Lasers in Plastic Surgery
David W. Low
Ivona Percec
INTRODUCTION
Plastic surgeons recognized the potential benefit of treating vascular lesions with lasers in the 1980s, but it was not until the widespread popularity of laser skin resurfacing in the following decade that most plastic surgeons jumped on laser surgery bandwagon. Taking advantage of the public’s fascination with high-technology, laser therapy has been partially misrepresented as the “state-of-the-art” treatment for a variety of conditions. Often described as painless, and exaggerated as producing perfect results, lasers have been misused as a marketing tool to lure patients away from conventional, low-tech techniques that can often produce equivalent results at significantly lower cost. On the other hand, there are some conditions such as port wine stains that are best treated by laser, and the standard of care demands familiarity with this treatment modality.
The modern plastic surgeon is therefore faced with the dilemma of trying to sort out which lasers are best for which conditions, which manufacturers’ claims are credible, and, ultimately, which lasers are the safest investment in a rapidly changing world of high-tech solutions to a variety of reconstructive and cosmetic problems.
This chapter provides a basic introduction to laser technology, laser tissue interactions, and examples of what conditions are appropriate for laser treatment with currently available laser technology. Laser safety is an important consideration for both the patient and the treating physician, and safety issues are discussed at the end of the chapter.
LASER PHYSICS
How Laser Light Is Produced
Criminal masterminds Auric Goldfinger and Dr. Evil were fascinated by amplified light, “a sophisticated heat beam which we called a ‘laser,’” but fortunately in the 21st century, most lasers are used instead for therapeutic purposes. Although the vast array of available lasers can be confusing, laser physics are straightforward, and only a basic understanding of light energy is necessary to understand how lasers work. Light energy can be described as either a series of particles (photons) or as a wave phenomenon. The color of light is determined by the distance between two successive waves (the wavelength, usually measured in nanometers). The human eye can see only a narrow range of the electromagnetic spectrum (visible light), and many lasers produce invisible light in the infrared range.
A molecule or atom in its resting state is composed of a nucleus and circulating electrons. If energy is added to the system, the electrons become excited and circulate at a higher orbit. Eventually, an excited electron will fall back to its resting orbit, releasing a specific packet of energy–a photon. That photon has a wavelength specific to that molecule. Some molecules have more than one excited orbit, and therefore, the light emitted may have more than one wavelength. If a photon collides with an excited electron, that electron falls back to its resting orbit, thereby releasing another photon. The two photons are in phase, meaning their wave patterns are synchronized and therefore reinforce each other. As these photons hit other excited electrons, more photons are released and the light energy increases (Figure 18.1).
A laser tube has a mirror at each end and contains a solid, liquid, or gas medium within it whose electrons are in a resting state. As energy is added to the system, the majority of the electrons become excited (population inversion) and begin to release photons. Only those photons that hit the mirrors directly are reflected back into the lasing medium, creating more and more photons that travel back and forth between the mirrors, parallel to the tube. Since the photons are in phase, the intensity of the light increases in the tube. This phenomenon has been described as light amplification by the stimulated emission of radiation, or the more familiar term LASER. (In contrast, TASER is an acronym for Thomas A. Swift’s Electric Rifle and has nothing to do with light energy.) To allow light to escape from the tube, one of the mirrors is only partially reflecting. The emitted light is coherent; it is in phase, parallel, and in most cases monochromatic. In contrast, incandescent light is noncoherent, meaning it has many wavelengths and is not parallel.
Light energy can be visible or invisible depending upon its wavelength. The spectrum of electromagnetic radiation ranges from long radio waves (wavelength > 10 cm) to extremely short gamma rays (<10-11 m). The entire spectrum includes radio, microwaves, infrared, visible (400 to 700 nm), ultraviolet, X-ray, and gamma rays.
Types of Lasers
The laser tube may contain either a gas, liquid, or solid lasing medium (Table 18.1), and new lasers are constantly being invented and promoted to the medical community. The first laser, invented in 1960, used a synthetic ruby rod, and other solid crystal lasers include yttrium aluminum garnet (YAG). The YAG crystal contains neodymium, erbium, or holmium ions, each with its own specific wavelength and tissue interactions. In a dye laser, the medium is a solution of a fluorescent dye in a solvent such as methanol. Organic rhodamine dye is used in the yellow dye laser, and although the earlier dye lasers had adjustable (tunable) wavelengths ranging from yellow to red, currently available dye lasers offer single wavelength light energy. In a helium-neon laser, it is a mixture of the gases helium and neon. In a diode laser, it is a thin layer of semiconductor material sandwiched between other semiconductor layers. Excimer lasers (the name is derived from the terms excited and dimers) use reactive gases, such as chlorine and fluorine, mixed with inert gases such as argon, krypton, and xenon. When electrically stimulated, a pseudomolecule (dimer) produces light in the ultraviolet range.
TABLE 18.1 LASERS WITH PLASTIC SURGERY APPLICATIONS | |||||||||||||||||||||||||||||||||||||||||||||||||||||||||||||||||||||||||||||||||||||||||||
---|---|---|---|---|---|---|---|---|---|---|---|---|---|---|---|---|---|---|---|---|---|---|---|---|---|---|---|---|---|---|---|---|---|---|---|---|---|---|---|---|---|---|---|---|---|---|---|---|---|---|---|---|---|---|---|---|---|---|---|---|---|---|---|---|---|---|---|---|---|---|---|---|---|---|---|---|---|---|---|---|---|---|---|---|---|---|---|---|---|---|---|
|
Laser Tissue Interactions
When the laser strikes an object, a variety of desirable and undesirable effects may result as the light is reflected, scattered, transmitted, or absorbed. A series of reflecting mirrors directs CO2 laser light to the handpiece, but reflected CO2 light off of a shiny surgical instrument is hazardous. The risk of inadvertent light reflection can be reduced by using ebonized instruments. The dull finish scatters laser light, diffusing the concentrated energy beam. Glass and clear liquids will transmit some types of laser light, allowing photocoagulation through glass slides, the vitreous of the eye, and water. Some lasers will also pass through the epidermis, allowing the energy to reach dermal vessels and pigment without disrupting the epidermal layer. It is the absorbed light that causes desirable or undesirable biologic effects. Except for the excimer lasers that break chemical bonds, most laser energy is converted into thermal (heat) energy. Depending upon the rate of tissue heating, surgical effects include welding, coagulation, protein denaturation, dessication, and vaporization. Some lasers will indiscriminantly target living tissue, while other lasers will semiselectively target a specific chromophore such as oxyhemoglobin, melanin, and tattoo pigmentation. Selective photothermolysis describes the ability of lasers to target blood vessels or pigment without harming the surrounding epidermis or dermis. It is generally safer to deliver cutaneous laser light in pulses rather than as a continuous beam, as the interval between pulses allows the tissue to cool before the heat is transferred to the surrounding dermis. Pulsed lasers respect the thermal relaxation time of dermal vessels (the time to dissipate the heat absorbed during a laser pulse).
Ablative Lasers.
Lasers that nonspecifically destroy the tissue can be used to remove skin lesions or remove layers of skin, usually with minimal blood loss because the dermal vessels are coagulated as the tissue is vaporized. CO2 laser light is absorbed by intracellular water, which vaporizes the tissue as the water turns to steam.
Vascular Lesion Lasers.
The fact that oxyhemoglobin absorbs green and yellow light has spawned a variety of lasers appropriate for treating dermal vessels. Historically, the argon (blue/green) laser was the first clinically useful laser, but yellow light has become the preferred color (oxyhemoglobin absorption peak at 577 nm yellow light), with the pulsed yellow dye laser (intentionally adjusted to 585 and 595 nm for greater dermal penetration) as the most popular type. The high-energy/short-duration pulse causes vascular disruption as the blood rapidly heats up and expands. The potassium titanyl phosphate (KTP) laser (532 nm–green light) also targets oxyhemoglobin, but the pulses are much longer in duration and tend to coagulate rather than disrupt vessels. The diode laser (800 nm) can also be used for vascular lesions, as the light is absorbed by both oxyhemoglobin and melanin.
Pigmented Lesion Lasers.
Pigmented lesion lasers target melanin. Benign pigmented lesions such as lentigines, café au lait spots, melasma, and Nevus of Ota or Ito may improve with a series of laser treatments. Although congenital nevi will also lighten with laser therapy, this remains controversial; although it is unlikely that laser will increase the risk of malignant transformation, it may delay the diagnosis of a changing nevus by masking the color change associated with a melanoma.
Photodynamic Therapy.
The use of light-activated drugs to treat acne and other skin conditions currently is best represented by Levulan (topical 5-aminolevulinic acid, DUSA pharmaceuticals). The compound is metabolized by sebaceous glands into porphyrins. The acne bacteria itself also produces porphyrin, and the use of blue, green, or red light stimulates the production of oxygen free radicals that destroy the bacteria and suppress the sebaceous gland activity. Photodynamic therapy has also been used for actinic keratoses, non-melanotic skin cancer, T-cell lymphoma, and photorejuvenation.1
Nonlaser Phototherapy.
Intense pulsed light (IPL) is not actually laser light. Xenon flashlamps generate multi-wavelength noncoherent light that is partially modulated
by a series of filters. IPL is used for sun-related pigmentary changes, telangiectasias, and hair removal. In radiofrequency treatment, radio waves are used to heat the collagen of the dermis and subdermis. It is thought to cause collagen contraction and stimulation of new collagen production. It has been promoted as a noninvasive, nonablative treatment for skin laxity in many areas of the body, including the face. Although some publicized results are impressive, the ability to consistently achieve such results is far less predictable than surgical skin tightening. Proper patient selection remains a clinical challenge.
by a series of filters. IPL is used for sun-related pigmentary changes, telangiectasias, and hair removal. In radiofrequency treatment, radio waves are used to heat the collagen of the dermis and subdermis. It is thought to cause collagen contraction and stimulation of new collagen production. It has been promoted as a noninvasive, nonablative treatment for skin laxity in many areas of the body, including the face. Although some publicized results are impressive, the ability to consistently achieve such results is far less predictable than surgical skin tightening. Proper patient selection remains a clinical challenge.
SPECIFIC LASER TREATMENTS (TABLE 18.2)
Vascular Lesions
Hemangiomas.
Hemangiomas are the most common benign tumor of infancy, and at least 60% occur in the head and neck region. Although an estimated 70% of hemangiomas regress satisfactorily, 30% of patients still have cosmetically significant deformities. Parents are eager to seek treatment options on a proactive basis, and the laser is a potentially useful option in several settings.2 The pulsed yellow dye laser may be useful for very early hemangiomas, ulcerated hemangiomas, and regressed hemangiomas that still contain vascular pigmentation or visible ectatic vessels. The laser only penetrates about a millimeter into the skin, and therefore, it is most effective for small flat hemangiomas. Parents should be advised that multiple laser treatments may be necessary every 2 to 4 weeks during the proliferative phase, as hemangiomas will often exhibit temporary regression followed by rebound growth. Laser therapy can be discontinued when the hemangioma finally enters a permanent state of regression. Topical application of anesthetic cream may be desirable to reduce both the patient and the parent discomfort. Laser treatments are not effective for already bulky or subcutaneous hemangiomas, as the light will not penetrate deeply enough to produce a noticeable improvement.
TABLE 18.2 CLINICALLY USEFUL LASERS AND OTHER PHOTOTHERAPY DEVICES | ||||||||||||||||||||||||||||||||||||||
---|---|---|---|---|---|---|---|---|---|---|---|---|---|---|---|---|---|---|---|---|---|---|---|---|---|---|---|---|---|---|---|---|---|---|---|---|---|---|
|
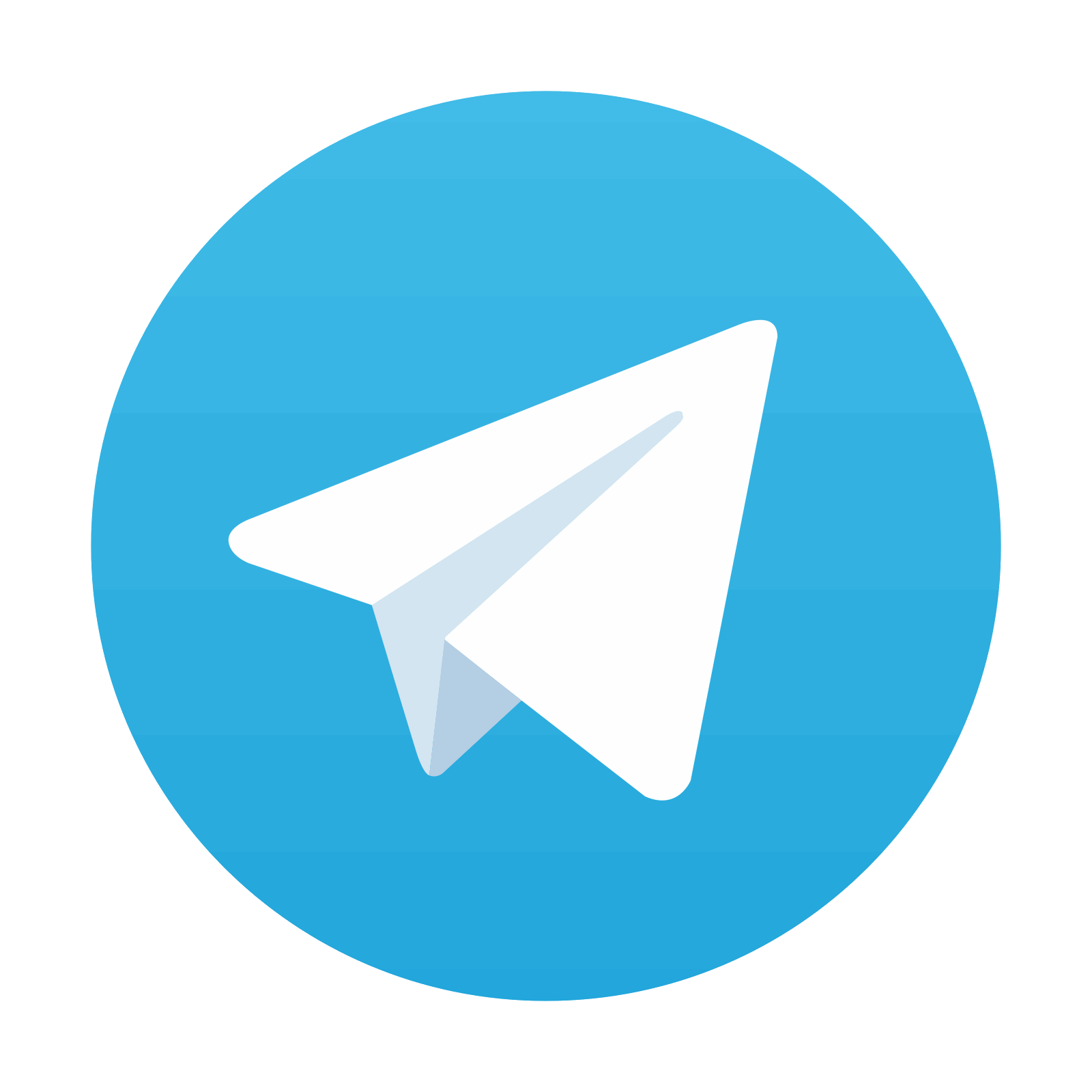
Stay updated, free articles. Join our Telegram channel

Full access? Get Clinical Tree
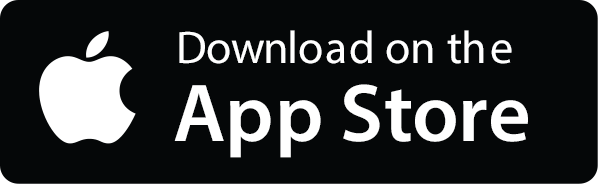
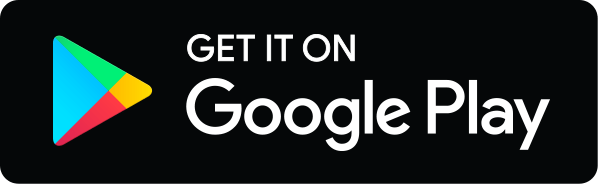
