!DOCTYPE html>
17. Respiratory Management in Burn Care
17.1 Introduction
Airway and respiratory management are necessary and important components of burn care because a significant minority of burn patients requires intubation and mechanical ventilation, often for weeks or even months. Burn patients require respiratory management for two major reasons: the presence of smoke inhalation injury (II) and/or a large percent total body surface area (% TBSA) burn. Inhalation of the products of combustion causes a cascade of injury and inflammatory response that typically leads to respiratory failure and the need for intubation and ventilation. Likewise, a large % TBSA thermal injury (typically ≥30% TBSA) imposes such a large systemic metabolic load on patients that intubation and ventilation are almost always necessary. This chapter reviews general concepts of airway and respiratory management in burn patients.
17.2 Airway Management
17.2.1 Endotracheal intubation
As noted previously, the two major indications for endotracheal intubation in burn patients are inhalation injury and a large % TBSA burn. Each of these indications has several individual components.
Inhalation injury (II) is classically caused by breathing in the hot products of combustion. The smoke produced by such combustion typically has hundreds of toxic chemicals. These chemicals react with airway and alveolar cells producing injury and initiating an intense inflammatory response. This injury and inflammatory response ultimately causes airway obstruction, impaired ciliary function and surfactant production, thickening of the alveolar-capillary membrane, ventilation-perfusion mismatch, hypoxemia, hypercarbia, acidosis, and respiratory failure [1–5]. II can be classified into injury above larynx which is caused primarily by heat, and injury below the larynx which is typically caused by chemical exposure. Patients with upper airway (heat) injury often require intubation because the local edema and tissue swelling from the burn injury may cause upper airway obstruction. Patients with lower airway and lung injury (chemical) often require intubation because of respiratory failure. Not infrequently patients will have both upper airways and lower airway and lung injury simultaneously [6].
The suspicion of an II (either upper or lower) often is enough of an indication for intubation. However, not all patients exposed to smoke will require intubation. It can be difficult to determine early in a patient’s clinical course if that patient will develop significant upper airway edema to cause obstruction and/or enough lower airway and lung injury to cause respiratory failure. Close observation with preparation to intervene immediately is a prudent course. If the patient is to be transported to another facility and obtain a definitive airway during transport is likely to be difficult, then prophylactic intubation is warranted. Ultimately, if the course is uncertain, then intubation is the wisest choice. If the intubation proves unnecessary, then the endotracheal tube can be removed. Conversely, if the patient is not intubated and subsequently requires intubation, the procedure may be very difficult because of upper airway edema and obscuring of the airway and landmarks.
Thus, patients with evidence of upper airway injury upon presentation or developing during observation, such as obvious orinasal burns, swelling, erythema, stridor, or hoarseness should be promptly intubated. Likewise, patients with evidence of lower airway and lung injury such as a history of closed space injury, the presence of true carbonaceous sputum either coughed up or suctioned, an elevated carboxyhemoglobin level >10% (see below), stridor, or hoarseness should also be promptly intubated.
A third component of II is carbon monoxide (CO) poisoning . CO is produced by virtually every type of fire. CO preferentially binds to hemoglobin over oxygen and causes hypoxemia. The degree of CO poisoning is determined by the degree of binding to hemoglobin to produce carboxyhemoglobin (COHg). This is measured as a percent of hemoglobin bound to CO. Most patients have baseline levels of COHg close to 0%. Mild to moderate symptoms occur with levels of 20–30%, severe symptoms with levels of 30–50%, and death with levels >50%. Documentation or suspicion of CO poisoning (levels >10%) mandates prompt intubation and placing the patient on 100% FiO2. The half-life of COHg can be reduced from 4 h on room air to less than 60 min with 100% oxygen [7].
Finally, there are rare instances where the lower airway and lung suffer direct thermal injury. Examples of this are inhalation of hot steam or inhalation of hot or flaming liquids such as flaming fuel. These situations almost always warrant immediate intubation.
The second major indication for early intubation in burn patients is the presence of a large %TBSA burn injury. The generalized inflammatory response to burn injury and the resulting soft tissue edema from that response plus the large fluid volume resuscitation often leads to airway edema even in the absence of an II or direct burns to the face and head. The metabolic load from the large thermal injury adds additional stress. Finally, the high levels of analgesia and sedation usually required to maintain adequate pain control and comfort in a burn patient often could not be administered safely in the absence of intubation and ventilation. There is no mandatory cut-off for % TBSA above which intubation is necessary. However, 30% TBSA seems a reasonable level at which to consider intubation and is the cut-off recommended by Acute Burn Life Support.
Another consideration in addition to the size of the burn is their anatomic location. Upper body burns (head and neck, torso above the nipples, upper extremities) may cause airway edema even if the airway and surrounding structures are not directly involved. This potentially dangerous situation should be recognized early and intubation performed before airway edema makes it difficult.
Finally, a last indication for intubation is a decreased level of consciousness (DLOC). This may occur with or without II and/or a large %TBSA burn. There are numerous reasons for this DLOC including hypoxia, hypercarbia, medications, substance abuse, associated traumatic injury, II, CO poisoning, etc. These patients should be immediately intubated and a thorough investigation into the cause of the decreased level of consciousness initiated.
Once the decision has been made to intubate, the entire team should be assembled and the most experienced person should intubate. Equipment should include a functioning bag and mask with 100% FiO2, suctioning device, ventilator, laryngoscope, and appropriate medications. Adjuncts to facilitate visualization of the vocal cords and airway include a fiberoptic bronchoscope and glideslope. The team should be prepared to perform an emergency cricothyroidotomy or tracheostomy if standard endotracheal intubation fails.
17.2.2 Tracheostomy
There are several controversies surrounding the use of elective tracheostomy in burn patients requiring prolong ventilation. The first controversy is whether tracheostomy is indicated (“to trach or not to trach?”). If one believes that tracheostomy is in fact indicated, the next issue is the timing of the tracheostomy (1 week post-injury? 2 weeks? 3 weeks?). Once one has decided when to perform a tracheostomy, the next issue is how to perform. The general choices are traditional, operative, open tracheostomy versus bedside percutaneous dilational tracheostomy, or some combination of the two. A final question is whether a neck burn impacts any of the other three issues presented. Let’s look at each of these in greater detail.
The advantages of tracheostomy include easier suctioning, better maintenance of oral hygiene, better ventilator mechanics, more secure, better tolerated requiring less sedation, and easier weaning from ventilation [8]. Disadvantages include necessity for an invasive procedure, easier colonization of respiratory tract, and possible development of a tracheoinnominate fistula. Studies on the effectiveness and safety of tracheostomy prior to the mid-1990s were mostly retrospective and did not provide good data. Early studies tended to report high complication rates and poorer outcomes with tracheostomy [9]. More recent studies report low complication rates and better outcomes [10].
Saffle et al. [11] randomized burn patients to early tracheostomy (4 days post-injury) or conventional treatment with ventilation via standard endotracheal tube until day 14 at which time the patients underwent tracheostomy. There were no differences in the two groups in length of stay, need for ventilator support, incidence of pneumonia, or survival. The early tracheostomy group demonstrated improvement in PAO2/FiO2 ratio within 24 h of the procedure. However, there was a significantly great number of patients in the conventional treatment group who were extubated compared to the early tracheostomy group. The authors concluded that early tracheostomy offered no particular advantage or disadvantage over conventional management.
Palmieri et al. [12] reported a retrospective review of 38 severely burn children who underwent tracheostomy at a mean time of 3.9 days after admission. Peak inspiratory pressure, PaO2/FiO2 ratio, pulmonary compliance, and ventilatory volumes all improved following tracheostomy. There were no tracheostomy-related deaths, no tracheostomy site infections, and no post-tracheostomy tracheal stenosis. The authors concluded that early tracheostomy in this population of burn patients was safe and effective, and that it resulted in a more secure airway and improvement in ventilator management.
Aggarwal et al. [13] reported a retrospective comparison of burn patients who received tracheostomy (n = 48) with burn patients who had ventilation with endotracheal intubation only (n = 84). The tracheostomy group had significantly larger % TBSA burns, a significantly greater incidence of inhalation injury, and a lower probability of survival based on the Abbreviated Burn Severity Index (ABSI). Duration of ventilation, length of hospital and ICU stay, and incidence of pulmonary infection were all greater in the tracheostomy group, but mortality was equivalent between the two groups.
The second controversy regarding tracheostomy is the timing of tracheostomy. Reports of tracheostomy performed as early as 3 [14] or 4 [11, 12] days after the initiation of mechanical ventilation have demonstrated safety and efficacy for the procedure and timing. A recent survey of ventilator practices showed that most (67%) of respondents considered tracheostomy after 2 weeks of mechanical ventilation. Reasons for earlier tracheostomy included large % TBSA burn, burns of the head and neck, failed extubation attempts and poor fluid resuscitation status. Additional considerations for early tracheostomy were severe traumatic brain injury and predicted prolonged need for mechanical ventilation [15].
The third controversy regarding tracheostomy is the choice of technique, either a traditional open tracheostomy or a bedside percutaneous dilational tracheostomy. The traditional method is performed in the operating room under general anesthesia. The neck is dissected through an anterior incision, the trachea is exposed, and the tracheostomy is place under direct vision. The percutaneous method is performed at the bedside usually under IV sedation with or without monitored anesthesia care. A needle is percutaneously placed into the trachea under bronchoscopic visualization. A guideline is placed through the needle, a dilator is used to dilate the track and the tracheostomy site, and a tracheostomy tube is placed. In the survey of ventilation practices, 78% of burn surgeons preferred to perform tracheostomy in the operating room [15]. Comparative trials of the two techniques have generally demonstrated either no difference in outcomes, or fewer complications and lower cost with the percutaneous technique [16–19].
The final issue is that of tracheostomy use in a patient with a neck burn. This remains controversial and the evidence for one clinical path over another is lacking [8]. As noted above, head and neck burns may be an indication for tracheostomy or even early tracheostomy. Smailes et al. studied this and found a higher incidence of stoma infections in grafted necks if the autograft was not fully adhered and healed to the neck. They recommended performing tracheostomy only after the neck autograft was completely healed, and suggested 10 days post-graft [19].
In conclusion, tracheostomy appears to be a useful tool in burn care, and the advantages largely outweigh its disadvantages. The most common timing for tracheostomy is approximately 2 weeks after the onset of ventilation although early tracheostomy (3–4 days) may be beneficial in some situations. Open tracheostomy is the preferred method of tracheostomy. However, percutaneous tracheostomy is safe and may offer some advantages over open. Patients with neck burns can safely undergo tracheostomy, but the procedure is best performed after excision and autografting.
17.3 Ventilator Management
Burn patients with injuries ≥30% TBSA or burn patients with inhalation injury typically require mechanical ventilation, often for weeks or even months. Burn patients are somewhat unique in the fact that they have normal lungs and normal lung function, at least at the time of initiation of mechanical ventilation. Obviously, patients with inhalation injury have lung pathology, and the inflammatory response of burn injury may induce some pathophysiologic changes in otherwise normal lungs. This makes evaluation of ventilator studies somewhat difficult because most of these studies have been done in patients with lung pathology such as acute lung injury (ALI), acute respiratory distress syndrome (ARDS), pneumonia, and others. Compounding this problem is the fact that burn patients have not infrequently been excluded from ventilator studies. Let’s now examine the various ventilator management strategies used in burn patients. We will start with the two most common ventilation management strategies for patients with ARDS: lung protective ventilation and the open-lung approach. We will conclude with several less common ventilation strategies.
17.3.1 Lung Protective Mechanical Ventilation
The Acute Respiratory Distress Syndrome Network (ARDS Net) trial in 2000 has had a profound effect on ventilator management. The trial compared ventilation with lower tidal volumes (6 mL/kg) to ventilation with traditional higher volumes (12 mL/kg). The lower tidal volume group demonstrated significantly lower mortality and greater number of ventilator-free days. Mean plateau pressures were also significantly lower in the lower tidal volume group. Subsequent studies have confirmed the safety and efficacy of lower tidal volume ventilation. It has become known as lung protective ventilation [20].
Lung protective ventilation (LPV) is appropriate and indicated for burn patients with and without inhalation injury. Initial ventilator setting goals in these patients are a tidal volume of 6–8 mL/kg with respiratory rate and positive end-expiratory pressure (PEEP) to attain normal arterial blood gas values for PAO2, PACO2, and pH, and with peak inspiratory pressures (PIP) less than 35 cm H2O. Numerous studies have validated the effectiveness of lung protective ventilation in patients with ARDS, ALI, and other pulmonary disorders requiring ventilation management [21–25].
Lung protective ventilation may present challenges in burn patients because of unique features of burn pathophysiology. Burn injury and fluid resuscitation results in soft tissue swelling and edema. Body wall and torso edema may be significant enough to decrease chest wall compliance. Under these circumstances, it may be necessary to increase tidal volume to compensate for decreased compliance. Likewise, a thermal burn extending over most or all of the torso may adversely decrease chest wall compliance. This is particularly true for circumferential and/or full-thickness burns.
17.3.2 The Open-Lung Approach to Mechanical Ventilation and Positive End-Expiratory Pressure (PEEP)
The protective lung strategy aims to reduce lung strain by decreasing lung volumes and pressures. The second strategy, the open-lung approach (OLA) , aims to decrease dynamic strain on alveoli. The purported advantages of OLA include improved oxygenation and ventilation, decreased atelectasis, and decreased shear injury from opening and closing of alveoli [26]. The hallmarks interventions of the OLA are: (1) to open alveoli with recruitment maneuvers, and then (2) keep them open with PEEP [27].
Recruitment maneuvers (RMs) comprise a variety of techniques used to temporarily increase intrapulmonary pressures and expand airless and atelectatic alveoli. The overall goal of RMs is to increase the number of open alveoli, improve lung aeration, increase the surface for gas exchange, and ultimately improve oxygenation. One key to successful recruitment is to use RMs that effectively open collapsed alveoli but do not over-distend and injure the alveoli. Indeed, one objection to RMs is that if they are improperly implemented, adverse effects such as hypotension, barotrauma , or oxygen desaturation can occur. There are several RMs that have been demonstrated to be effective including sigh breaths, sustained inflation, assisted ventilation, prone positioning, and stepwise increase in airway pressure and/or PEEP. The ideal RM protocol (type, frequency, duration, peak pressure, etc.) is not known and patient response to an RM is impossible to predict. Thus, RMs must be individualized. There is ample clinical data to support the concept that stepwise RMs tend to better improve oxygenation with less adverse hemodynamic or respiratory consequences than traditional abrupt RMs. Once a successful PM has been performed, the second hallmark of OLA ventilation is to maintain alveolar patency with PEEP [28–30].
Use a relatively high PEEP: 15 to 20 cm H2O
Use ARDSNet PEEP-FiO2 escalation tables (PEEP set based on oxygenation)
Titrate PEEP to maximum static compliance
Set PEEP to slightly above the lower inflection point of pressure-volume curve
Use stepwise recruitment to find lowest PEEP with maximal oxygenation
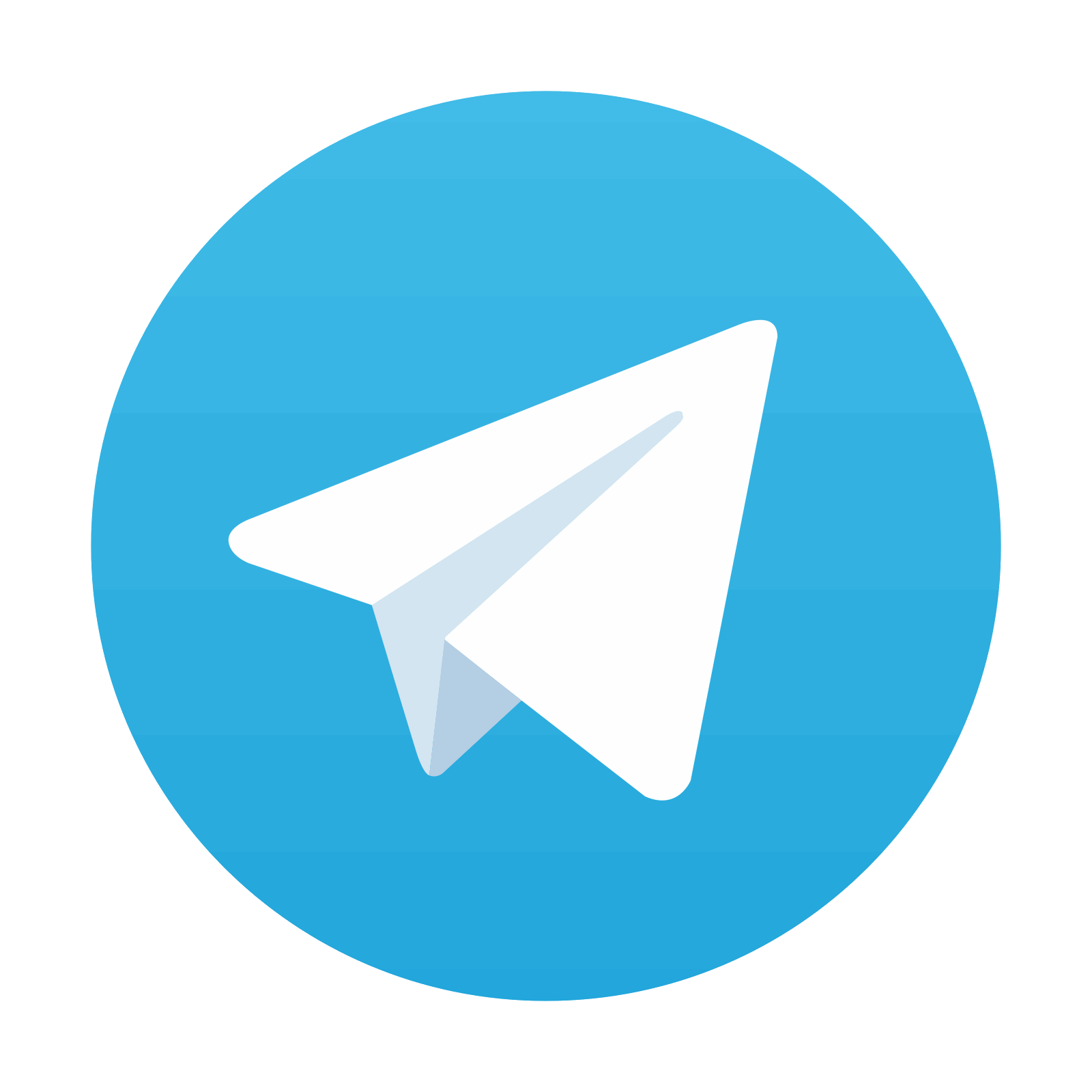
Stay updated, free articles. Join our Telegram channel

Full access? Get Clinical Tree
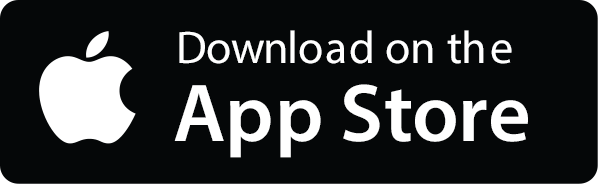
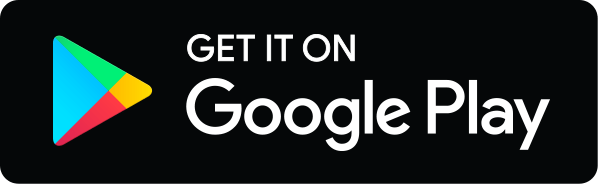