3.2.4.2 Skin Aging: Changes in the Epidermis and Stratum Corneum
3.2.4.4 The Dermatoglyphic Pattern of the Skin
3.2.4.5 Aging and Mechanical Properties of Skin
3.2.4.7 Photo-Aging Mechanisms
3.2.4.8 Photo-Aging and Appearance
3.2.4.9 Compromised Elderly Skin Treatments
a. Sunscreens—An Ounce of Prevention
b. All-Trans Retinoic Acid (Tretinoin): The Gold Standard
c. Cosmeceutical Treatments for Aging Skin
d. Vitamin A and “Cosmeceutical” Derivatives
f. Alpha and Beta Hydroxy Acids
We begin with a brief review of skin structure to facilitate our discussion of skin aging. This subject is discussed in more detail in other chapters to this book. Discussion of the structure of skin will naturally refer to its various layers (23, 24) or strata. The skin’s multiple layers work together to perform its multiple functions. Figure 1 is a hematoxyln and eosin (H&E) stained image of a cross-section of human skin.
Figure 1 (H&E stained cross section of human skin labeled to show the various layers. The “basket weave” appearance of the stratum corneum is an artifact of processing. http://en.wikipedia.org/wiki/Epidermis_(skin) public domain image)
The epidermis and dermis are fundamentally different types of tissue. The epidermis overlays the papillary dermis and is composed of cells. The main type of cell of the epidermis is the keratinocyte. Keratinocytes are found from the basal layer to the granular layer where they are transformed into the corneocytes of the stratum corneum (SC). Keratinocytes make many proteins including keratins, the main structural proteins of the SC (25). Two other important cells in the epidermis are melanocytes and Langerhans cells.
The dermis has two layers, papillary and reticular. The hypodermis or subcutaneous tissue is sometimes considered part of the dermis but will not be considered in this review. The dermis is a fibrous tissue composed primarily of collagen, elastin, and proteoglycans, which form the dermal matrix. The papillary dermis is named for the dermal papilla protruding into the epidermis (Figure 1). The fibroblasts, which make the proteins and proteoglycans of the dermis, are found in the dermal matrix (24).
The main structural protein of the dermis is collagen. Collagen has a unique amino acid sequence. Every third amino acid is glycine, which does not have a side chain. This allows collagen to form a compact triple-helix with three collagen chains winding around each other as if in a braid. Each triple helix is about 300 nm long and 1.5 nm in diameter (26). Collagen is synthesized in fibroblasts as pro-collagen and exported from the cell into the dermal matrix where it is processed by proteolytic enzymes, which remove sections from both ends of pro-collagen to form 300-nm filaments. These filaments then aggregate in the extracellular space to form collagen fibers.
The matrix in the papillary dermis is loosely packed with fibers compared to the tightly packed reticular dermis (24). The papillary dermis contains type III collagen, which is found at relatively low levels in the reticular dermis where type I collagen predominates (27–29). Oxytalan fibers are thin (10–12nm) elastic fibers found perpendicular to the dermal-epidermal junction in the papillary dermis (30, 31). Elaunin fibers are also found in the papillary dermis parallel to the plane of the skin (30).
Elastin provides elasticity to skin (32). In elastin, four protein chains are bound together through the desmosine cross-link. Elastin has an amino acid residue called allysine, made from lysine by the enzyme lysyl oxidase. The desmosine cross-link is formed between three allylisine chains and one lysine chain. Amorphous elastin fibers surrounded by fibrillin microfibrils are found predominately in the reticular dermis (33, 34) (Figure 2).
Figure 2: Electron micrograph of the reticular dermis showing collagen, elastin, a fibroblast, and a mast cell. (© Raymond Boissy, used with permission)
Glycosaminoglycans or GAGS bind water in the dermal matrix (35) keeping the dermis hydrated. Hyaluronic acid (HA), a major component of GAGS, is a very high molecular weight. GAGs such as chondroitin sulfate and keratan sulfate bind to core proteins to form proteoglycans. Proteins called link proteins bind proteoglycans to HA in a noncovalent proteoglycan complex that is very hygroscopic.
Figure 2 is an electron micrograph of the reticular dermis showing collagen and elastin fibers as well as a fibroblast and a mast cell. Mast cells contain histamine granules and release histamine in response to heat or irritants. The reticular dermis is much more densely packed with fibers than the papillary dermis.
The dermal matrix is significantly affected by both intrinsic and extrinsic aging as will be discussed below.
Skin barrier function resides primarily in the epidermis and barrier may be considered “la raison d’être” of the epidermis (36). Layers of the epidermis are shown schematically in Figure 3.
Figure 3: Diagram showing layers of the epidermis (illustration by Robin M. Wickett)
The epidermal barrier defends the body from water loss to the environment, absorption of noxious chemicals from the environment, and microbial infection. These defensive functions depend critically on the top layer, the stratum corneum (SC), and are thought to be integrated with SC formation and homeostasis (37, 38).
For 30 years the most common model used to organize thinking about the SC barrier has been the “bricks and mortar” model proposed by Elias (39). This model is based on the fact that corneocytes are surrounded by the extracellular lipids of the SC. Thus the corneocytes are modeled as bricks and the lipids as mortar. The model has proven useful for representing the phenomena associated with describing ingredient skin penetration. Moderately hydrophobic molecules are thought to penetrate the SC by diffusing through the lipid mortar, winding their way around the corneocytes bricks (40). Harding has described an updated version of the bricks-and-mortar model that includes the presence of the desmosomes that hold SC cells together prior to desquamation and the role of natural moisturizing factor in SC function (41). This model is illustrated in Figure 4.
Figure 4: Schematic of the “bricks and mortar” model for human stratum corneum illustrating the corneocyte “bricks,” the intercellular lipid “mortar,” and the desmosomes connecting the corneocytes. (M.O. Visscher, used with permission)
The “bricks and mortar” of the SC barrier are formed in the stratum granulosum (SG) (Figure 4) where keratinocytes are transformed to form the corneocytes “bricks” and lipids are released into the intercellular space to form the “mortar.” The SG is named for the two types of granules that appear in the cells, keratohyalin granules (24) that are composed of protein and lamellar bodies that primarily contain lipids (42, 43).
A complex process occurs in the SG: the nucleus is digested, the cytoplasm disappears, and lipids are released into the intercellular space to form the mortar (39, 44, 45). The keratin intermediate filaments aggregate to form microfibrils (46, 47) inside the corneocytes (48, 49). aided by filaggrin from the keratohyalin granules.
Filaggrin is an acronym for filament-aggregating protein (50–53). Filaggrin contains a high level of positively charged amino acids, allowing it to interact with the negatively charged epidermal keratins (54). Filaggrin is then modified to come off the microfibrils, through conversion of the positively charged amino acid arginine to uncharged citruline by the enzyme peptidyl arginine deminase (PAD) (55, 56). After release from the microfibrils filaggrin is digested by proteolytic enzymes to produce the amino acid components of the natural moisturizing factor (NMF) of the stratum corneum (57–62).
NMF consists of lactate, amino acids from filaggrin breakdown, and pyrollidone carboxylic acid (PCA) formed from the amino acid glutamine (63–65). These natural moisturizers are important to maintain proper hydration of the SC allowing it to be flexible and to desquamate properly (57, 66–69). Digestion of filaggrin to NMF is thought to happen in the mid-stratum corneum (70).
In the stratum granulosum (SG) the keratinocyte cell membrane is replaced by the corneocyte cell envelope, a very tough structure made of cross-linked protein with lipids covalently attached to its surface (71–75). The resulting corneocytes or squames (the bricks) are flat cells that tend to be in the shape of either a hexagon or pentagon. Corneocyte size and distribution have been investigated by several authors. A single corneocyte is about 25 μm on a side, with a surface area ranging from about 550 to 1300 μm2 depending on age and body site (76–79), and a thickness of about 0.5–1.0 μm (80, 81). Rougier et al. (79) reported corneocyte surface area on most body sites investigated to range from 900 to 1100 μm2 but to be as low as 500 μm2 on the forehead. A negative correlation was found between corneocyte size and the penetration of benzoic acid through skin in vivo. The stratum corneum is 12–16 cell layers thick on most body sites, but it can vary from as little as nine cell layers on the forehead or eyelids to as much as 25 on the dorsum of the hand and 50 or more on the palms or the soles of the feet (82, 83).
The lipids that are released into the intercellular space as the SC forms are glucosyl ceramides, cholesterol, cholesterol esters, and long-chain fatty acids. In the intercellular space the glucosyl ceramides are converted to ceramides (84, 85). SC ceramides are polar lipids, but much less hydrophilic than the phospholipids of the original cell membrane. Figure 5 shows the structure of some of the main SC ceramides with both the original and new nomenclature that is used for them. Phospholipids from the keratinocytes of the viable layers are broken down by phospholipases (86–88) in the lower stratum corneum. After this extracellular processing, SC lipids spontaneously organize into multiple layers between the SC cells (89). This layered structure is critical to the barrier function of the skin.
Figure 5: Key ceramides of the SC barrier.
3.2.4.2 SKIN AGING: CHANGES IN THE EPIDERMIS
AND STRATUM CORNEUM
A general trend for corneocytes to increase in surface area with age has been reported by several groups (77, 79, 90-93). The reported increase in surface area between young adults and adults over 55 ranges from 8 to 14% depending on body site and method of measurement. Figure 6 shows corneocyte size data from two different age groups reported by Grove et al. (91, 93).
Note the extreme variability in the data from the older age group compared to the narrower distribution in the younger group. The average size of corneocytes is increased in the older age group but there is considerable overlap between the two data sets.
Figure 6: Projected corneocyte surface area for two age ranges for men (●) and women (○). Reprinted from Grove et al. (93) with permission of the Society of Cosmetic Chemists.
While the “bricks” of the SC may increase in size with age, the “mortar” content is apparently decreasing. Imokawa et al. reported a gradual decrease in total SC ceramides with age (94). Rogers et al. reported progressive reductions in fatty acids, ceramides and cholesterol with age in subjects ranging in age from 21–60 years on the hands, legs, and face (95). The reduction in ceramide I (EOS) linolate was especially noteworthy.
SC barrier function is affected by both intrinsic and extrinsic aging (96), but it is relatively robust to both and changes are not always in the direction that one might expect. The most common and best-validated method for determining SC barrier function in vivo is by measurement of the passive diffusion of water through the skin by measuring the rate water evaporates from the surface of dry nonsweating skin. This parameter is known as trans-epidermal water loss (TEWL) (97–99).
Several groups have studied the effects of age on SC barrier function by measuring TEWL. Results have varied. Roskos and Guy (100) reported no correlation between baseline TEWL on the ventral forearm and age for subjects ranging in age from 20 to 90 years, and Marks et al. (101) did not see a significant correlation to age on the forearm with a similar age range, but a trend to lower TEWL was observed for subjects above 55. Rougier et al. found no significant change in TEWL on the upper outer arm in subjects ranging in age from 20 to 80 years (79). Kligman (102) reported that TEWL on both the forearm and lower legs averaged slightly lower for a group of individuals aged 66–81 compared to a 19–26 year old cohort. Leveque et al. (77) reported TEWL from the arm to be approximately constant from 25 to 65 years of age but to decline slightly between ages 65 and 75.
Wilhelm et al. (103) compared TEWL data from a young group (26.7±2.8 years) and an older group (70.5±13.8 years) on several body sites. TEWL was significantly lower from the older age group on all sites except the palm. Saijo et al. (104) measured TEWL from the dorsum of the hand and the dorsum of foot on children from one to five years of age, young adults from 21 to 34 years of age, and older adults ranging from 63 to 78 years of age. Aged individuals had the lowest TEWL values on both body sites. There was no difference in TEWL from the dorsum of the hand between children and young adults. Ghadially et al. (105) reported average TEWL of 4.43±0.16 g/(m2-hr) for a group of six individuals over 80 compared to 6.41±0.93 g/(m2-hr) for a group of 21 subjects under 30.
Schwindt et al. (106) found significantly lower TEWL on the backs of subjects averaging 69.8 years of age compared to subjects averaging 27.7 years old. In a recent study Luebberding et al. reported TEWL data from six different body sites on 150 women divided equally into five age groups, 18–29, 30–39, 40–49, 50–59, and 60–80 (107). No correlation was found between TEWL and age on the neck, forearm, or back of the hand. TEWL was negatively correlated to age on the forehead and cheek. Interestingly, TEWL on the décolleté area was positively correlated to age, ranging from 5.62 gm/m2-hr on the youngest group to 7.86 gm/m2-hr on the oldest, with a high level of statistical significance. In our review of the literature these data from the décolleté are the only ones to show a statistically significant increase in TEWL with age.
The SC barrier to penetration of compounds into the skin has also been evaluated as a function of age. Roskos et al. compared the penetration of six drugs of differing polarity into the skin of subjects 22–40 years of age and over 65 years old in vivo (108). Permeation rates for the two most hydrophobic drugs, testosterone and estradiol, were not significantly different between the two age groups but hydrocortisone, benzoic acid, acetylsalicylic acid, and caffeine all penetrated the skin of the older group at a significantly lower rate than into the younger. Rougier et al. also reported that benzoic acid penetrated older skin significantly more slowly than younger in vivo (79).
Analysis of all these results leads to the somewhat surprising conclusion that basal SC barrier function is relatively unaffected by age from childhood through late middle age and then may actually begin to increase slightly sometime after age 60. It is notable that this apparent improvement in baseline barrier function occurs in spite of the reduction in SC barrier lipids discussed above. Rogers et al. point out that while lipids decrease, the ratio of ceramides to other SC structural lipids remain approximately constant (95). It is also possible that skin permeation of some compounds through SC is slower because of the larger corneocytes. Rougier at al. found a negative correlation between corneocyte size and the skin permeation rate of benzoic acid in vivo (79).
Most studies indicate that the reactivity of skin to irritant may actually decrease with age, or at least is not more marked with age as one might expect. Bettley and Donoghue reported fewer reactions to patch testing with soap in subjects 50 and older compared to subjects 10–49 years of age (109). Grove et al. reported lower skin reactivity to a variety of irritants including dimethyl sulfoxide, ethyl nicotinate, and lactic acid (93, 110) in older subjects. Maibach and coworkers (106, 111) and Robinson (112) observed lower reactivity of older skin to sodium lauryl sulfate (SLS). Robinson also reported that reaction to another strong irritant, octanoic acid, was significantly lower in subjects more than 55 years old while reactions to the weaker irritants acetic acid and decanol were only directionally reduced with age (112). Bowman et al. performed a ten-day cumulative irritation test comparing 26 subjects age 18–45 with 26 subjects age 65–80 using 11 products of widely varying irritancy (113). They found no statistically significant differences in response between the age groups, but the response was directionally less in the older age group for all the compounds that produced significant irritation.
While the static barrier function of the epidermis does not seem to diminish with age, recovery of barrier function after disruption has been found to be significantly slower in older skin. Ghadially et al. disrupted SC by either tape stripping or acetone treatment to the point that TEWL was increased to the range of 20–30 g/m2-hr (105). Recovery of the barrier was quantified by measuring TEWL over the next several days. After acetone treatment young skin (under age 30) recovered its barrier function by 50% at 24 hours, while older skin (over 80) had only recovered 15% at 24 hours. Younger subjects took about four days to recover to 90% of their original SC barrier to TEWL while older skin required seven days to reach this level of recovery regardless of the type of insult (tape or acetone). The slower recovery of barrier function may be related to slower rates of epidermal renewal in older skin.
It is well known that corneocytes are continually shed into the environment and are being continually replaced by cells generated in the SG (see above). The SC “turnover time” is most frequently measured by staining the SC with the fluorescent dye, dansyl chloride, and monitoring the rate of dye disappearance with a UV light. When all of the fluorescence has disappeared, the SC has turned over completely (114). Grove and coworkers reported that SC turnover time is increased in elderly skin (76, 93). The turnover time for SC on the volar forearm increased from 19.8 ± 1.4 days in cohort under 35 years old to 28.1 ± 2.7 days in an over 60 age group. Similar changes were seen on the inner arm. These results confirmed the positive correlation between age and the time to turn over the SC reported by Roberts and Marks (115).
Grove found the number of SC cell layers was approximately constant between age groups, indicating that the increased SC turnover time results from decreased epidermal cell proliferation rates with age. Marks reported a small but statistically significant decline with age in the number of epidermal cells in the DNA synthesis phase (116). Engelke et al. investigated differences between young normal skin, young dry skin, aged normal skin, and aged dry skin (117) using an antibody that stains the nuclei of proliferating cells (Ki-S3) (118). Antibody staining indicated a significant reduction in epidermal proliferation rate in aged “normal skin” compared to young normal skin. A slower rate of epidermal cell proliferation is consistent with the increased time to repair a disrupted SC barrier as reported by Ghadially (105). Grove also reported that small chemically induced blisters healed more slowly in subjects between 65 and 70 years of age than in subjects 18–25 years of age (119).
The greater tendency of older subjects to develop dry skin is well known (120–122). Elderly dry skin seems to be especially prone to itching (120, 123–126), which can sometimes be severe. Dry skin in the elderly tends to develop some distinctive patterns, especially on the legs. Figure 7 below shows dry skin that developed on the legs of three elderly subjects who lived in the desert Southwest. These pictures were taken after one week of washing twice daily with a standard soap and no use of moisturizers. Notice the distinct pattern of lines on some of the subjects.
Figure 7: Dry skin on the lower legs of elderly subjects (70–90 y.o.) after washing twice daily with a standard soap and avoidance of moisturizer use.
This pattern of lines frequently develops in elderly subjects but occurs rarely if at all in younger subjects (R.R. Wickett, personal observations). Treatment with an effective moisturizer reduces or eliminates the pattern in some subjects but not in others. In Figure 8 below we see that moisturizer treatment of the right legs has nearly eliminated the pattern in the subject on the left but had little effect on the subject on the right.
Figure 8: Dry skin resulting from soap use on the legs two elderly subjects. After one week of washing with soap and no moisturizer use the right leg of each subject was treated with an effective moisturizing lotion for two weeks while soap treatment continued on both legs.
It is not entirely clear why the elderly are so much more prone to developing dry skin. One possibility is reduction of levels of NMF (see above) in elderly skin. It has been shown that NMF levels are lower in dry skin (62). Data on the effect of age on NMF levels do not present a consistent picture. One of the key moisturizing components of NMF is the very hydrophilic molecule pyrollidone carboxylic acid (PCA) (56, 57) (see above). Harding et al. reported lower levels of PCA in elderly subjects and the difference was more marked deeper in the stratum corneum (70). Horii et al. reported a general decrease in free amino acids with increasing levels of dry skin in elderly subjects (127).
On the other hand, Jacobson et al. found that some SC amino acids decreased with age while others increased, but no difference was seen in total NMF free amino acids (FAA) normalized to protein content between young and old subjects (128). Takahashi and Tezuka (129) reported that NMF FAA actually increased in older subjects. However, both of these groups sampled the stratum corneum by scraping skin flakes from the surface rather than tape stripping to sample from lower levels of the SC as Harding et al. did. Further, as Tagami (96) has pointed out, Takahashi and Tezuka reported their results as FAA/corneocyte. Thus the higher levels of NMF FAA they report could be due to the presence of larger corneocytes in older skin (77, 78, 93) as discussed above.
Lactate is a component of the NMF that may be derived either from sweat (130, 131) or through anaerobic metabolism in the upper epidermis (132). Nakagawa et al. investigated the correlation between NMF components and SC stiffness, pH and hydration in summer and winter with healthy subjects (67). The only components that correlated significantly to SC stiffness and hydration were lactate and potassium. One might expect that the slower metabolism of aging skin reflected by the lower SC turnover rate might lead to lower levels of lactate in the SC. However, Prahl and coworkers reported that keratinocytes from aging skin produce higher levels of lactate ex vivo (133). It is well established that sweating rates decrease with age (134), which might lead to lower levels of lactate and thus stiffer, less well-hydrated SC.
Another factor in skin aging may be the reduction of sebum production that occurs with age (135). Fluhr et al. have presented data from studies of asebic mice, indicating that glycerol derived from the hydrolysis of sebum contributes to skin hydration (136). Sebum production declines dramatically in women at menopause but does not really decline much in men before age 80 (135), so this seems unlikely to be the whole story either. It seems likely that the tendency for elderly subjects to develop dry skin has multiple contributing factors including lower PCA levels, the slower rate of SC renewal, lower levels of lactic acid due to reduced sweating, possibly reduced glycerol due to reduced sebum production, and other factors that have yet to be discovered.
There are been relatively few studies specifically comparing the effects of intrinsic and extrinsic aging on the SC. Tagami’s group studied the dorsum of the hands of Japanese golfers who only wore a golfing glove on one hand (96, 137). Roughness of the skin surface was measured from silicon replicas. Interestingly, there was a significant negative correlation between the difference in roughness between exposed and covered hands and a golfer’s handicap. Better golfers (lower handicap) had larger increases in roughness on their exposed hand. Hydration as measured by higher-frequency conductance was lower for the exposed site indicating a drier skin surface, but there was no difference in barrier function as measured by TEWL.
Both intrinsic aging and photo-aging affect the dermis far more dramatically than the epidermis. The papillary dermis is strongly affected by both intrinsic aging and chronic sun exposure. Figure 9 shows the effect of aging on abdominal skin of 18- and 76-year-old females. At the time these micrographs were published (1955) the abdominal skin of women received very little sun exposure, so the changes represent the effect of intrinsic aging.
Figure 9: Abdominal skin from an 18-year-old female (left) and a 76-year-old female (right). Note the flattening of the dermal-epidermal junction as the papillary dermis diminishes with age.- (modified from Andrew [138] with permission of the Society of Cosmetic Chemists).
This flattening of the papillary dermis makes the skin less resistant to shearing stress, and the skin of elderly people is more easily ulcerated than that of younger people.
The thickness of the skin varies with age and changes depend on body site. In general, skin thickness increases for the first two decades of life. After this initial period, skin thickness may be fairly constant until about age 60. De Rigal et al. reported ultrasound results showing that skin thickness on both the ventral and dorsal forearm decreased significantly with age, but only after age 60 (139). Gniadecka and Jemec, however, reported a gradual decline in skin thickness on the forearms and ankles starting at about age 20 (140). Skin on the forehead and buttocks was found to increase in thickness with age. Takema et al. also found skin thickness to decrease with age on the forearms and increase on the forehead, corners of the eyes, and the cheeks (141). They interpreted this increased thickness as the result of sun exposure on the face.
3.2.4.4 THE DERMATOGLYPHIC PATTERN OF THE SKIN
Young skin shows a complex pattern of shallow lines on the surface. This dermatoglyphic pattern is altered on aging and a pattern with fewer but deeper lines that become more oriented emerges (93). Natural lines of tension in the skin called Langer’s lines may influence the directional orientation. Hassan Zahouani and coworkers have studied these line patterns in detail (142–144). Figure 10 shows typical patterns from subject in various age ranges, pseudo-colored to indicate topography.
Figure 10: Changes with age in the dermatoglyphic pattern of human skin. Figure courtesy of Hassan Zahouani, used with permission.
A highly aligned dermatogyphic pattern can also be seen on the legs of some of the subjects in Figure 8 above.
In addition to the flattened dermal-epidermal junction (Figure 9) and changes in the dermatoglyphic pattern, intrinsically aged skin has diminished collagen synthesis leading to reduced levels of collagen in the dermis (145–147). El-Domyati et al. reported that there is gradual loss of the transverse elaunin fiber network and shortening and eventual reduction of the oxytalin fibers, which were nearly gone by the ninth decade of life in sun-protected skin. Underlying elastic fibers in the mid to deep reticular dermis of sun-protected skin remained relatively unchanged in amount with age (146). Focal loss of elastin and clumping of elastin fiber bundles has been observed in sun-protected elderly skin in some individuals (148).
3.2.4.5 AGING AND MECHANICAL PROPERTIES OF SKIN
Skin is a viscoelastic material. Measurement of mechanical properties of skin in vivo is complicated by its complex, layered nature (Part 1). It is difficult to deform the SC without producing significant deformations in the underlying layers, and of course it is impossible to deform the dermis without deforming the SC. The general principle is that smaller deformations (minimum strain) will help to localize the response to the SC, and larger deformation will reflect more on the properties of the underlying layers (149).
The most commonly used instrument for measuring skin elasticity is the Cutometer® (150–152). The Cutometer uses negative pressure to pull the skin vertically into the opening of the probe (151, 152). Negative pressures can be controlled between 20 and 500 mbar. The height the skin raises into the probe is determined by an optical system. Probes with diameters of 2, 4, 6, and 8 mm are available. In the most widely used mode, deformation is measured while vacuum is applied quickly, held constant for a defined period of time, and then released (Figure 11).
Figure 11: Schematic of Response of Skin to Cutometer
Table 1 defines parameters that can be obtained from the Cutometer curve.
TABLE 1 Cutometer Parameters from Figure 11
UeElastic deformation of the skin due to the application of stress (vacuum)
UvViscoelastic creep occurring after the elastic deformation
UfTotal extensibility of the skin during stress application
UrElastic recovery due to stress removal
UaTotal recovery at the end of the stress-off period
RAmount of deformation not recovered by the end of the stress-off period
Ua/UfOverall elasticity of the skin, including creep and creep recovery
Ur/UePure elasticity ignoring viscoelastic creep
Uv/UeRatio of viscoelastic to elastic extension, called the viscoelastic ratio
Ur/UfRatio of elastic recovery to total deformation
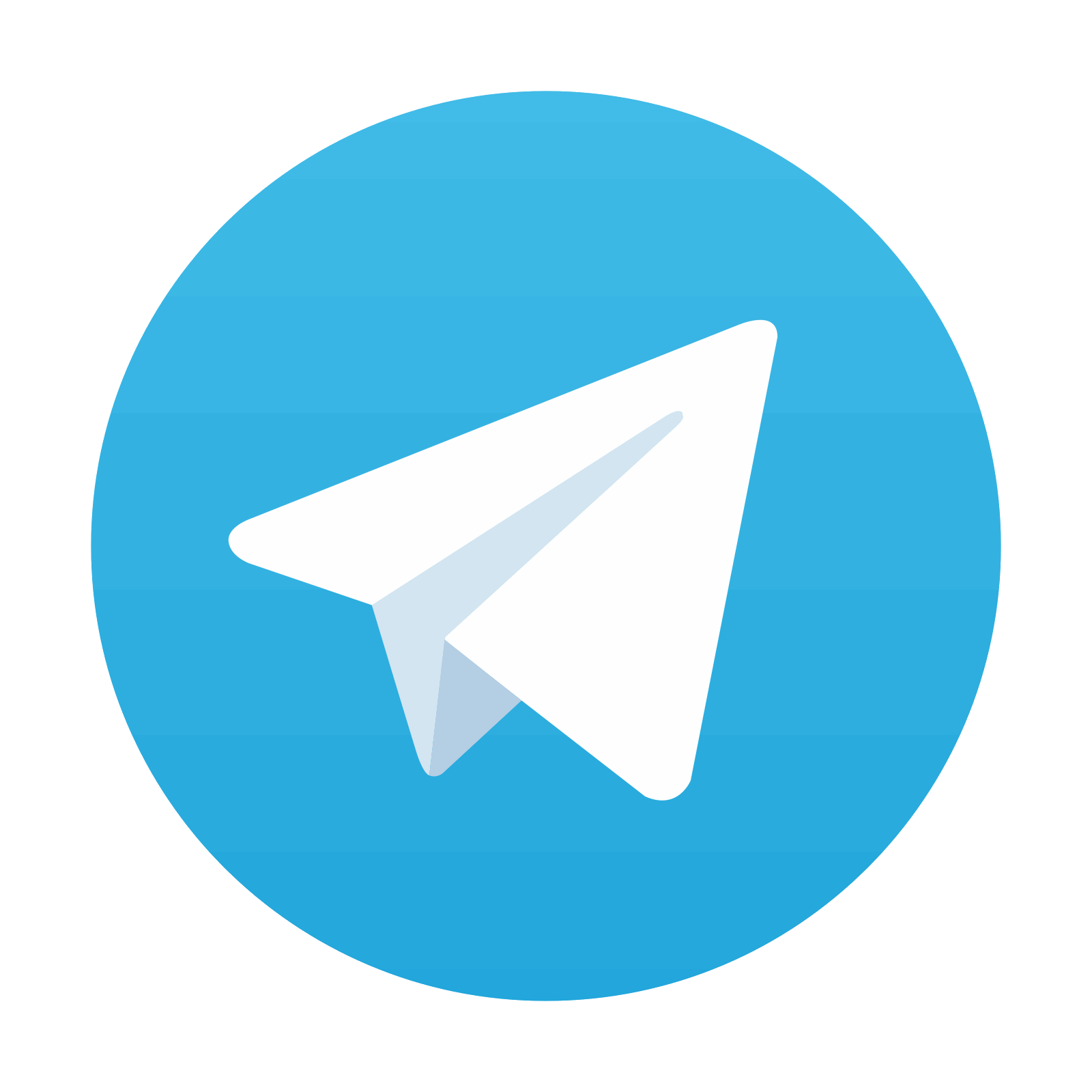
Stay updated, free articles. Join our Telegram channel

Full access? Get Clinical Tree
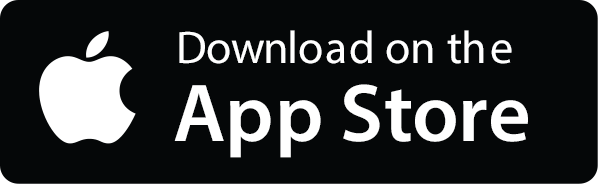
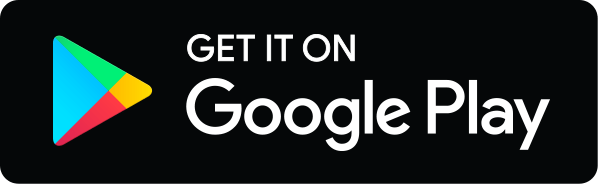