Sustaining an inhalation injury increases the risk of severe complications and mortality. Current evidential support to guide treatment of the injury or subsequent complications is lacking, as studies either exclude inhalation injury or design limit inferences that can be made. Conventional ventilator modes are most commonly used, but there is no consensus on optimal strategies. Settings should be customized to patient tolerance and response. Data for pharmacotherapy adjunctive treatments are limited.
Key points
- •
Management of inhalation injuries is complex and significantly increases the risk of morbidity and mortality.
- •
There remains a paucity of quality evidence to guide treatment.
- •
There is no universally accepted ventilation mode and setting should be customized according to patient compliance, airway resistance, and work of breathing.
Introduction/epidemiology
The management of a large burn can prove to be extremely challenging. When this is compounded by the presence of smoke inhalation, the care gets even more complex with a significant increase in both morbidity and mortality. , The Cocoanut Grove fire was a nightclub fire which took place in Boston, Massachusetts, on November 28, 1942, resulting in the deaths of 492 people. , The majority who perished on the scene suffered from smoke inhalation.
Inhalation injury is defined as the aspiration and/or inhalation of superheated gasses, steam, hot liquids, or noxious products of incomplete combustion (found in smoke). The severity of the injury is related to the temperature, composition, and length of exposure to the inhaled agent. The incidence is around 2% to 14% of patients admitted to burn centers. Inhalation injury can occur with or without a skin burn. A significant number of fire-related deaths are not due to the skin burn, but to the toxic effects of the by-products of combustion (airborne particles). Burns that are associated with carbon monoxide (CO) and/or hydrogen cyanide (CN) poisoning, hypoxia, and upper airway edema can pose serious challenges during the early clinical course of a patient with inhalation injury. Individuals of all age (pediatric/adults and seniors) are at a higher risk of death when their thermal injury is complicated by smoke inhalation. Inhalation injury greatly increases the incidence of respiratory failure, acute respiratory distress syndrome (ARDS), and death.
Pathophysiology
Upper airway injuries are usually a result of thermal injuries and involve the mouth, oropharynx, and larynx. In rare cases, such as steam inhalation in an enclosed space, heat can cause subglottic injury. , Lower airway injuries tend to be caused by chemical injuries or particulate matter from smoke and involve the trachea, bronchi, and alveoli.
Thermal injury to the airway causes denaturation of proteins, which activates the complement cascade and the release of histamine. It also releases free radicals, which combine with nitric oxide in the endothelium of the airway and cause airway edema by increasing the microvascular pressure and tissue permeability. The continued presence of pro-inflammatory cytokines and free radicals leads to worsening airway edema, contributing to increased resuscitation requirements during acute burn resuscitation.
Generally, the upper airway protects the lower airway from heat, so lower airway inhalation injury is usually caused by the chemicals present in smoke, which cause a pro-inflammatory response due to their caustic nature. The presence of chemicals in the airways causes increased bronchial blood flow, which leads to increased permeability and destruction of the bronchial epithelium as well as bronchospasm. , Bronchial blood flow is increased 10-fold, secondary to local cellular damage, and loss of hypoxic pulmonary vasoconstriction. , Initially, the goblet cells produce copious secretions, which eventually harden and become casts, causing airway obstruction. The coagulation cascade is also initiated during an injury secondary to smoke inhalation. This occurs due to a combination of coagulation factors from plasma exuded following vascular injury, tissue factor present in pulmonary endothelial cells, and alveolar macrophages, which results in fibrin deposits in the alveolar space. The combination of loss of hypoxic pulmonary vasoconstriction, increased blood flow to injured lung parenchyma, decreased ventilation of collapsed lung segments, decreased pulmonary compliance, increased pulmonary resistance, and edema contributes to ventilation-perfusion mismatch and profound hypoxemia. ,
Diagnosis
Patients with acute burn injury are evaluated initially using the Advanced Burn Life Support protocol. After the primary survey using ABCDE (Airway, Breathing, Circulation, Disability, Exposure), the secondary survey calculates the percentage total burn surface area (TBSA) involved. A proper history and examination are done to determine the likelihood of inhalation injury ( Box 1 ).
History
- •
Reported mechanism of burn
- •
Potential and duration of exposure to flame, smoke, or chemicals
- •
Exposure in an enclosed space
- •
Loss of consciousness or disability
- •
Headache, delirium, hallucinations, or comatose
- •
Burning sensation in the nose or throat
- •
Cough with increased sputum production
- •
Stridor or odynophagia after smoke exposure
- •
Wheezing or dyspnea with rhonchi
- •
Examination
- •
Facial burns, singed eyebrows, facial, or nasal hair
- •
Soot or carbonaceous material on the face, airway, or sputum
- •
Signs of upper airway obstruction (eg, hoarseness, retraction, stridor, drooling, dysphagia)
- •
Suspicion of lower airway disease (eg, tachypnea, decreased breath sounds, wheezing, rales, rhonchi, or use of accessory respiratory muscles)
- •
Laboratory test
- •
Complete blood count
- •
Basic metabolic panel (blood urea nitrogen, creatinine, electrolytes)
- •
Lactate
- •
Toxicology screen
- •
Arterial blood gas
- •
Carboxylated hemoglobin and methemoglobin
- •
Cyanide concentration
- •
Imaging
- •
Chest radiograph
- •
Bronchoscopy
- •
Computed tomography of the chest
- •
Considering the presence of inhalation injury is an independent predictor of mortality, and it is crucial to have a high index of suspicion. Fiber-optic bronchoscopy, radionuclide imaging with 133 Xenon, computed tomography (CT), elevated carboxyhemoglobin (COHb), and pulmonary function testing (PFT) have all been considered for diagnosing inhalation injury. Bronchoscopy is now considered the gold standard for diagnosing inhalation injury. Testing with xenon-133 or PFT is not always available or acutely practical. Hassan and colleagues demonstrated the arterial partial pressure of oxygen to the fraction of inspired oxygen ratio (P/F) as a predictor of survival once the initial burn resuscitation has been completed. However, the P/F is easily affected by the mode of ventilation as well as resuscitation volumes. Patients with inhalation injury may present with a normal chest radiograph and arterial blood gas. Oh and colleagues found that the radiologist’s score added to bronchoscopy findings could enhance prognostication of a composite endpoint of pneumonia, acute lung injury/ARDS, and death. Yamamura and colleagues showed that CT findings of greater than 3.0 mm bronchial wall thickness 2 cm distal to the carina had a statistically significant correlation with development of pneumonia, number of days on ventilator, and intensive care unit (ICU) length of stay. CT findings could be used complementary to bronchoscopy findings.
Bronchoscopy
Bronchoscopic findings can be important in managing patients and can also have therapeutic effects. , Hassan and colleagues showed TBSA, age, and bronchoscopic findings had a positive correlation with mortality. Flexible bronchoscopy is unable to evaluate damage in the distal airways leading to sometimes discordance in bronchoscopy findings and overall mortality. Patients with inhalation injury are at high risk of ventilator-associated pneumonia, increasing mortality risk upward of 60%. It is a common practice to obtain bronchoalveolar lavage at the time of bronchoscopy.
Bronchoscopy has been used for suctioning out inspissated secretions, casts, and foreign bodies, which may be causing airway obstruction. The most commonly used grading system is the Abbreviated Injury Scale, which takes into account absence or presence of carbonaceous deposits, erythema, edema, bronchorrhea, or obstruction.
The role of repeated bronchoscopies remains controversial, and literature has only retrospective studies or case reports. A retrospective review showed a decreased duration of mechanical ventilation (MV), shorter length of ICU stay, and hospital stay for patients who underwent repeated bronchoscopies. There was also a trend toward mortality benefit favoring repeated bronchoscopies. It has become a common practice to perform repeated daily bronchoscopies in patients with initial grade 2 or higher degree of inhalation injury.
Intubation
The focus of airway management during the first 24 hours is to maintain airway patency with adequate oxygenation and ventilation. Inhalation injury frequently increases respiratory secretions and may generate a large amount of carbonaceous debris in the patient’s respiratory tract. Frequent and adequate suctioning is necessary to prevent occlusion of the airway and endotracheal tube (ETT). Orotracheal intubation using a cuffed ETT is preferred. In adults, if possible, the ETT should be of sufficient size to permit adequate pulmonary toilet and diagnostic and therapeutic bronchoscopy following transfer to the burn center. In children, cuffed ETTs are also preferred using an age-appropriate size. In instances where non-burn trauma mandates cervical spine protection (eg, motor vehicle collisions and falls), cervical spine stabilization is critical during intubation. In impending airway obstruction, clearance of the cervical spine should be postponed.
Indications for early intubation:
- •
Signs of airway obstruction: hoarseness, stridor, accessory respiratory muscle use, sternal retraction
- •
Extent of the burn (TBSA burn greater than 40%–50%)
- •
Extensive and deep facial burns
- •
Burns inside the mouth
- •
Significant edema or risk for edema
- •
Difficulty swallowing
- •
Signs of respiratory compromise (ie, inability to clear secretions, respiratory fatigue, poor oxygenation, or ventilation)
- •
Decreased level of consciousness where airway protective reflexes are impaired
- •
Anticipated patient transfer of large burn with airway issue without qualified personnel to intubate in route
The timing of intubation in burn patients with inhalation injury is crucial. Esnault and colleagues showed patients with face and neck burns who were intubated at the burn center had a five times higher chance of encountering a difficult intubation as compared with prehospital intubation by Emergency Medical Services (EMS). With increasing facial swelling and edema, there may be distortion of the normal upper airway anatomy making intubation difficult.
Although it is important to intubate patients in a timely manner, it is equally essential to avoid unnecessary intubations. A recent retrospective analysis showed that one-third of the intubated patients were extubated within 24 hours and more patients intubated at a non-burn center had early extubation compared with patients intubated at a burn center or by EMS (42% vs 20%). Almost 80% of patients extubated early did not have any evidence of inhalation injury. This study underlines the importance of quickly getting patient to an experienced burn center to correctly identifying patients with inhalation injury.
Early tracheostomy
Tracheostomy is a commonly performed procedure in critical care and helps in ventilation weaning, reducing amount of sedation required, decreasing ICU delirium, and improving bronchial hygiene with a secure airway. The literature however is controversial when it comes to using tracheostomy for burn patients with inhalation injuries. Laryngotracheal stenosis may occur in up to 24% of patients with inhalation injury. Aggarwal and colleagues did not show any mortality difference in burn patients who underwent tracheostomy versus those who did not. Although evidence does not support prophylactic tracheostomies, a survival benefit was seen with tracheostomy in patients with 60% TBSA burns. Tracheostomy is not associated with increased pneumonia; however, persistent tracheostomy is a commonly reported complication.
Tsuchiya and colleagues showed that there is no mortality difference between early (mean day 4) versus late (mean 2 weeks) tracheostomies in burn patients. Early tracheostomy however has been associated with improvement of the P/F, fewer days on MV, shorter hospital length of stay, and earlier first day of active exercises. , There is a 16 times increased risk of dysphagia in burn patients with inhalation injury compared with patients without inhalation injury. Smailes and colleagues showed that this risk of dysphagia increased if tracheostomy was performed more than 7 days after oral intubation and early tracheostomy could potentially decrease this risk of developing dysphagia. Stefan and colleagues published a normogram to predict the need for tracheostomy in which age, TBSA, and inhalation injury were significant factors. Further studies will be needed to validate the utility of the normogram in clinical practice.
High versus low tidal volume ventilation
Low tidal volume ventilation has consistently been validated for the treatment of ARDS in adults. This MV strategy has shown to improve morbidity and mortality from ARDS. Lung-protective ventilation is based on the principle of limited tidal volumes (6–8 mL/Kg of ideal body weight and plateau pressures of <30 cm H 2 O). However, the ARDSNET trial excluded burn patients with greater than 30% TBSA.
Given the unique characteristics of burn patients, such as airway obstruction from fibrin casts and decreased chest compliance, low tidal column ventilation can be challenging. In contrast, a single retrospective study on pediatric patients suffering from inhalation injury found that high tidal volume ventilation (15 ± 3 mL/kg) significantly decreased ventilator days and the incidence of both atelectasis and ARDS. Although optimal tidal volume remains undefined, most experts recommend limiting tidal volumes to the lowest level tolerated by the patient’s compliance, airway resistance, and work of breathing.
Modes of mechanical ventilation
Ventilator management of patients with inhalation injury might deviate from conventional practices given their unique physiology. Currently, there is no single ventilator mode that prevails, and this is due to a paucity of clinical trials. Larger randomized trials are needed to elucidate what is the best mode of MV for patients with inhalation injuries. Chung and colleagues found that conventional modes, particularly pressure support ventilation and volume-assist control modes, were the most used modes in North America. However, unconventional modes are also being used as discussed. If a conventional mode is to be used, providers should aim to limit the tidal volume to the lowest level tolerated by the patient’s compliance and airway resistance.
Airway Pressure Release Ventilation
Airway pressure release ventilation (APRV) is an inverse ratio, pressure-controlled mode of ventilation that allows for spontaneous breaths. APRV has not been shown to improve survival. One trial conducted in China, on patients with ARDS, found that APRV use was associated with shorter durations of both MV and ICU stay. Batchinsky and colleagues found no survival benefit of APRV use versus conventional therapy in patients with inhalation injury and respiratory failure.
High-Frequency Percussive Ventilation
Cioffi and colleagues first described high-frequency percussive ventilation (HFPV) in 1989 as a mean of ventilatory support, assisting with clearance of secretions, as well as decreasing barotrauma and pneumonia. HFPV delivers very small, high-frequency tidal breaths that lower airway pressures as well as produce intrabronchial percussion and airway turbulence. This action helps mobilization and clearance of secretions and plugs.
The use of prophylactic HFPV in patients with inhalation injury has demonstrated benefits in mortality, incidence of pulmonary infection, and barotrauma; however, it is important to mention that this study on 54 patients between 1987 and 1990 was compared with a historic cohort. In a single-center prospective, randomized, controlled clinical trial, comparing HFPV with low tidal volume ventilation in patients admitted to a burn intensive care unit with respiratory failure (approximately 35% had inhalation injury), the researchers found no difference in ventilator-free days or mortality.
High-Frequency Oscillatory Ventilation
High-frequency oscillatory ventilation delivers very small tidal volumes at high frequency to maximize lung recruitment with sustained mean airway pressure. Given the pathophysiology of inhalation injury and bronchial obstructive changes, this mode is unlikely to be effective. Cartotto and colleagues compared patients with ARDS secondary to inhalation injury versus ARDS from burns without inhalation injuries and found that the former group had worsening hypoxia and hypercapnia. This ventilation strategy has also failed to prove any benefit on ARDS patients without inhalation injury in two large randomized controlled trials. ,
Extracorporeal Membrane Oxygenation
Extracorporeal membrane oxygenation (ECMO) is usually seen as a rescue strategy when every other measure to improve oxygenation has failed. Data on burn patients are extremely scarce. A meta-analysis published in 2013 showed no improvement in survival for burn patients suffering from acute hypoxemic respiratory failure. A recent registry showed that the mortality of burn patients (inhalation injury 26.7%) receiving ECMO was comparable that for non-burn ECMO patients.
Complications
Pulmonary Edema
Pulmonary edema is the excessive accumulation of fluid in the lung parenchyma. Non-cardiogenic pulmonary edema is caused by increase in capillary permeability and changes in the pulmonary gradient within the pulmonary capillaries. Pulmonary edema occurs in the presence of inhalation injury but may be worsened during acute burn resuscitation. Those with inhalation injuries commonly require higher than calculated resuscitation, which increases the risk for pulmonary edema and other complications associated with over resuscitation. Fluid creep is a phenomenon, described by Pruitt, in which more fluid is given than is calculated using the standard formulas. This results in abdominal and extremity compartment syndrome, pulmonary and cerebral edema, ARDS, and multiple organ failure, thereby increasing morbidity and mortality in burn patients.
Acute Respiratory Distress Syndrome
Epidemiology
ARDS was originally described by Ashbaugh and colleagues. Later, in 2012, the ARDS task force introduced the Berlin definition; this criterion specifies risk factors, timing of onset, and replaces the previous acute lung injury category by identifying three levels of ARDS severity. The levels of ARDS severity are based on P/F.
Patients suffering from either cutaneous burns or inhalation injury are at an increased risk of developing ARDS. Belenkiy and colleagues found that 33% of mechanically ventilated burn patients developed ARDS and these patients had a mortality rate of 33%. Lam and colleagues found that patients with inhalation injury developed ARDS sooner and were more hypoxic when compared with burn patients without inhalation injury.
Protective ventilation
Burn patients with ARDS have historically been excluded from clinical trials. Based on the ARDSnet findings on non-burn patients, low tidal volume ventilation (6–8 mL/Kg of ideal body weight and plateau pressures of <30 cm H 2 O) has consistently shown decreased mortality as well as decreased ventilator days and barotrauma. The use of higher positive end-expiratory pressure in addition to low tidal volume helps minimize atelectrauma and has shown improvement in survival. However, the use of low tidal volume ventilation in patients with ARDS secondary to inhalation injury can be challenging given the unique pathophysiology. Data from a pediatric study showed benefits when using higher tidal volumes.
Prone positioning
Prone ventilation refers to the delivery of MV, whereas the patient is lying in the PP. This maneuver improves gas exchange by ameliorating the ventral-dorsal transpulmonary pressure difference, reducing dorsal lung compression, improving lung perfusion, and providing more homogeneous aeration of the lung. The PROSEVA trial, a multicenter, prospective, randomized, controlled trial on non-burn patients with ARDS, found that in patients with P/F of less than 150 mm Hg, early prolonged PP significantly decreased 28-day and 90-day mortality. Data on burn patients are limited. A case series of 18 burn patients with ARDS showed improvement in oxygenation when PP was applied.
Inhaled pulmonary vasodilators
Inhaled pulmonary vasodilators selectively dilate the vessels that perfuse well-ventilated lung zones, resulting in improved oxygenation due to better ventilation/perfusion (V/Q) matching. A meta-analysis of 12 trials from non-burn ARDS patients found that the usage of nitric oxide improved oxygenation without improving survival. Small studies on both pediatric and adult burn patients showed improvement in oxygenation parameters.
Neuromuscular blockade
Neuromuscular blockade agents (NMB) were used quite frequently in the past for patient with severe ARDS. This practice was due to the findings from the ACURASYS trial. The study showed that patients receiving NMB had a decrease in mortality, more ventilator free days and less barotrauma. Nine years later the publishing of the ROSE trial debunked that theory showing no difference in patients with severe ARDS using NMB. Currently, there is no specific evidence to guide the use of NMB in burn-injured patients with ARDS, unless it is necessary for ventilator desynchrony.
Corticosteroids
The use of corticosteroids for ARDS remains controversial as there is still no consensus on its use. The ARDSnet trial, the largest trial to date, showed no difference in mortality. However, when all the studies are taken together, the data suggest a probable benefit in mortality. There are no data published specific to burn patients, and for this reason, the decision to use corticosteroids must be approached carefully, weighing the risk and benefits specific to the population.
Pneumonia
The most common complication in patients with inhalation injury is pneumonia. The combination of airway injury, debris, impaired clearance of mucous, and obstruction of small airways leads to the development of pneumonia. Those with inhalation injuries are at significantly higher risk of developing pneumonia than burned without an inhalation injury. In some studies, the incidence of pneumonia in those with inhalation injury ranges from 27% to 70% with a mortality rate as high as 60%. , In a study by Liodaki and colleagues, the development of pneumonia increased length of stay (36.0 days vs 17.1 days, P < .01) and those with larger burns were at higher risk of developing a pneumonia (the mean TBSA in the pneumonia group was 24.8 vs 16.9 in the non-pneumonia group, P < .01). The investigators also found that 73.9% of patients who developed pneumonia required a tracheostomy with an average number of days on the ventilator of 25.2 days.
The diagnosis of pneumonia is a combination of clinical examination, radiographic findings, and microbiologic evidence. Typically, those with pneumonia will have an elevated white blood cell count, fever, tachycardia, and purulent respiratory secretions and may have evidence of worsening respiratory status (eg, increased respiratory rate and increased ventilator requirements). It may be difficult to elucidate whether these clinical signs/symptoms and laboratory findings are indicative of infection or are normal in the setting of burn injuries, given that it is a pro-inflammatory state. A chest radiograph may assist in the diagnosis of pneumonia. The classic finding is a consolidation or infiltrate. If there is a suspicion of pneumonia, bronchoscopy and bronchoalveolar lavage can be performed to obtain cultures.
Once there is a clinical concern of pneumonia, empirical antibiotics should be started. The local antibiogram of the hospital can help guide antibiotic choice. The most common organisms in ventilator-associated pneumonias are Pseudomonas, Staphylococcus aureus , and Enterobacteriaceae . Cultures should be obtained to appropriately narrow antibiotics and direct therapy. Shorter courses are preferred over longer courses, typically 7 to 10 days.
Exogenous Surfactant
After severe smoke inhalation injury, the lung loses its ability to protect itself through many mechanisms. Exogenously replacing the lost surfactant has been proposed as a strategy to mitigate post-inhalation injury complications. , , Sen and colleagues conducted a retrospective, case series ( n = 7) of pediatric patients given inhaled calfactant 80 mL/m 2 every 12 hours for two doses and found improved oxygenation in the four patients treated early after injury.
Inhaled Heparin
Originally studied in an ovine model, the use of inhaled heparin has been shown to reduce tracheobronchial cast formation, improve oxygenation, minimize barotrauma, and reduce pulmonary edema after inhalation injury. Early small clinical studies have demonstrated mixed results, and the planned large multicenter, randomized controlled trial was halted due to difficult enrollment and high costs associated with conducted trial. In a retrospective cohort study, inhaled unfractionated heparin (UFH) 5000 units administered during the first 72 hours failed to demonstrate a reduction in pneumonia, ventilator days, mortality, or length of stay. Elsharnouby and colleagues conducted a prospective, double-blind randomized study and found inhaled UFH 10,000 units (vs 5000 units) improved lung injury scores and reduced duration of MV.
Following the positive findings of their initial single-center, retrospective cohort study, Walroth and colleagues conducted a similar two-center study comparing two different dosing arms to a control group ( n = 105). , The interventional treatment protocol consisted of inhaled UFH (5000 units or 10,000 units) given every 4 hours and an inhaled mucolytic (acetylcysteine or sodium bicarbonate) plus albuterol every 4 hours. Inhaled therapies were initiated on admission, alternated, and administered such that a nebulized treatment was given every 2 hours for 7 days (or until extubation, if sooner). Ventilator-free days in the first 28 days were significantly improved in the two UFH groups versus control (heparin 5000 units 22.0 ± 9.0, heparin 10,000 units 17.9 ± 7.0, control 13.6 ± 9.0; P < .001). Similarly, there were significantly lower rates of ventilator-associated pneumonia and shorter lengths of stay in the groups administered inhaled UFH. Although the treatment groups were well-matched to controls based on injury severity and age and the investigators describe similar practices between centers, the analysis is limited by the retrospective design, including only two centers without controlling for potential center effect and assumptions in other aspects of clinical management. Although blood-stained sputum is common after severe smoke inhalation injury and large doses (150,000 units) of inhaled UFH have been noted to have systemic absorption over time resulting in increased activated partial thromboplastin time, doses of 10,000 units of inhaled UFH seem safe and well tolerated, as demonstrated in this large cohort and several preceding studies. , , ,
Toxic exposures
In cases of CO poisoning, there is reduced oxygen carrying capacity of the hemoglobin and the oxygen dissociation curve shifts to the left, leading to tissue hypoxia and subsequent organ dysfunction. These patients should be placed on 100% humidified Fi O 2 via non-rebreather, if not already intubated, reducing the half-life of CO from 4 hours to about 45 minutes. COHb values should be repeated hourly until the value is less than 10%, where Fi O 2 can be weaned. Depending on the degree of exposure, neurologic sequelae may persist.
CN toxicity can lead to tissue hypoxia by binding to and causing a conformational change in cytochrome-c oxidase, thereby, blocking mitochondrial respiration and the formation of adenosine triphosphate. Although amyl and sodium nitrite can function as an antidote, they should be considered contraindicated in severe respiratory insufficiency, because they work as an antidote by forming methemoglobin. Methemoglobin can potentiate poor oxygen delivery, as it also has a high affinity for oxygen. Sodium thiosulfate has been used successfully but is noted to have a slow onset and poor penetration into the central nervous system and mitochondria, where symptoms are most present and mechanism relies. Hydroxocobalamin has been proposed as an effective antidote due to its rapid onset and distribution with a mechanism of directly binding CN to form cyanocobalamin to ultimately eliminate in the urine. , , In addition, hydroxocobalamin is a nitric oxide scavenger and inhibits nitric oxide synthase, so it can produce profound hypertension over the subsequent hours. Although used off-label in vasoplegia, the hypertensive effects may be less prominent in patients with shock. Outside of hypertension, adverse effects can include headache, chromaturia or hyperpigmentation, and colorimetric-based laboratory inaccuracies. Although some have found it efficacious, cost-effective, and well tolerated (even prehospital use), others have associated its use with higher rates of acute kidney injury.
Mass casualty events, whether intentional or from neglect, can result in significant degrees of inhalation injuries with unknown chemical exposure. Although toxic exposures should be a part of the differential, patients often present with overlapping symptoms. Cases of passive exposure to chlorine gas or inappropriate handling of concentrated acids and bases are highly reported. , ,
Future directions
There are still more pathophysiological stones to roll over. Work from the Glue Grant and more recently the SYSCOT study group has demonstrated the presence of a “genomic storm,” leukocyte changes, and early transcriptomic evidence of immune pathway shutdown in severe burn injuries. As discussed, ventilatory management of patients with varying degrees of inhalation injury and acute lung injury is still widely variable between centers and experts. There is limited evidential support for pharmacotherapeutic options beyond inhaled UFH, which may be aided by advancing technologies and epigenomic findings. Related, patients with severe inhalation injury have significantly higher odds of developing pneumonia. What roles do microbial diversity, antimicrobial exposure, or its manipulation play in outcomes or potential treatment? Bacteriophage therapy seems like a promising route for future investigation in pneumonia. What roles should blood purification or extracorporeal organ support have in severe inhalation injury? Can early mobilization reduce complications following inhalation injury? In 2018, the American Burn Association’s Cutaneous Thermal Injury and Inhalation Injury working group identified three priorities for future research: (1) airway repair mechanisms, (2) airway microbiome, and (3) biomarker identification.
Summary
Management of a thermal burns associated with an inhalation injury has increased the risk of mortality and morbidity in burn patients. A multidisciplinary approach consisting of physicians, nurses, therapists, pharmacists, and other key members of a burn team is necessary in optimizing outcomes. Care should be directed toward early diagnosis and referral, supportive care, airway protection with aggressive airway management, fluid resuscitation, treatment of CO, and CN poisoning and prevention/management of pneumonia. Improvements in mortality from inhalation injury are mainly due to widespread improvements in critical care rather than focused interventions for smoke inhalation.
Clinics care points
- •
Treatment of severe inhalation injuries can be complicated and should be protocolized.
- •
When there is suspicion of smoke inhalation with elevated carboxyhemoglobin, provide 100% oxygen to reduce the half-life of carbon monoxide.
- •
Diagnostic and therapeutic bronchoscopy should be routinely deployed in the initial treatment of inhalation injury.
- •
Severe inhalation injuries should be treated with inhaled heparin and a mucolytic plus a bronchodilator.
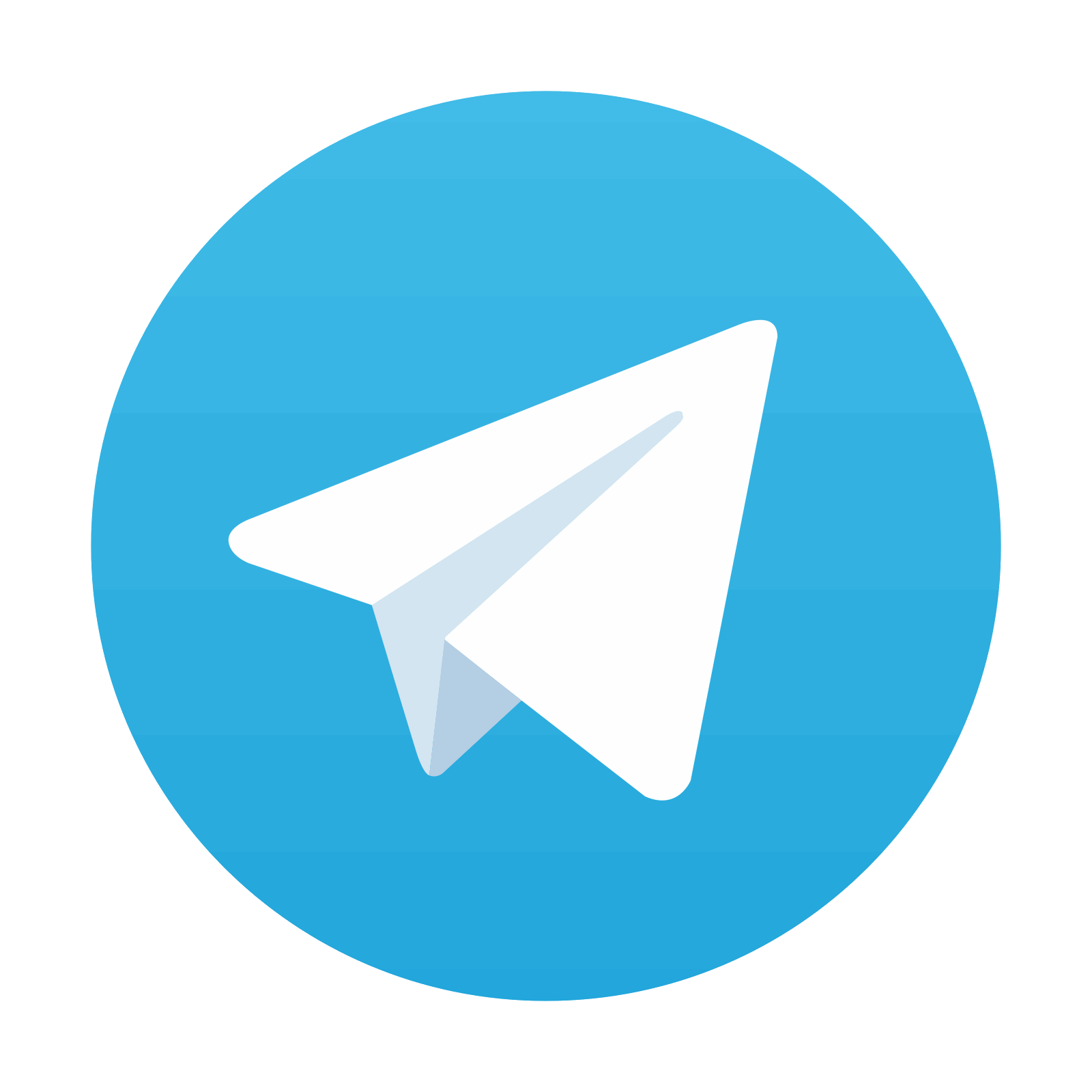
Stay updated, free articles. Join our Telegram channel

Full access? Get Clinical Tree
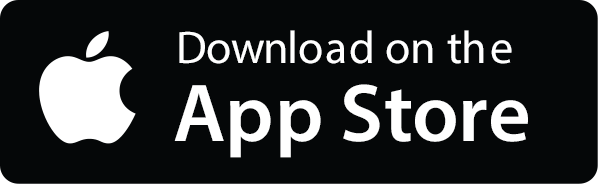
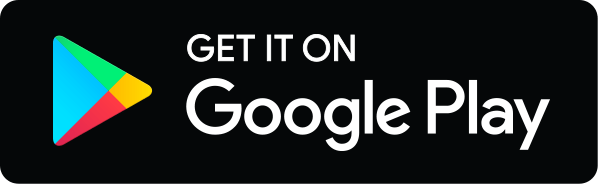
