Development and Structure of Skin: Introduction
|
Skin: An Overview
Skin is a complex organ that protects its host from its environment, at the same time allowing interaction with its environment. It is much more than a static, impenetrable shield against external insults. Rather, skin is a dynamic, complex, integrated arrangement of cells, tissues, and matrix elements that mediates a diverse array of functions: skin provides a physical permeability barrier, protection from infectious agents, thermoregulation, sensation, ultraviolet (UV) protection, wound repair and regeneration, and outward physical appearance (Table 7-1). These various functions of skin are mediated by one or more of its major regions—the epidermis, dermis, and hypodermis (Fig. 7-1; see also Fig. 6-1, Chapter 6). These divisions are interdependent, functional units; each region of skin relies upon, and is connected with, its surrounding tissue for regulation and modulation of normal structure and function at molecular, cellular, and tissue levels of organization (see Chapter 6).
Function | Tissue Layer | Some Associated Diseases |
---|---|---|
Permeability barrier | Epidermis | Atopic dermatitis Ectodermal dysplasias Ichthyoses Keratodermas Exfoliative dermatitis Bullous diseases |
Protection from pathogens | Epidermis Dermis | Verruca vulgaris Ecthyma Cellulitis Leishmaniasis Human immunodeficiency virus Tinea pedis/corporis |
Thermoregulation | Epidermis Dermis Hypodermis | Ectodermal dysplasias Raynaud Hyperthermia |
Sensation | Epidermis Dermis Hypodermis | Diabetic neuropathy Leprosy Pruritus Postherpetic neuralgia |
Ultraviolet protection | Epidermis | Xeroderma pigmentosum Oculocutaneous albinism |
Wound repair/regeneration | Epidermis Dermis | Keloid Venous stasis ulcer Pyoderma gangrenosum |
Physical appearance | Epidermis Dermis Hypodermis | Melasma Vitiligo Scleroderma Lipodystrophy |
Figure 7-1
The major regions of skin. Skin is composed of three layers: (1) epidermis, (2) dermis, and (3) hypodermis. The outermost epidermis is separated from the dermis by a basement membrane zone, the dermal–epidermal junction. Below the dermis lies the subcutaneous fat (hypodermis). Epidermal appendages, such as hair follicles and eccrine and apocrine sweat glands, begin in the epidermis but course through the dermis and/or the epidermis. Blood vessels, lymphatics, and nerves course through the subcutaneous fat and emerge into the dermis.
Whereas the epidermis and its outer stratum corneum provide a large part of the physical barrier provided by skin, the structural integrity of skin as a whole is provided primarily by the dermis and hypodermis. Antimicrobial activities are provided by the innate immune system and antigen-presenting dendritic cells of the epidermis, circulating immune cells that migrate from the dermis, and antigen-presenting cells of the dermis (see Chapter 10). Protection from UV irradiation is provided in great measure by the most superficial cells of the epidermis. Inflammation begins with the keratinocytes of the epidermis or immune cells of the dermis, and sensory apparatus emanates from nerves that initially traverse the hypodermis to the dermis and epidermis, ending in specialized receptive organs or free nerve endings. The largest blood vessels of the skin are found in the hypodermis, which serve to transport nutrients and immigrant cells (see Fig. 6-1, Chapter 6). The cutaneous lymphatics course through the dermis and hypodermis, serving to filter debris and regulate tissue hydration. Epidermal appendages provide special protective or sensory functions. Skin also determines a person’s physical appearance, influenced by pigmentation provided by melanocytes, with body contours, appearance of age, and actinic damage influenced by the epidermis, dermis, and hypodermis. The skin begins to be organized during embryogenesis, where intercellular and intracellular signals, as well as reciprocal cross talk between different tissue layers, are instrumental in regulating the eventual maturation of the different components of skin.
What follows is an integrated description of the major structural features of the skin and how these structures allow the skin to achieve its major functions, followed by a review of their embryologic origins. Also highlighted are illustrative cutaneous diseases that manifest when these functions are defective. Understanding the genetic and molecular bases of skin disease has confirmed, and in some cases revealed, the many factors and regulatory elements that play critical roles in skin function.
Epidermis
One of the most fundamental and visible features of skin is the stratified, cornified epidermis (Fig. 7-2). The epidermis is a continually renewing structure that gives rise to derivative structures called appendages (pilosebaceous units, nails, and sweat glands). The epidermis ranges in thickness from 0.4 to 1.5 mm, as compared with the 1.5- to 4.0-mm full-thickness skin. The majority of cells in the epidermis are keratinocytes that are organized into four layers, named for either their position or a structural property of the cells. These cells progressively differentiate from proliferative basal cells, attached to the epidermal basement membrane, to the terminally differentiated, keratinized stratum corneum, the outermost layer and barrier of skin (see Chapter 46). Intercalated among the keratinocytes at various levels are the immigrant resident cells—melanocytes, Langerhans cells, and Merkel cells. Other cells, such as lymphocytes, are transient inhabitants of the epidermis and are extremely sparse in normal skin. There are many regional differences in the epidermis and its appendages. Some of these differences are apparent grossly, such as thickness (e.g., palmoplantar skin vs. truncal skin, Fig. 7-3); other differences are microscopic.
Figure 7-2
Schematic of epidermis. The epidermis is a stratified, cornified epithelium. The deepest layer consists of basal cells (BL) that rest upon the basement membrane of the dermal–epidermal junction (DEJ). These cells differentiate into the cells of the spinous layer (SL), characterized by abundant desmosomal spines. Spinous cells eventually become granular layer cells (GL), producing many of the components of the cornified envelope. Ultimately, the terminally differentiated keratinocytes shed their nuclei and become the stratum corneum (SC), a cross-linked network of protein and glycolipids.
Pathologic changes in the epidermis can occur as a result of a number of different stimuli: repetitive mechanical trauma (as in lichen simplex chronicus), inflammation (as in atopic dermatitis and lichen planus), infection (as in verruca vulgaris), immune system activity and cytokine abnormalities (as in psoriasis, Fig. 7-4), autoantibodies (as in pemphigus vulgaris and bullous pemphigoid), or genetic defects that influence differentiation or structural proteins [as in epidermolysis bullosa (EB) simplex, epidermolytic ichthyosis and other ichthyoses, and Darier disease].
The keratinocyte is an ectodermally derived cell and is the primary cell type in the epidermis, accounting for at least 80% of the total cells. The ultimate fate of these cells is to contribute the components for the epidermal barrier as the stratum corneum. Thus, much of the function of the epidermis can be gleaned from the study of the structure and development of the keratinocyte.
Keratinocyte differentiation (keratinization) is a genetically programed, carefully regulated, complex series of morphologic changes and metabolic events whose endpoint is a terminally differentiated, dead keratinocyte (corneocyte) that contains keratin filaments, matrix protein, and a protein-reinforced plasma membrane with surface-associated lipids (see Chapter 46).
Keratins are a family of intermediate filaments and are the hallmark of all epithelial cells, including keratinocytes.1,2 They serve a predominantly structural role in the cells. Fifty-four different functional keratin genes have been identified in humans—34 epithelial keratins and 17 hair keratins.3 The coexpression of specific keratin pairs is dependent on cell type, tissue type, developmental stage, differentiation stage, and disease condition (Table 7-2). Furthermore, the critical role of these molecules is underscored by the numerous manifestations of disease that arise because of mutations in these genes (see Table 7-2). Thus, knowledge of keratin expression, regulation, and structure provides insight into epidermal differentiation and structure.
Basic | Acidic | Tissue Expression | Disease Association |
---|---|---|---|
1 | 10 | Suprabasal keratinocytes | Epidermolytic ichthyosis; diffuse nonepidermolytic PPK (keratin 1) |
1 | 9 | Suprabasal keratinocytes (palmoplantar skin) | Epidermolytic PPK (epidermolytic hyperkeratosis) |
2 | 10 | Upper spinous and granular layers | Superficial epidermolytic ichthyosis |
3 | 12 | Cornea | Meesmann’s corneal dystrophy |
4 | 13 | Mucosal epithelium | White sponge nevus |
5 | 14 | Basal keratinocytes | Epidermolysis bullosa simplex |
6a | 16 | Outer root sheath, hyperproliferative keratinocytes, palmoplantar keratinocytes | Pachyonychia congenita; focal nonepidermolytic PPK |
6b | 17 | Nail bed, epidermal appendages | Pachyonychia congenita; steatocystoma multiplex |
8 | 18 | Simple epithelium | Cryptogenic cirrhosis |
The basal layer (stratum germinativum) contains mitotically active, columnar-shaped keratinocytes that attach via keratin filaments (K5 and K14) to the basement membrane zone at hemidesmosomes (see Chapter 53), attach to other surrounding cells through desmosomes, and that give rise to cells of the more superficial, differentiated epidermal layers. Membrane-bound vacuoles that contain pigmented melanosomes are transferred from melanocytes by phagocytosis.4 The pigment within melanosomes contributes to the overall skin pigmentation perceived macroscopically.5 The basal layer is the primary location of mitotically active cells of the epidermis. Cell kinetic studies suggest that the basal layer cells exhibit different proliferative potentials (stem cells, transit amplifying cells, and postmitotic cells), and in vivo and in vitro studies suggest that there exist long-lived epidermal stem cells (see Chapter 45).6,7 Because basal cells can be expanded in tissue culture and used to reconstitute sufficient epidermis to cover the entire skin surface of burn patients,8,9 such a starting population is presumed to contain long-lived stem cells with extensive proliferative potential, located within the basal epidermal layer (at the base of epidermal proliferating units) and the hair follicle bulge.10–13
The second type of cell, the transit amplifying cells of the basal layer, arises as a subset of daughter cells produced by the infrequent division of stem cells, either by symmetric or asymmetric cell division.14 These cells provide the bulk of the cell divisions needed for stable self-renewal and are the most common cells in the basal compartment. These cells subsequently give rise to the third class of epidermal basal cells, the postmitotic cells that undergo terminal differentiation. In humans, the normal transit time for a basal cell, from the time it loses contact with the basal layer to the time it enters the stratum corneum, is at least 14 days. Transit through the stratum corneum and subsequent desquamation require another 14 days. These periods of time can be altered in hyperproliferative or growth-arrested states.
The shape, structure, and subcellular properties of spinous cells correlate with their position within the midepidermis. They are named for the spine-like appearance of the cell margins in histologic sections. Suprabasal spinous cells are polyhedral in shape with a rounded nucleus. As these cells differentiate and move upward through the epidermis, they become progressively flatter and develop organelles known as lamellar granules (see Section “Granular Layer”). Spinous cells also contain large bundles of keratin filaments, organized around the nucleus and inserted into desmosomes peripherally.
Spinous cells retain the stable K5/K14 keratins that are produced in the basal layer and only synthesize new messenger RNA (mRNA) for these proteins in hyperproliferative disorders. Instead, new synthesis of the K1/K10 keratin pair occurs in this epidermal layer. These keratins are characteristic of an epidermal pattern of differentiation and thus are referred to as the differentiation-specific or keratinization-specific keratins. However, in hyperproliferative conditions such as psoriasis, actinic keratoses, and wound healing, synthesis of K1 and K10 mRNA and protein is downregulated, and the synthesis and translation of messages for K6 and K16 are favored. Correlated with this change in keratin expression is a disruption of normal differentiation in the subsequent granular and cornified epidermal layers (see Sections “Granular Layer” and “Stratum Corneum”). mRNA for K6 and K16 are present throughout the epidermis normally, but the message is only translated on stimulation of proliferation.
The “spines” of spinous cells are abundant desmosomes, calcium-dependent cell surface modifications that promote adhesion of epidermal cells and resistance to mechanical stress (see Chapters 46 and 53).15 Although desmosomes are related to adherens junctions, the latter associate with actin microfilaments at cell–cell interfaces, via a distinct set of cadherins (e.g., E-cadherin) and intracellular catenin adapter molecules. That the desmosomes are integral mediators of intercellular adhesion is clearly demonstrated in diseases in which these structures are disrupted, by genetic disorders, autoantibodies, or bacterial proteases (Table 7-3).16,17
Protein | Diseases |
---|---|
Desmoglein 1 | Pemphigus foliaceus Striate palmoplantar keratoderma Staphylococcal scalded-skin syndrome Bullous impetigo |
Desmoglein 3 | Pemphigus vulgaris |
Desmoglein 4 | Autosomal recessive hypotrichosis |
Plakoglobin | Palmoplantar keratoderma with wooly hair and arrhythmogenic right ventricular cardiomyopathy (Naxos disease) |
Plakophilin 1 | Ectodermal dysplasia/skin fragility syndrome (skin erosions, dystrophic nails, sparse hair, and painful palmoplantar keratoderma) |
Plakophilin 2 | Arrhythmogenic right ventricular cardiomyopathy |
Desmoplakin | Lethal acantholytic epidermolysis bullosa Striate palmoplantar keratoderma, type I Palmoplantar keratoderma with left ventricular cardiomyopathy and wooly hair Autosomal dominant arrhythmogenic right ventricular cardiomyopathy |
The importance of calcium as a mediator of adhesion is well illustrated in the cases of two conditions that exhibit characteristic epidermal dyscohesion: (1) Darier disease (keratosis follicularis) and (2) Hailey–Hailey disease (benign chronic pemphigus) (see Chapter 51).18 Both of these diseases are caused by mutations in genes that regulate calcium transport, SERCA2 in Darier disease and ATP2C1 in Hailey–Hailey disease.
Lamellar granules are also formed in this layer of epidermal cells (Fig. 7-5). These secretory organelles deliver precursors of stratum corneum lipids into the intercellular space (see Chapter 47). Genetic diseases demonstrate the importance of steroid and lipid metabolism for sloughing of cornified cells—in recessive X-linked ichthyosis, for example, mutation of steroid sulfatase results in a retention hyperkeratosis (see Chapter 49).19
Figure 7-5
Junction of the stratum granulosum (SG) and stratum corneum (SC). Lamellar granules (LG) are in the intercellular space and cytoplasm of the granular cell. Keratohyalin granules (KHG) are also evident. Inset: Lamellar granule, ×28,750. (From Holbrook K: Structure and development of the skin. In: Pathophysiology of Dermatologic Disease, 2nd edition, edited by Soter NA, Baden HP. New York, McGraw-Hill, 1991, p. 7, with permission. Inset used with permission from EC Wolff-Schreiner, MD.)
Named for the basophilic keratohyalin granules that are prominent within cells at this level of the epidermis, the granular layer is the site of generation of a number of the structural components that will form the epidermal barrier, as well as a number of proteins that process these components (see Fig. 7-2).20,21 Keratohyalin granules (see Fig. 7-5) are composed primarily of profilaggrin, keratin filaments, and loricrin. It is in this layer that the cornified cell envelope begins to form, with the conversion of profilaggrin to filaggrin. After aggregation with keratin to form macrofilaments, filaggrin is degraded into molecules such as urocanic acid and pyrrolidone carboxylic acid, which contribute to hydration of the stratum corneum and help filter UV radiation. Loricrin is a cysteine-rich protein that forms the major protein component of the cornified envelope. Upon its release from keratohyalin granules, loricrin binds to desmosomal structures and is subsequently cross-linked to the plasma membrane by tissue transglutaminases (TGMs, primarily TGMs 3 and 1) to form the cornified cell envelope.
Mutations in the TGM1 gene have been shown to be the basis of some cases of lamellar ichthyosis.22,23 Another form of ichthyosis, ichthyosis vulgaris, is caused by mutations in the gene encoding filaggrin.24,25 Loricrin abnormalities result in a form of Vohwinkel syndrome with ichthyosis and pseudoainhum, as well as the disease progressive symmetric keratodermia.26–28 These findings emphasize the importance of proper formation of the cornified envelope in normal epidermal keratinization.
The final stage of granular cell differentiation into a corneocyte involves the cell’s own programed destruction, during which process almost all cellular contents are destroyed, with the exception of the keratin filaments and filaggrin matrix.20
Complete differentiation of granular cells results in stacked layers of anucleate, flattened cornified cells that form the stratum corneum. It is this layer that provides mechanical protection to the skin and a barrier to water loss and permeation of soluble substances from the environment.21,29 The stratum corneum barrier is formed by a two-compartment system of lipid-depleted, protein-enriched corneocytes surrounded by a continuous extracellular lipid matrix. These two compartments provide somewhat segregated but complementary functions that together account for the “barrier activity” of the epidermis. Regulation of permeability, desquamation, antimicrobial peptide activity, toxin exclusion, and selective chemical absorption are all primarily functions of the extracellular lipid matrix. On the other hand, mechanical reinforcement, hydration, cytokine-mediated initiation of inflammation, and protection from UV damage are all provided by the corneocytes.
Melanocytes are neural crest-derived, pigment-synthesizing dendritic cells that reside primarily in the basal layer (see Chapter 72).30 The function of melanocytes has been highlighted by disorders in melanocyte number or function. The classic dermatologic disease, vitiligo, is caused by the autoimmune depletion of melanocytes.31 Causes of other disorders of pigmentation are found in various defects in melanogenesis, including melanin synthesis, melanosome production, and melanosome transport and transfer to keratinocytes (see Chapters 72 and 75). Regulation of melanocyte proliferation and homeostasis is under intensive study as well as a means to understanding melanoma (see Chapter 124).32 Keratinocyte–melanocyte interactions are critical for melanocyte homeostasis and differentiation, influencing proliferation, dentricity, and melanization.
Merkel cells are slow-adapting type I mechanoreceptors located in sites of high-tactile sensitivity (see Chapter 120).33 They are present among basal keratinocytes in hairy skin and in the glabrous skin of the digits, lips, regions of the oral cavity, and the outer root sheath of the hair follicle. Keratin 20 is restricted to Merkel cells in the skin and thus may be the most reliable molecular marker. Ultrastructurally, Merkel cells are easily identified by the membrane-bounded, dense-core granules that collect opposite the Golgi and proximal to an unmyelinated neurite (Fig. 7-6). These granules contain neurotransmitter-like substances and markers of neuroendocrine cells, including Met-enkephalin, vasoactive intestinal peptide, neuron-specific enolase, and synaptophysin. Although increasingly more is being learned about the normal function of Merkel cells, they are of particular clinical note because Merkel cell-derived neoplasms are particularly aggressive and difficult to treat (see Chapter 120).
Langerhans cells are dendritic antigen-processing and antigen-presenting cells in the epidermis (see Chapter 10).34 Although they are not unique to the epidermis, they form 2% to 8% of the total epidermal cell population, mostly found in a suprabasal position. The cytoplasm of the Langerhans cells contains characteristic small rod- or racket-shaped structures called Langerhans cell granules or Birbeck granules (Fig. 7-7). Langerhans cells principally function to sample and present antigens to T cells of the epidermis. Because of these functions, they are implicated in the pathologic mechanisms underlying allergic contact dermatitis, cutaneous leishmaniasis, and human immunodeficiency virus infection. Langerhans cells are reduced in the epidermis of patients with certain conditions, such as psoriasis, sarcoidosis, and contact dermatitis; they are functionally impaired by UV radiation, especially UVB.
Because of their effectiveness in antigen presentation and lymphocyte stimulation, dendritic cells and Langerhans cells have become prospective vehicles for tumor therapy and tumor vaccines. These cells are loaded with tumor-specific antigens, which will then stimulate the host immune response to mount an antigen-specific, and therefore tumor-specific, response.
Dermal–Epidermal Junction
The dermal–epidermal junction (DEJ) is a basement membrane zone that forms the interface between the epidermis and dermis (see Chapter 53).35,36 The major functions of the DEJ are to attach the epidermis and dermis to each other and to provide resistance against external shearing forces. It serves as a support for the epidermis, determines the polarity of growth, directs the organization of the cytoskeleton in basal cells, provides developmental signals, and serves as a semipermeable barrier.
The DEJ can be subdivided into three supramolecular networks: (1) the hemidesmosome-anchoring filament complex, (2) the basement membrane itself, and (3) the anchoring fibrils. The critical role of this region in maintaining skin structural integrity is revealed by the large number of mutations in DEJ components that cause blistering diseases of varying severity, covered in detail in Chapter 62. These bullous diseases are grouped according to the level of the cleavage within the DEJ—the most superficial, EB simplex, involves basal keratinocyte cleavage. Junctional EB occurs within the lamina lucida and lamina densa regions. Dystrophic EB is the deepest level of blistering, within the sublamina densa/anchoring filaments. Chapter 53 provides a detailed discussion of the DEJ networks.
Dermis
The dermis is an integrated system of fibrous, filamentous, diffuse, and cellular connective tissue elements that accommodates nerve and vascular networks, epidermally derived appendages, and contains many resident cell types, including fibroblasts, macrophages, mast cells, and transient circulating cells of the immune system (see Figs. 6-9 and 6-14). The dermis makes up the majority of skin and provides its pliability, elasticity, and tensile strength. It protects the body from mechanical injury, binds water, aids in thermal regulation, and includes receptors of sensory stimuli. The dermis interacts with the epidermis in maintaining the properties of both tissues, collaborates during development in the morphogenesis of the DEJ and epidermal appendages (see Section “Development of Skin Appendages”), and interacts in repairing and remodeling skin after wounding.
The dermis is arranged into two major regions: (1) the upper papillary dermis and (2) the deeper reticular dermis. These two regions are readily identifiable on histologic section, and they differ in their connective tissue organization, cell density, and nerve and vascular patterns. The papillary dermis abuts the epidermis, molds to its contours, and is usually no more than twice its thickness (see Fig. 6-9). The reticular dermis forms the bulk of the dermal tissue. It is composed primarily of large-diameter collagen fibrils, organized into large, interwoven fiber bundles, with branching elastic fibers surrounding the bundles (see Fig. 6-14). In normal individuals, the elastic fibers and collagen bundles increase in size progressively toward the hypodermis. The subpapillary plexus, a horizontal plane of vessels, marks the boundary between the papillary and reticular dermis. The lowest boundary of the reticular dermis is defined by the transition of fibrous connective tissue to adipose connective tissue of the hypodermis.
The connective tissue matrix of the dermis is comprised primarily of collagenous and elastic fibrous tissue.37,38 These are combined with other, nonfibrous connective tissue molecules, including finely filamentous glycoproteins, proteoglycans (PGs), and glycosaminoglycans (GAGs) of the “ground substance.” 39
Collagen forms the bulk of the acellular portion of the dermis, accounting for approximately 75% of the dry weight of skin, and providing both tensile strength and elasticity. (For details regarding the polypeptide structure and distribution of collagens, see Chapter 63.) The periodically banded, interstitial collagens account for the greatest proportion of collagen in adult dermis (type I, 80% to 90%; type III, 8% to 12%; and type V, <5%). Type VI collagen is associated with fibril and in the interfibrillar spaces. Type IV collagen is confined to the basal lamina of the DEJ, vessels, and epidermal appendages. Type VII collagen forms anchoring fibrils at the DEJ.
Elastic connective tissue (see Chapter 63) is a complex molecular mesh, extending from the lamina densa of the DEJ throughout the dermis and into the connective tissue of the hypodermis.38 Elastic fibers return the skin to its normal configuration after being stretched or deformed. They are also present in the walls of cutaneous blood vessels and lymphatics and in the sheaths of hair follicles. Mutations in elastin, the elastic fiber matrix component, cause the disease cutis laxa. Elastic fibers are normally located between bundles of collagen fibers, although in certain pathologic conditions, such as Buschke–Ollendorff syndrome, both elastic and collagen fibers become assembled within the same bundle. The importance of the elastic fiber network is clearly seen in the number of multisystem diseases that arise because of mutations in components of this network. The defect underlying pseudoxanthoma elasticum (PXE) is a mutation in ABCC6, a member of the large adenosine triphosphate-dependent transmembrane transporter family. Thus, this disease that is characterized by loss of skin elasticity and calcified elastic fibers is unlikely a primary defect in elastic tissue, but rather a metabolic disorder with secondary involvement of elastic fibers.40–42 In addition to genetic mutations, solar radiation and aging also contribute to elastic fiber damage.43
The fibrous and cellular matrix elements are embedded within more amorphous matrix components, which also are found in basement membranes.44–46 PGs are large molecules consisting of a core protein that determines which GAGs will be incorporated into the molecule. The PG/GAG complex can bind water up to 1,000 times its own volume and have roles in regulation of water binding and compressibility of the dermis, as well as increasing local concentrations of growth factors through binding (e.g., basic fibroblast growth factor). They also link cells with the fibrillar and filamentous matrix, influencing proliferation, differentiation, tissue repair, and morphogenesis.
The major PGs in the adult dermis are chondroitin sulfates/dermatan sulfate, including biglycan, decorin, and versican; heparan/heparan sulfate PGs, including perlecan and syndecan; and chondroitin-6 sulfate PGs, which are components of the DEJ (see Chapter 63). Glycoproteins interact with other matrix components via integrin receptors. They facilitate cell migration, adhesion, morphogenesis, and differentiation. Fibronectin is synthesized by both epithelial and mesenchymal cells, and it covers collagen bundles and the elastic network. Vitronectin is present on all elastic fibers except for oxytalan. Tenascin is found around the smooth muscle of blood vessels, arrector pili muscles, and appendages such as sweat glands.
Fibroblasts, macrophages, and mast cells are the regular residents of the dermis, mostly found around the papillary region and surrounding vessels of the subpapillary plexus (see Fig. 6-20), as well as in the reticular dermis between collagen fiber bundles. The fibroblast is a mesenchymally derived cell that migrates through the tissue and is responsible for the synthesis and degradation of fibrous and nonfibrous connective tissue matrix proteins and a number of soluble factors. Fibroblasts provide a structural extracellular matrix framework as well as promote interaction between epidermis and dermis by synthesis of soluble mediators. Studies of human fibroblasts indicate that even within a single tissue, phenotypically distinct populations exist, some of which relate to regional anatomical differences.47,48 These cells are also instrumental in wound healing and scarring, increasing their proliferative and synthetic activity during these processes.
The monocytes, macrophages, and dermal dendrocytes constitute the mononuclear phagocytic system of cells in the skin. Macrophages are derived from precursors in the bone marrow, differentiate into circulating monocytes, and then migrate into the dermis to differentiate. These cells are phagocytic; process and present antigen to immunocompetent lymphoid cells; are microbicidal, tumoricidal, secretory, and hematopoietic (see Chapter 10); and are involved in coagulation, atherogenesis, wound healing, and tissue remodeling.
Mast cells (see Chapter 149) are specialized secretory cells that, in skin, are present in greatest density in the papillary dermis, near the DEJ, in sheaths of epidermal appendages, and around blood vessels and nerves of the subpapillary plexus. The surface of dermal mast cells is coated with fibronectin, which probably assists in securing cells within the connective tissue matrix. Mast cells are secretory cells that are responsible for immediate-type hypersensitivity reaction in skin and are involved in the production of subacute and chronic inflammatory disease. They synthesize secretory granules composed of histamine, heparin, tryptase, chymase, carboxypeptidase, neutrophil chemotactic factor, and eosinophilic chemotactic factor of anaphylaxis, which are mediators in these processes. Mast cells can become hyperplastic and hyperproliferative in mastocytosis (see Chapter 149).
The dermal dendrocyte is a dendritic, highly phagocytic fixed connective tissue cell in the dermis of normal skin. Similar to many other bone marrow-derived cells, dermal dendrocytes express factor XIIIa and CD45, and they lack typical markers of fibroblasts. These cells are particularly abundant in the papillary dermis and upper reticular dermis, frequently in the proximity of vessels of the subpapillary plexus. Dermal dendrocytes function in the afferent limb of an immune response as antigen presenting cells (see Chapter 10). They are also likely the cells of origin of a number of benign fibrotic proliferative conditions in the skin, such as dermatofibromas and fibroxanthomas (see Chapter 66).
Cutaneous Vasculature
The blood vessels of skin provide nutrition for the tissue and are involved in temperature and blood pressure regulation, wound repair, and numerous immunologic events.49 The microcirculatory beds in skin progress from arterioles to precapillary sphincters. Extending from the sphincters are arterial and venous capillaries, which become postcapillary venules, and finally, collecting venules. When compared with vasculature of other organs, the vessels of skin are adapted to shearing forces, as they have thick walls supported by connective tissue and smooth muscle cells. Special cells, known as veil cells
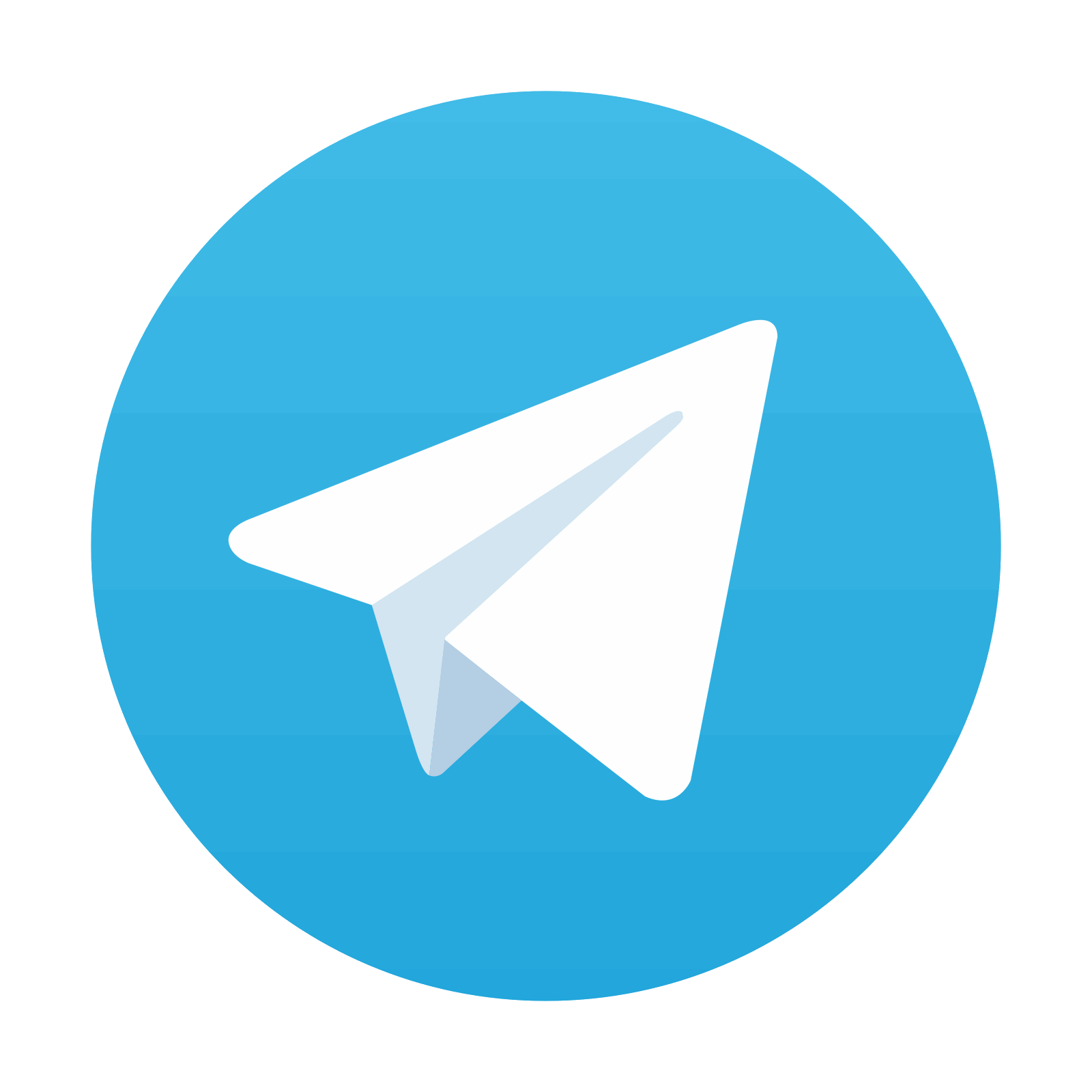
Stay updated, free articles. Join our Telegram channel

Full access? Get Clinical Tree
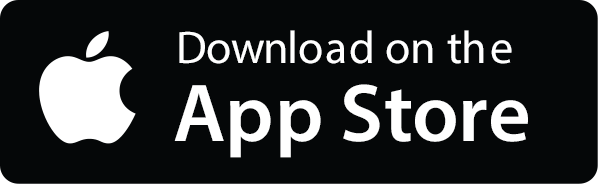
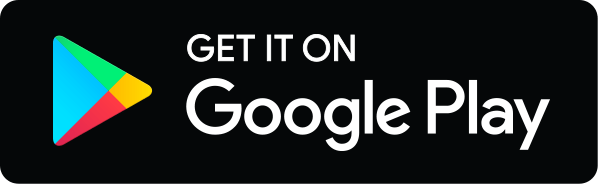
