Among the severe genetic disorders of the skin that are suitable for gene and cell therapy, most efforts have been made in the treatment of blistering diseases including dystrophic epidermolysis bullosa. This condition can be recessively or dominantly inherited, depending on the nature and position of the mutation or mutations in the gene encoding type VII collagen. At present, there is no specific treatment for recessive dystrophic epidermolysis bullosa, and gene and cell therapy approaches hold great promise. This article discusses the different gene therapy approaches that have been used for the treatment of this disease and the new perspectives that they open.
Among the severe genetic disorders of the skin that are suitable for gene and cell therapy, most efforts have been made in the treatment of blistering diseases including dystrophic epidermolysis bullosa (DEB). DEB can be recessively (RDEB, OMIM #226600) or dominantly (DDEB, #131750) inherited, depending on the nature and position of the mutation or mutations in the gene encoding type VII collagen ( COL7A1 ). RDEB is one of the most severe genodermatoses of children and young adults. Individuals with RDEB suffer from lifelong severe skin and mucosal blistering followed by scarring caused by the loss of adhesion between the epidermis and the dermis. Hands and feet in particular are severely affected, and repeated scarring leads to the fusion of digits and their retraction. Aggressive squamous cell carcinomas frequently develop in the areas subjected to repetitive blistering and scarring, and represent the most frequent cause of death in these patients.
The gene encoding type VII collagen, COL7A1 , is segmented into 118 exons, spreads over 32 kb on human chromosome 3p21, and encodes a large cDNA of 8.9 kb. Type VII collagen is synthesized as a 290-kDa α1-chain protein precursor that assembles into a homotrimeric-quaternary structure. Homotrimers further assemble into antiparallel dimers in the extracellular matrix to form anchoring fibrils, which are key structures for the dermal-epidermal adherence.
At present, there is no specific treatment for RDEB, and gene and cell therapy approaches hold great promise. The first successful clinical trial of skin gene therapy was performed in a patient suffering from junctional epidermolysis bullosa (JEB). This pioneer work paved the way for future trials aimed at correcting severe genetic skin disorders including RDEB. Meanwhile, novel strategies and technologies based on recent knowledge have been developed, allowing for successful preclinical studies in skin gene therapy trials using different approaches. This article discusses the different gene therapy approaches that have been used for the treatment of RDEB and the new perspectives that they open.
Therapeutic strategies
The epidermis being a continuously self-renewing tissue, the curative treatment for RDEB must either be repeated throughout the lifetime of the patient or must involve the use or modification of stem cells that are able to permanently reconstitute the tissue. Epidermal stem cells have the capacity to regenerate for a lifetime, and considerable progress has been made in the culture of these human epidermal stem cells. When appropriate culture conditions are used, it is possible to obtain large amounts of cultured epithelia from a small skin specimen, obtained by biopsy, within 3 to 4 weeks. Over the past 20 years, cultured epithelial autografts have been successfully used worldwide to treat extensive third-degree burn wounds. Major improvements have also been achieved in in vitro skin-equivalent systems with fibroblast-containing dermal components, tissue engineering, and surgical procedures. These systems have been reported to improve the quality of engrafted skin and wound healing. Treatment of RDEB by transplantation of genetically modified skin equivalent could thus benefit from these biotechnological advances.
Strategy at the DNA Level
Genomic locus transfer
The first successful gene transfer applied to RDEB was achieved by microinjecting a PAC vector into a COL7A1- deficient cell line. This genomic approach has several advantages such as the presence of the endogenous regulatory elements for physiologic expression of the transgene, and the maintenance of the genomic environment that can contain additional regions involved in the control of gene transcription. However, the efficiency of transfection methods for large DNA molecules is low, and the pursuit of this type of approach could benefit from systems of high capacity DNA vector such as herpes virus-based vectors.
cDNA transfer
For a long time, gene therapy for RDEB has been hampered because of the inability of the large-sized COL7A1 cDNA (8.9 kb) to transfer. The recent progress in vectorology has permitted vectors to accommodate cDNAs as large as 10 kb, and several groups using different approaches have been able to correct primary human keratinocytes and/or fibroblasts derived from patients with RDEB.
Ex vivo gene transfer
The most efficient approach to achieve permanent transgene expression in epidermal stem cells is the ex vivo approach, whereby epidermal stem cells are harvested from the host by a skin biopsy and gene transfer is performed when the cells are growing in tissue culture. Genetically corrected cells are expanded, analyzed, and then grafted back onto the recipient. Several groups have reported successful COL7A1- cDNA transfer into keratinocyte cell lines, primary keratinocytes, or fibroblasts derived from a patient with RDEB using either nonviral gene transfer or virus-mediated gene transfer.
Ortiz-Urda and colleagues have corrected primary keratinocytes from a patient with RDEB using a nonviral gene transfer method. A plasmid encoding the COL7A1 cDNA together with a selectable marker (blasticidin resistance) under the control of the CMV promoter was cotransfected with a plasmid expressing φC31 integrase. This phage-derived integrase mediated the integration of the construct that contained attB sites flanking the expression cassette, at the pseudo-attP sites naturally present in the human genome. Because of the low efficacy of the process, stably modified keratinocytes were selected using blasticidin. Subsequently, the groups of David Woodley and Mei Chen have used a self-inactivating (SIN) lentiviral vector to efficiently transduce immortalized-RDEB keratinocytes or primary-RDEB fibroblasts with the COL7A1 cDNA under the control of a modified retroviral promoter (MND). These investigators have shown functional correction with either keratinocytes or fibroblasts, and have shown that fibroblasts alone can correct the dermal-epidermal adherence defect. Similarly, Goto and colleagues have shown functional correction, by correcting either RDEB keratinocytes or fibroblasts using a classic retroviral vector without selecting the cells with an antibiotic. In parallel, Gache and colleagues have transduced primary-RDEB keratinocytes using a classic retroviral vector expressing COL7A1 cDNA under the control of the viral-LTR (long terminal repeat) and the gene that provides resistance to an antibiotic. The transduced cells were selected with zeocin to enrich the population in corrected cells.
In all cases, demonstration of functional correction was performed using grafting of the genetically corrected cells onto immunodeficient mice to show anchoring fibril formation in vivo in reconstructed human skin. The advantage of the ex vivo gene therapy approach aimed at grafting genetically corrected epithelia or skin equivalents is that it is potentially applicable to every patient with RDEB and that it has already been successful in the treatment of JEB. However, it is a labor-intensive procedure, which is applicable to selected area of the patient’s skin, but is more difficult to apply to affected mucosae.
The groups of Paul Khavari and David Woodley have used intradermal injection of ex vivo genetically corrected RDEB fibroblasts in RDEB skin equivalents grafted onto immune deficient mice. In one case, the fibroblasts were transfected with plasmids allowing for the selection of rare recombination events mediated by the φC31 integrase (as discussed earlier). In the second case, cells were transduced using the SIN-lentiviral vector expressing COL7A1 cDNA under the control of the retroviral-MND promoter. Both groups have shown that intradermally injected fibroblasts synthesized and secreted type VII collagen for up to 4 months, leading to anchoring fibril formation at the dermal-epidermal junction and restoration of the dermal-epidermal adherence. This strategy has the potential to treat large body areas of the skin. However, long-term maintenance has not been documented and the feasibility of this approach in patients remains to be assessed.
Collectively, these pioneering studies have established that corrective gene transfer for RDEB is feasible by different means. This research has provided a starting point for further refinement in future preclinical and clinical efforts using safer SIN-viral vectors expressing the COL7A1 cDNA under the control of human promoters.
In vivo gene transfer
Woodley and colleagues have shown functional correction after in vivo injection of a SIN-lentiviral vector expressing type VII collagen into RDEB skin equivalents grafted onto immunodeficient mice. This approach would avoid the need of culturing the cells, could permit treatment of areas not readily accessible, and theoretically would also offer a systemic treatment. However, in vivo injections of such vectors raise major safety concerns. Using this procedure, the possible expression of the transgene in targeted Langerhans cells or dendritic cells could lead to a higher risk of immune response against recombinant type VII collagen. But the main issue of such an approach is that the viral vector that is injected in vivo in a well-vascularized tissue could disseminate into the body and affect other organs, where the effect of ectopic expression of type VII collagen is unknown, or could genetically modify germline cells—an effect which is prohibited by the ethics committees.
Strategy at the RNA Level
Classic gene therapy approaches for genetic disorders is based on the complementation of a functional deficiency via the transfer of the relevant wild-type nucleic acid sequence to cells and organs. A different methodological principle is to correct the endogenous genetic information itself to direct the biosynthesis of a functional protein product. The exon skipping strategy is based on the capacity of small antisense sequences to mask signals recognized by the spliceosome machinery, thus leading to the excision of the target exon or exons carrying the mutation or mutations. If the target exon sequence is dispensable and the process maintains the open reading frame, it produces a shortened protein, which is partly or completely functional, and which restores the phenotype.
This approach has been successfully applied to Duchenne muscular dystrophy (DMD) in animal models (for a review see the article by Aartsma-Rus and van Ommen ), and a phase 2 clinical trial for DMD is actually ongoing in patients in the Netherlands.
Computational analysis of COL7A1 -genomic sequence revealed that this gene is particularly suited for the exon skipping strategy. Indeed, targeted skipping of any of the 84 exons encoding the central collagenous region preserves the reading frame of the mRNA. Moreover, these exons encode multiples of the Gly-X-Y collagenous repeat, so that the shortening of the protein sequence resulting from exon skipping would preserve the periodicity of these collagenous repeats. Recently, the group of Hiroshi Shimizu has convincingly shown the dispensability of exon 70 of COL7A1 , by transducing RDEB keratinocytes and fibroblasts using a retroviral vector encoding a COL7A1 cDNA in which exon 70 was deleted. This group has also induced in vivo targeted skipping of exon 70 using antisense oligoribonucleotides in RDEB skin equivalents grafted onto nude mice, albeit with low efficacy. Although the efficacy of this approach for RDEB remains to be shown, it offers the opportunity of a noninvasive systemic in vivo treatment using easy-to-manufacture compounds (oligoribonucleotides). Drawbacks are that it can be used only on a subset of patients carrying mutations within exons that are dispensable for type VII collagen function, and it would require lifelong periodic administration.
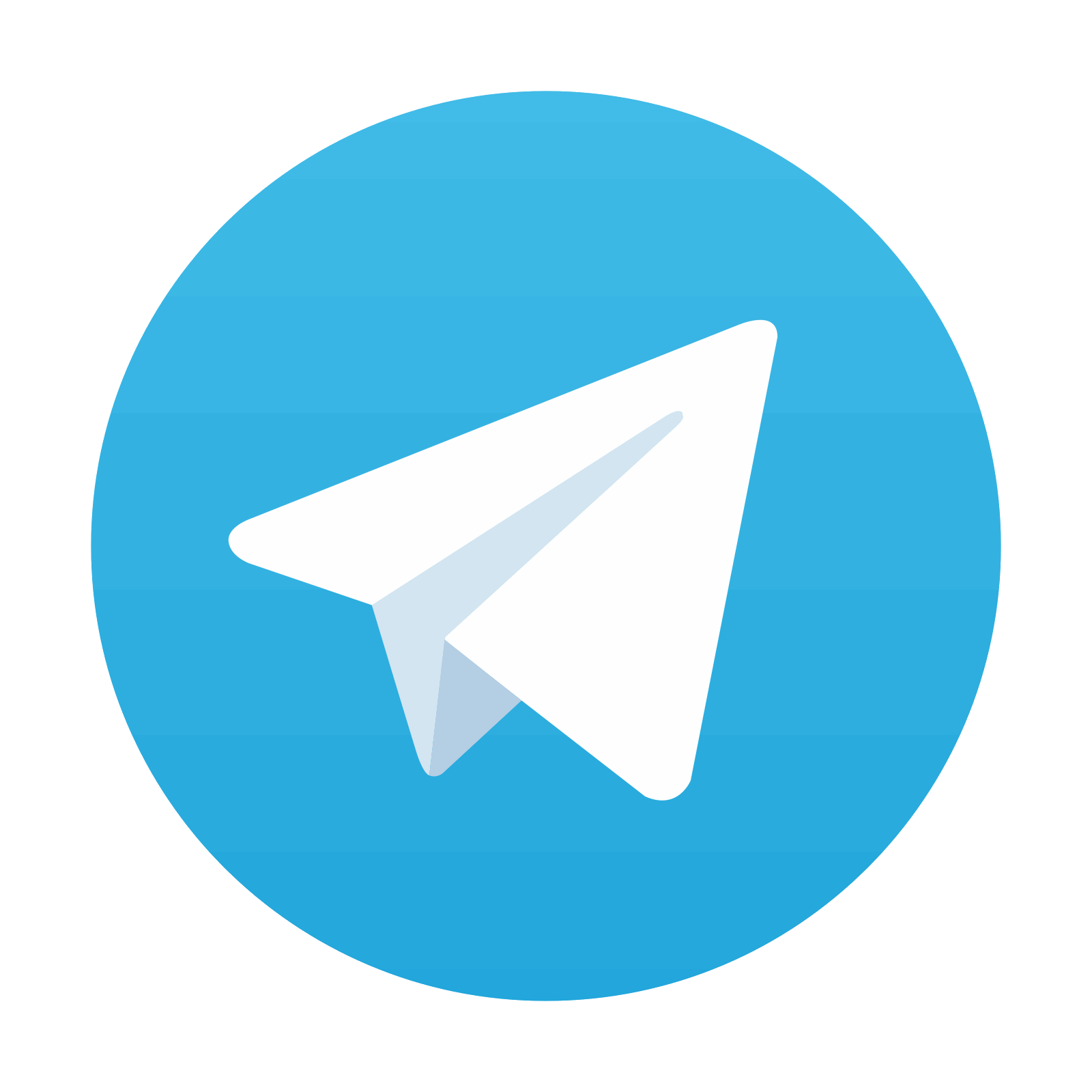
Stay updated, free articles. Join our Telegram channel

Full access? Get Clinical Tree
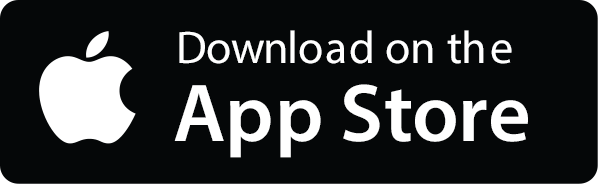
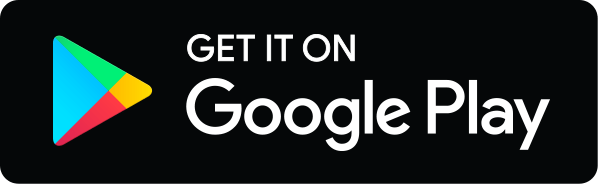