Fig. 4.1
Schematic representation of iontophoresis. D is the drug, which can be either positively (D+) or negatively (D−) charged. N represents a neutral molecule. EM is the electromigration and EO the electroosmosis
4.2 Chronology: From First Reports to Recent Discoveries
The use of electricity in therapy dates back to the ancient Greeks who used electrical shocks from torpedo rays (Banga and Chien 1988). References to the use of a galvanic current for drug delivery date back to the mid-eighteenth century, while in the nineteenth century, there were numerous attempts to deliver metal ions and alkaloids into the skin (Helmstadter 2001). One of the first reports proving that an electric current could drive molecules across the skin came from the French physician Stéphane Leduc in 1900. In a classic series of experiments in rabbits, he demonstrated that cationic strychnine (present as a solution of strychnine sulfate) was transported from the anode, whereas negatively charged cyanide ions (present as a solution of potassium cyanide) were transported from the cathode (Banga and Chien 1988).
Since then, the electrotransport of numerous molecules with diverse clinical applications has been investigated (Kalia et al. 2004). Recent examples include topical delivery of cosmeceuticals and therapeutic agents for dermatological conditions (e.g., sodium ascorbyl phosphate, sodium dexamethasone phosphate, or valaciclovir) and the administration of molecules with systemic effect (e.g., granisetron or ranitidine) (Abla et al. 2006; Marra et al. 2008; Taveira et al. 2009; Cazares-Delgadillo et al. 2010a, b; Dubey et al. 2011; Djabri et al. 2012; Gratieri et al. 2012). In addition to the generally recognized advantages of the patient-friendly transdermal route, iontophoresis enables an almost unique level of control over drug input kinetics. Drug concentrations in the plasma are determined by the intensity and duration of the applied current profile and can be easily modified; the additional driving force (i.e., the potential gradient) also enables therapeutic drug concentrations to be reached more quickly and hence reduces the time required for the onset of pharmacological action. Moreover, as the molecules normally selected for delivery by iontophoresis do not permeate passively across the skin, cessation of the electric current will terminate drug transport unless a drug depot is formed in the stratum corneum (Cazares-Delgadillo et al. 2010c).
The ability of the applied current profile to exert tight control over drug concentrations in the blood was highlighted in an investigation into the transdermal iontophoretic delivery kinetics of zolmitriptan – used for the treatment of migraine – from an iontophoretic patch system in Yorkshire swine in vivo, drugs levels closely followed the variations in the applied current, and the drug was detected in the blood after only 2.5 min (Patel et al. 2009). This tight control of dosing and input kinetics also allows therapies to be tailored to meet individual patient needs.
In addition, the iontophoretic current can be used to enable delivery kinetics that mimic the physiological secretion release profiles of endogenous molecules. This is of particular relevance for the use of peptides and proteins as therapeutic drugs; the skin represents a significantly less challenging enzymatic barrier than the oral route even though it does contain metabolizing enzymes (Zhang et al. 2009). Until recently, there was a general consensus that drug candidates for iontophoresis would have to be small hydrophilic molecules (<500 Da) or, at best, cationic peptides that could benefit from EO for delivery into the skin under physiological conditions (Guy et al. 2000). However, recent studies demonstrating the electrotransport of proteins are helping to expand the range of applications of this technology (Cazares-Delgadillo et al. 2007; Dubey and Kalia 2010, 2011; Dubey et al. 2011). In 2007, it was shown that it was possible to deliver cytochrome c, a 12.4 kDa protein, noninvasively across intact skin and that EM was the dominant mechanism accounting for 90 % of transport (Cazares-Delgadillo et al. 2007). Subsequently, ribonuclease A (13.6 kDa with a pI of 8.64) was successfully delivered across porcine and human skin demonstrating that, in addition to structural integrity, biological activity was also retained post-iontophoresis (Dubey and Kalia 2010). More recently, it was shown that transdermal iontophoresis was also able to deliver (i) a biologically active negatively charged protein (ribonuclease T1; 11.1 kDa and a pI of 4.27) (Dubey and Kalia 2011) and (ii) biologically active human basic fibroblast growth factor (17.4 kDa), in therapeutically relevant amounts (Dubey et al. 2011). These results demonstrate that it is possible to administer complex biomolecules noninvasively across the skin.
4.3 Application Sites Other than the Skin
Iontophoretic transport across several other biological tissues has been investigated for either local or systemic delivery, e.g., the buccal (Campisi et al. 2010) and nasal mucosae (Lerner et al. 2004), the sclera (Chopra et al. 2010), the cornea (Vaka et al. 2008), and the nail (Delgado-Charro 2012; Nair et al. 2009). The only physical requirement is that the drug formulation and the electrodes can be correctly positioned and that electrical contact is maintained throughout the current application period. The risk of irritation at the site of application is decreased by the use of low current densities. The maximal current supported by each tissue depends on the resistance of the tissue to current flow, given by Ohm’s law (Eq. 4.1):

where I is the current applied through the tissue in amperes, V is the potential difference measured across the tissue in volts, and R is the resistance of the tissue in ohms. Tissues with greater aqueous content such as the sclera and the cornea offer a lower resistance to current flow, and higher current densities can be applied using lower potential differences; less heat is generated, and this reduces the risk of irritation or pain as compared to other less conductive tissues, with more lipophilic character, such as the skin. In fact, while the maximum current density generally applied on the skin is 0.5 mA/cm2 (Ledger 1992), tolerability studies in humans showed the maximum current density tolerated by the sclera was 5.5 mA/cm2 applied for 20 min or half this current density applied for double the time (i.e., 40 min) (Parkinson et al. 2003). Nevertheless, factors such as application time and surface area along with current density need clinical evaluation for each protocol to optimize the treatment efficiency and safety.

(4.1)
Conclusion
Our understanding of iontophoretic drug delivery has improved considerably since it was first reported more than 100 years ago. However, it is only in the last decade that the FDA has approved prefilled commercial iontophoretic patch systems and only in the past 5 years that we have seen the feasibility of delivering biologically active proteins noninvasively across the skin. As the technologies involved in making transdermal iontophoretic systems progress, this should open the door to new opportunities for development. In parallel, studies exploring electrically assisted delivery across other biological tissue also provide new therapeutic applications of the technology. Recent studies indicate that iontophoresis is a promising strategy for delivering both small and high molecular weight molecules, e.g., antibodies, noninvasively to the eye through the sclera (Chopra et al. 2010). This was clearly demonstrated by in vitro experiments investigating the iontophoretic delivery of bevacizumab (Avastin®, Genentech, Inc., CA, USA) using human sclera as the barrier and a current density of 3.8 mA/cm2; current application resulted in a 7.5-fold enhancement in delivery. Bevacizumab is a recombinant humanized monoclonal antibody used in ophthalmology (off-label) for the treatment of neovascularization in diseases such as diabetic retinopathy and age-related macular degeneration (wet form). As it is currently administered by repeated intravitreal injection, which can cause severe complications, noninvasive administration by iontophoresis could improve compliance and efficacy (Pescina et al. 2010).
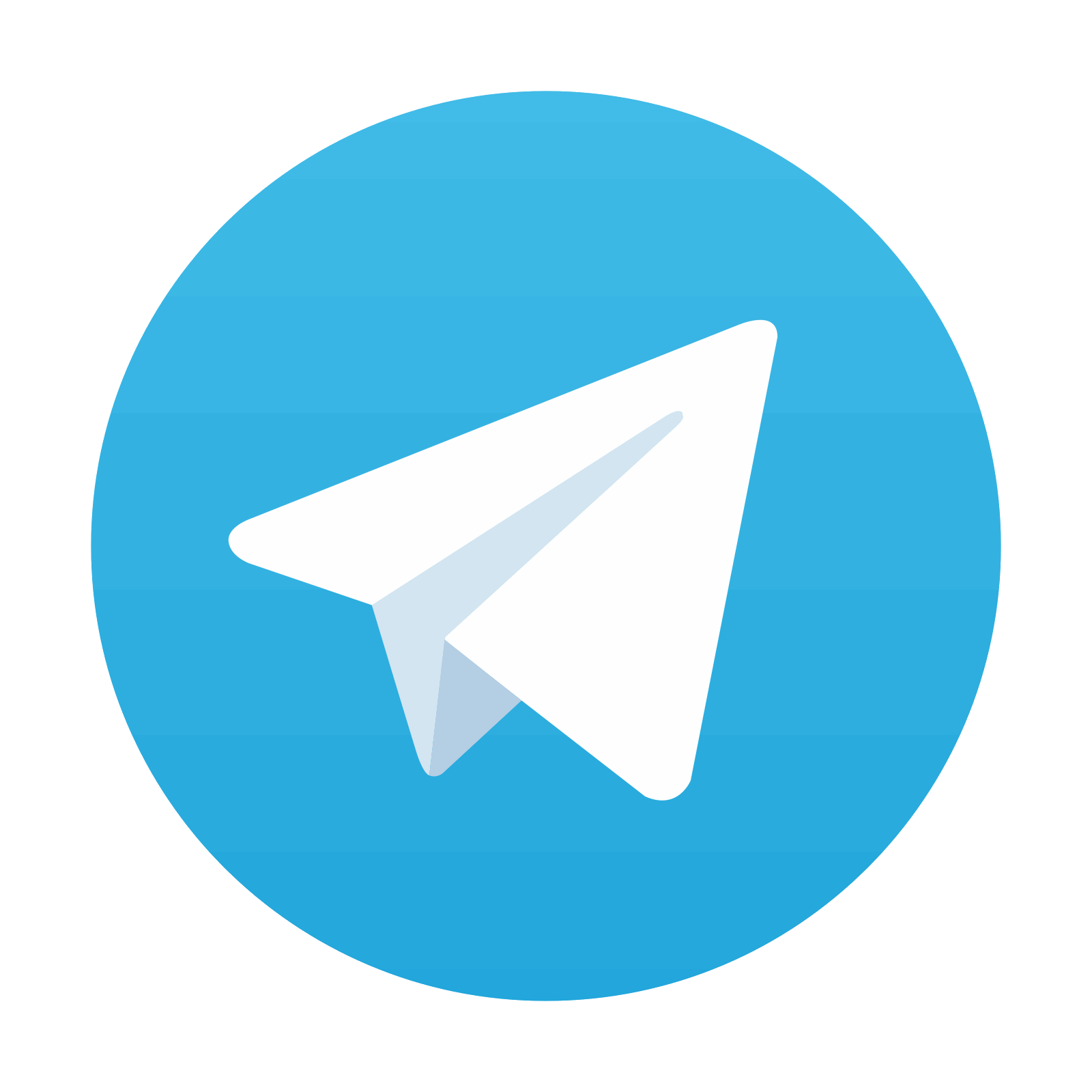
Stay updated, free articles. Join our Telegram channel

Full access? Get Clinical Tree
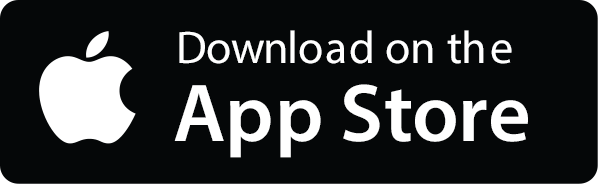
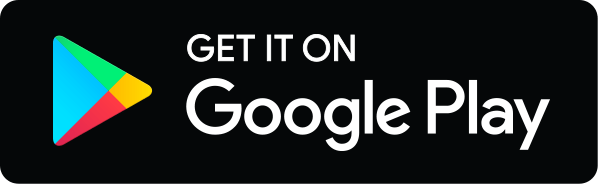