillary blood flow is decreased due to leukocyte crowding, and the zone of erythema due to vasodilation. With inadequate resuscitation capillary flow is further decreased in the zone of stasis, which increases the chance of burn depth progression. Therefore, providing adequate fluid resuscitation during burn shock is essential to optimizing an optimal outcome.
Clearly, not providing enough fluid leads to adverse outcomes but this knowledge has led to tendency to provide excessive amounts of fluid, described as “fluid creep” [1, 2, 13–15]. It is clear, however, that providing too much fluid has its own set of complications including extremity compartment syndromes, and intrathoracic and intra-abdominal compartment syndromes. There has also been a description of blindness from increased intraocular pressure [85]. In addition, excessive fluid can enter the lungs to exacerbate the risks for acute respiratory distress syndrome or worsen smoke inhalation injury. Too much fluid can overwhelm the heart and lead to congestive heart failure. Excessive edema leads to a greater distance for nutrients to travel from the capillaries to the wound, so it has been associated with impaired wound healing. While these complications may occur with appropriate burn resuscitation, they are clearly increased with excessive over-resuscitation.
Extremity compartment syndromes are caused by pressures within a closed muscle compartment that lead to impaired capillary flow to the enclosed muscle. There are two factors that lead to compartment syndromes in burned extremities: significant capillary leakage within an enclosed muscle compartment and a deep circumferential burn that will not expand. In other words, small superficial and circumferential burns that are relatively small do not lead to compartment syndromes. Deep, full-thickness and circumferential burns do not expand and are at risk for compartment syndromes. The classic symptom of compartment syndromes is pain that is caused by ischemia to the muscle. The pain is worsened with passive motion. Unfortunately, many patients have so much pain due to the burn itself that compartment syndromes are missed. Therefore, one should always be cognizant that compartment syndromes may occur in patients with deep circumferential burns.
The diagnosis of extremity compartment syndrome may not always be simple. The risks are greater for the problem if the patient has larger and deeper circumferential burns. Some caregivers feel there is no compartment syndrome if the extremity has a distal pulse. Unfortunately, compartment damage may occur in the presence of distal pulses. In order to lose the distal pulse, the compartment pressure must be higher than systolic blood pressure. It requires compartment pressures of around 30 mmHg to lose capillary flow to the muscle, so damage often occurs despite having distal pulses [86]. If there is a question of compartment tightness, one may easily measure pressures by placing an 18 gauge needle on monitor (such as central venous pressure monitor) and inserting it into the compartment. If the pressure is >30 mmHg, then escharotomies are usually indicated. If the patient has very large and deep burns, I will often perform escharotomies early in the course of the initial resuscitation. Failure to perform escharotomies when indicated may lead to muscle necrosis and potentially, amputations. As a worst case scenario, I had one patient with 80% TBSA burns sent to us 4 days after injury who required bilateral above knee amputations because most of the extremity muscle had died. He had never had escharotomies. There is very little downside to performing escharotomies since those same wounds will ultimately require grafting.
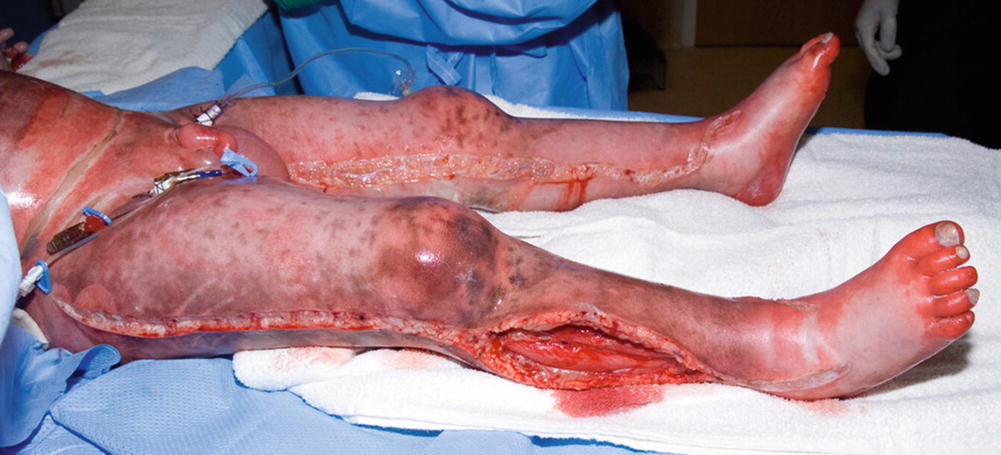
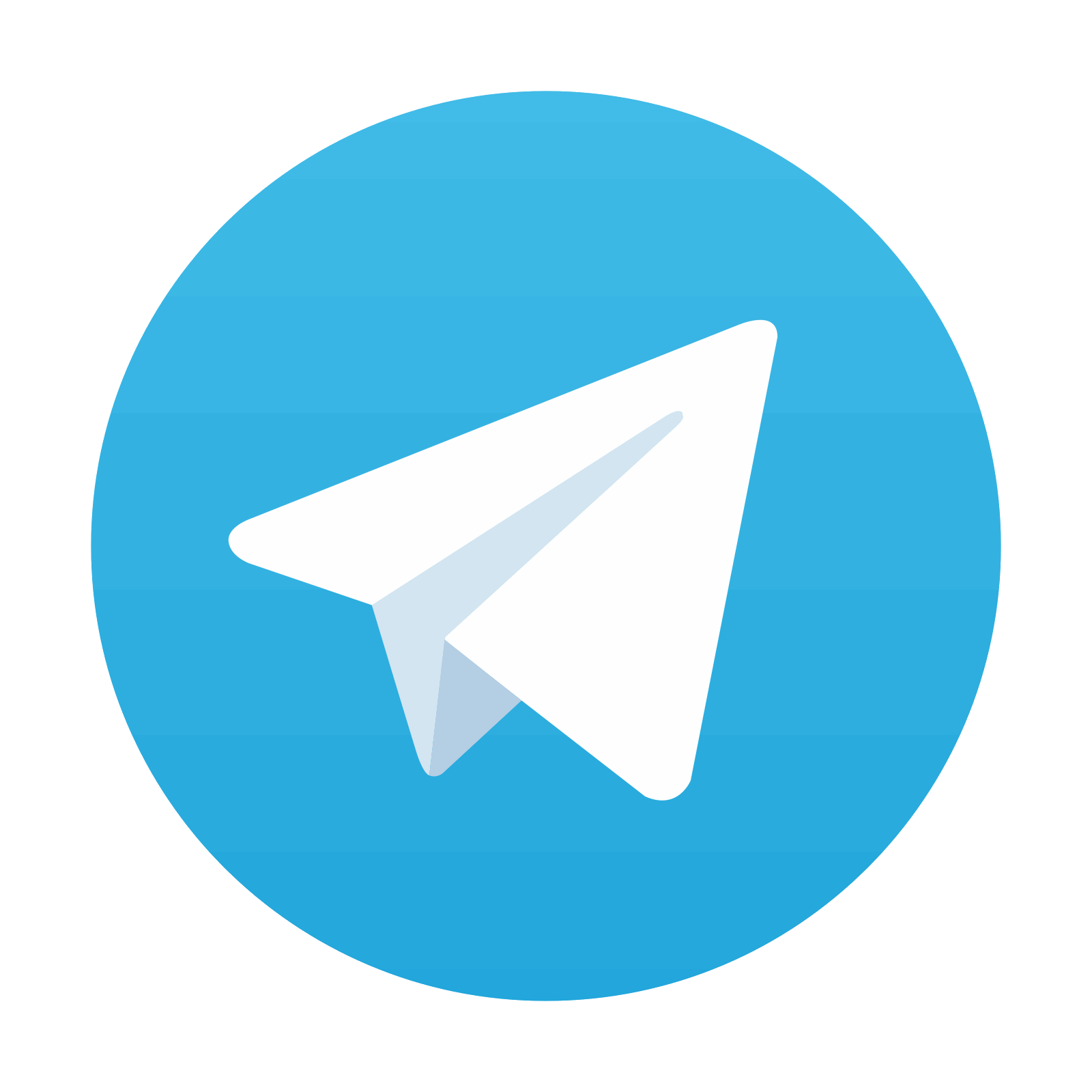
Stay updated, free articles. Join our Telegram channel

Full access? Get Clinical Tree
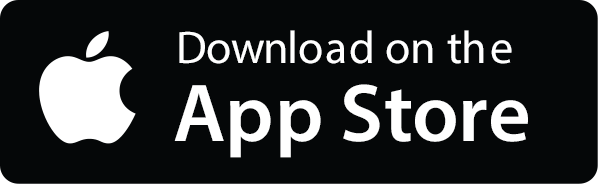
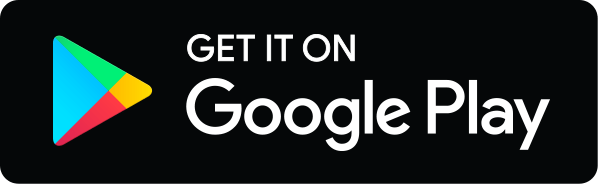